Why atoms are the Universe’s greatest miracle
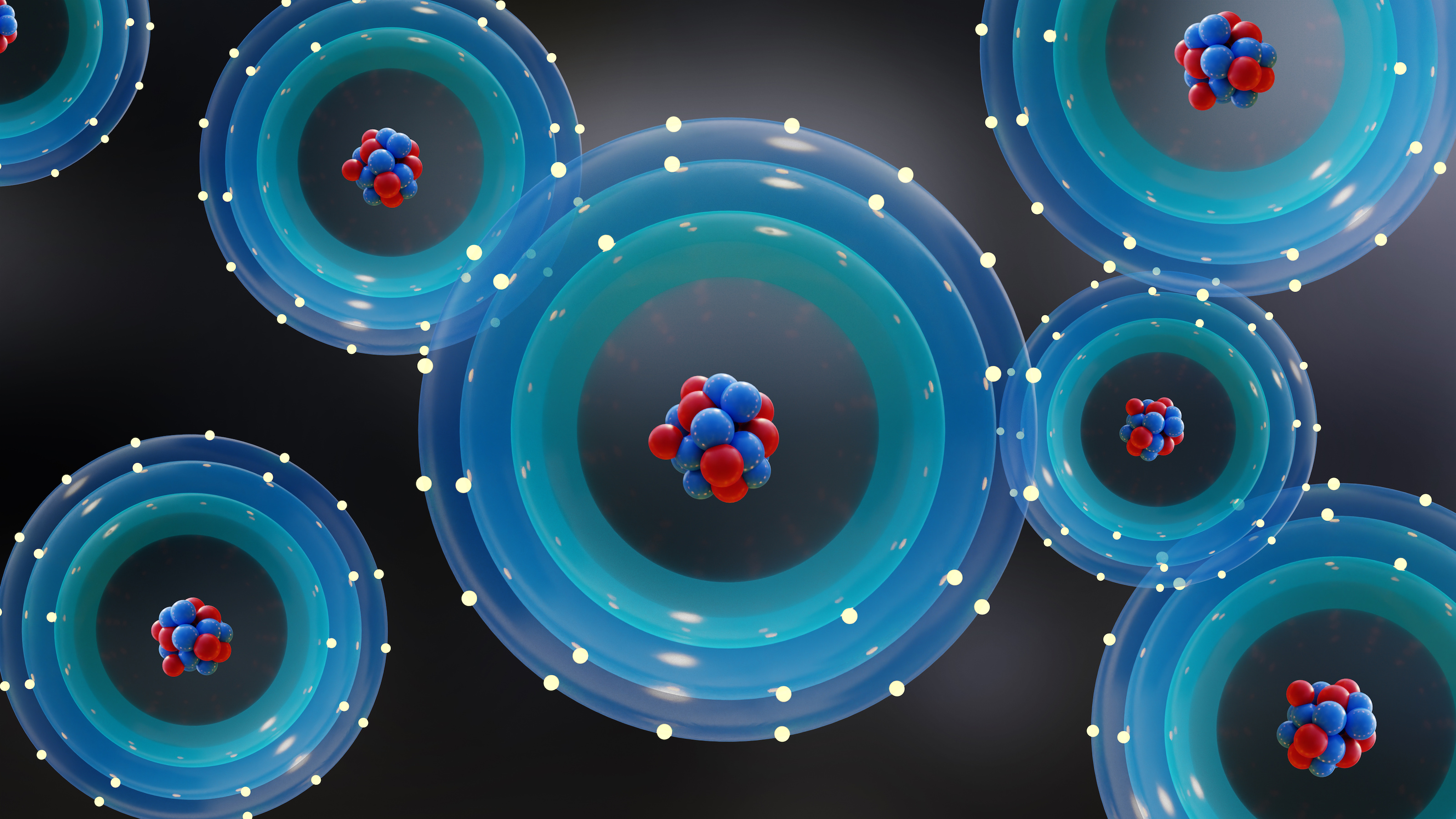
- The humble atom is one of the simplest structures in all the Universe, with a tiny, massive nucleus of protons and neutrons orbited by much lighter electrons.
- And yet, perhaps the most miraculous property of our Universe is that it allows the existence of these atoms, which in turn make up some pretty amazing things, including us.
- Are atoms truly the greatest miracle in all of existence? By the end of this article, you just might be convinced.
One of the most remarkable facts about our existence was first postulated over 2000 years ago: that at some level, every part of our material reality could be reduced to a series of tiny components that still retained their important, individual characteristics that allowed them to assemble to make up all we see, know, encounter, and experience. What began as a simple thought, attributed to Democritus of Abdera, would eventually grow into the atomistic view of the Universe.
Although the literal Greek word “ἄτομος” — meaning “uncuttable” — doesn’t quite apply to atoms, being that they’re made of protons, neutrons, and electrons, any attempt to “divide” the atom further causes it to lose its essence: the fact that it’s a certain, specific element on the periodic table. That’s the essential property that allows it to build up all of the complex structures that exist within our observed reality: the number of protons contained within its atomic nucleus.
An atom is such a small thing that if you were to count up the total number of atoms contained within a single human body, you’d have to count up to somewhere around 1028: more than a million times as great as the number of stars within the entire visible Universe. And yet, just the very fact that we, ourselves, are made of atoms is perhaps the greatest miracle in the entire Universe.

It’s a simple fact that the humble atom is what’s at the core of all the matter we know of within the Universe, from plain old hydrogen gas to humans, planets, stars, and more. Everything that’s made up of normal matter within our Universe — whether solid, liquid, or gas — is made of atoms. Even plasmas, found in very high-energy conditions or in the sparse depths of intergalactic space, are simply atoms that have been stripped of one or more electrons. Atoms themselves are very simple entities, but even with such simple properties, they can assemble to make complex combinations that truly boggle the imagination.
The behavior of atoms is truly remarkable. Consider the following.
- They’re made up of a small, massive, positively charged nucleus, and orbited by a large, low-mass, diffuse cloud of negatively charged electrons.
- When you bring them close to one another, atoms polarize one another and attract, leading to them either sharing electrons together (covalently) or to one atom siphoning one-or-more electrons (ionically) off of the other.
- When multiple atoms bind together, they can create molecules (covalently) or salts (ionically), which can be as simple as having only two atoms bound together or as complex as having several million atoms bound together.
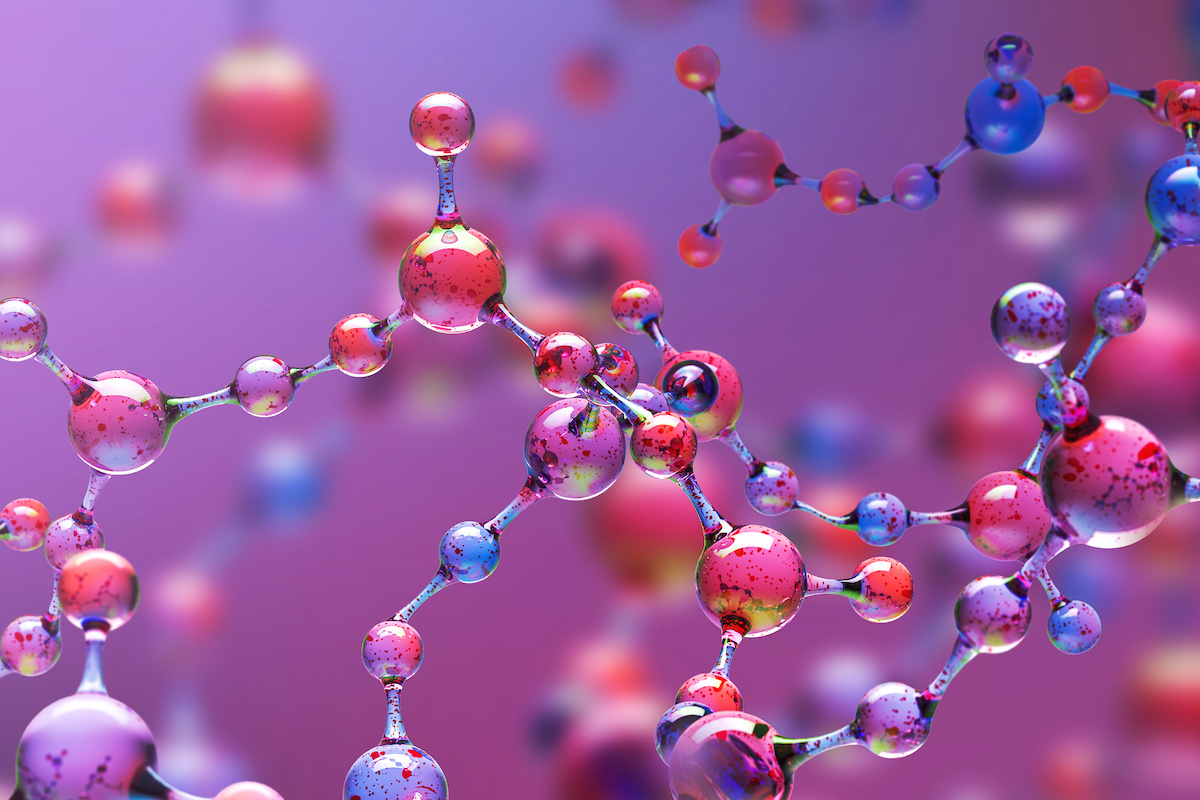
There are two keys to understanding how atoms interact.
- Understanding that each atom is made of electrically charged components: a positively charged nucleus and a series of negatively charged electrons. Even when charges are static, they create electric fields, and whenever charges are in motion, they create magnetic fields. As a result, every atom that exists can become electrically polarized when brought into the presence of an electric field, and every atom that exists can become magnetized when exposed to a magnetic field.
- Understanding, furthermore, that electrons in orbit around an atom will occupy the lowest available energy level. While the electron can be located anywhere in space within about 0.1 nanometers of the atomic nucleus (more or less), it can only occupy a certain set of values as far as energy is concerned, as dictated by the rules of quantum mechanics. The distributions of where these energy-level-dependent electrons are likely to be found are also determined by the rules of quantum mechanics, and obey a specific probability distribution, which is uniquely computable for each type of atom with any arbitrary number of electrons bound to it.
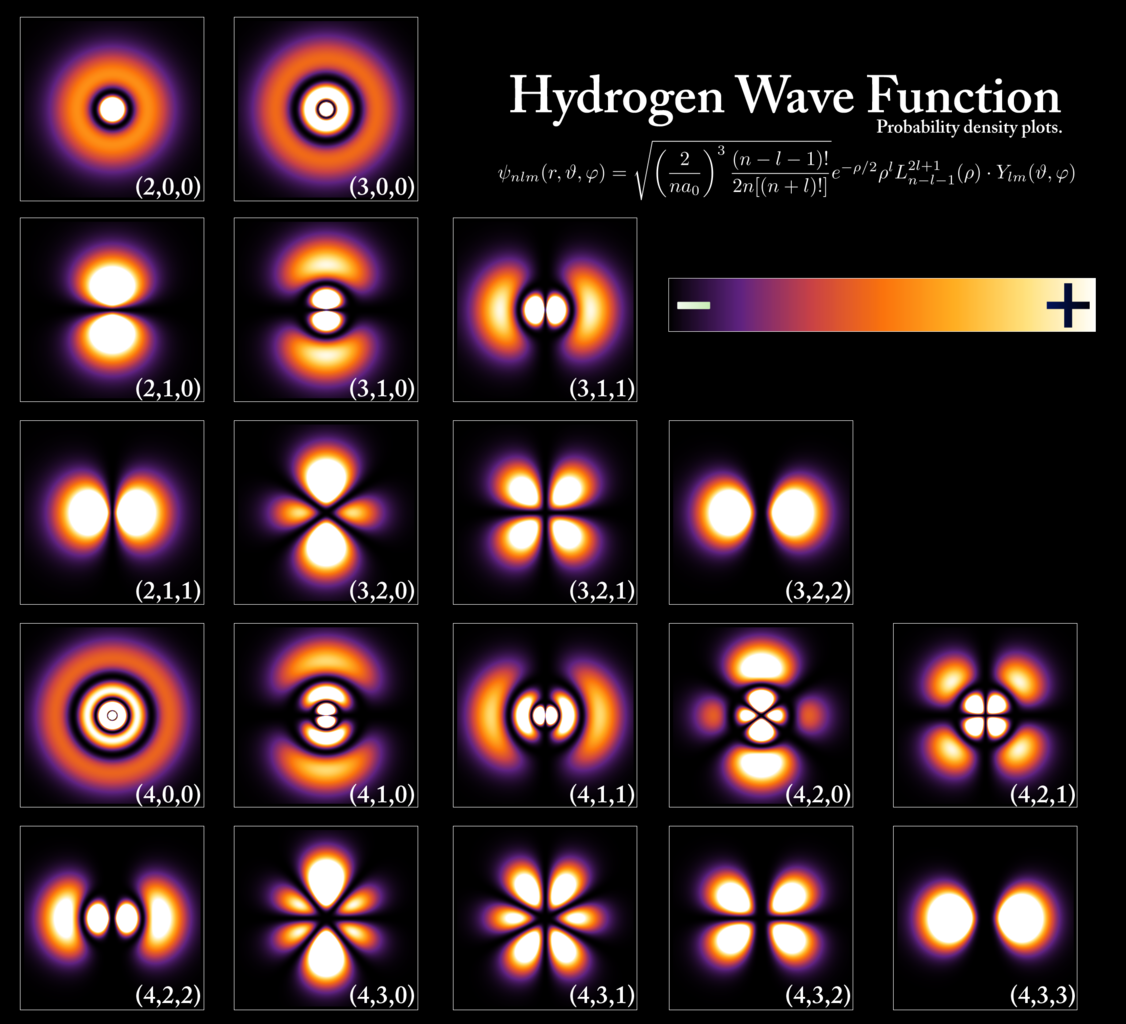
To an extremely good approximation, this view of matter within the Universe:
- that it’s made up of atoms,
- with a heavy, positively charged nucleus and light, negative charges surrounding it,
- that polarize in response to electric fields and that magnetize in response to magnetic fields,
- that can either exchange (ionically) or share (covalently) electrons with other atoms,
- forming bonds, causing polarization and magnetization, and affecting the other atoms around them,
can explain almost everything in our familiar, everyday lives.
Atoms assemble with one another to make molecules: bound states of atoms that fold together in almost innumerable sets of configurations, and that can then interact with one another in a variety of ways. Link a large number of amino acids together and you get a protein, capable of carrying out a number of important biochemical functions. Add an ion onto a protein, and you get an enzyme, capable of changing the bond structure of a variety of molecules.
And if you construct a chain of nucleic acids in just the right order, and you can encode both the construction of an arbitrary number of proteins and enzymes, as well as to make copies of yourself. With the right configuration, an assembled set of atoms will compose a living organism.
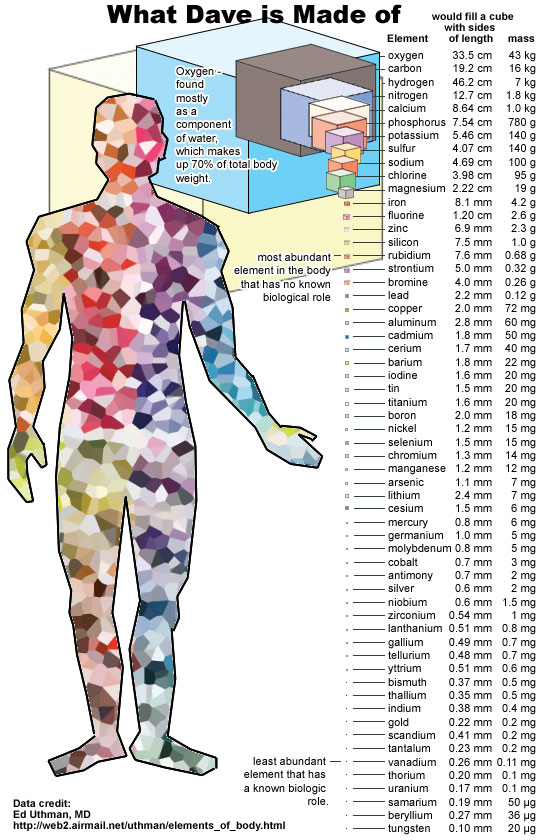
If all of human knowledge were someday wiped out in some grand apocalypse, but there were still intelligent survivors who remained, simply passing on the knowledge of atoms to them would go an incredibly long way toward helping them not only make sense the world around them, but to begin down the path of reconstructing the laws of physics and the full suite of the behavior of matter.
The knowledge of atoms would lead, very swiftly, to a reconstruction of the periodic table. The knowledge that there were “interesting” things in the microscopic world would lead to the discovery of cells, of organelles, and then of molecules and their atomic constituents. Chemical reactions between molecules and the associated changes in configurations would lead to the discovery of both how to store energy as well as how to liberate it, both biologically as well as inorganically.
What took human civilization hundreds of thousands of years to achieve could be re-discovered in a single human lifetime, and would bring fascinating hints of more to come when properties like radioactivity or the interaction possibilities between light and matter were discovered as well.
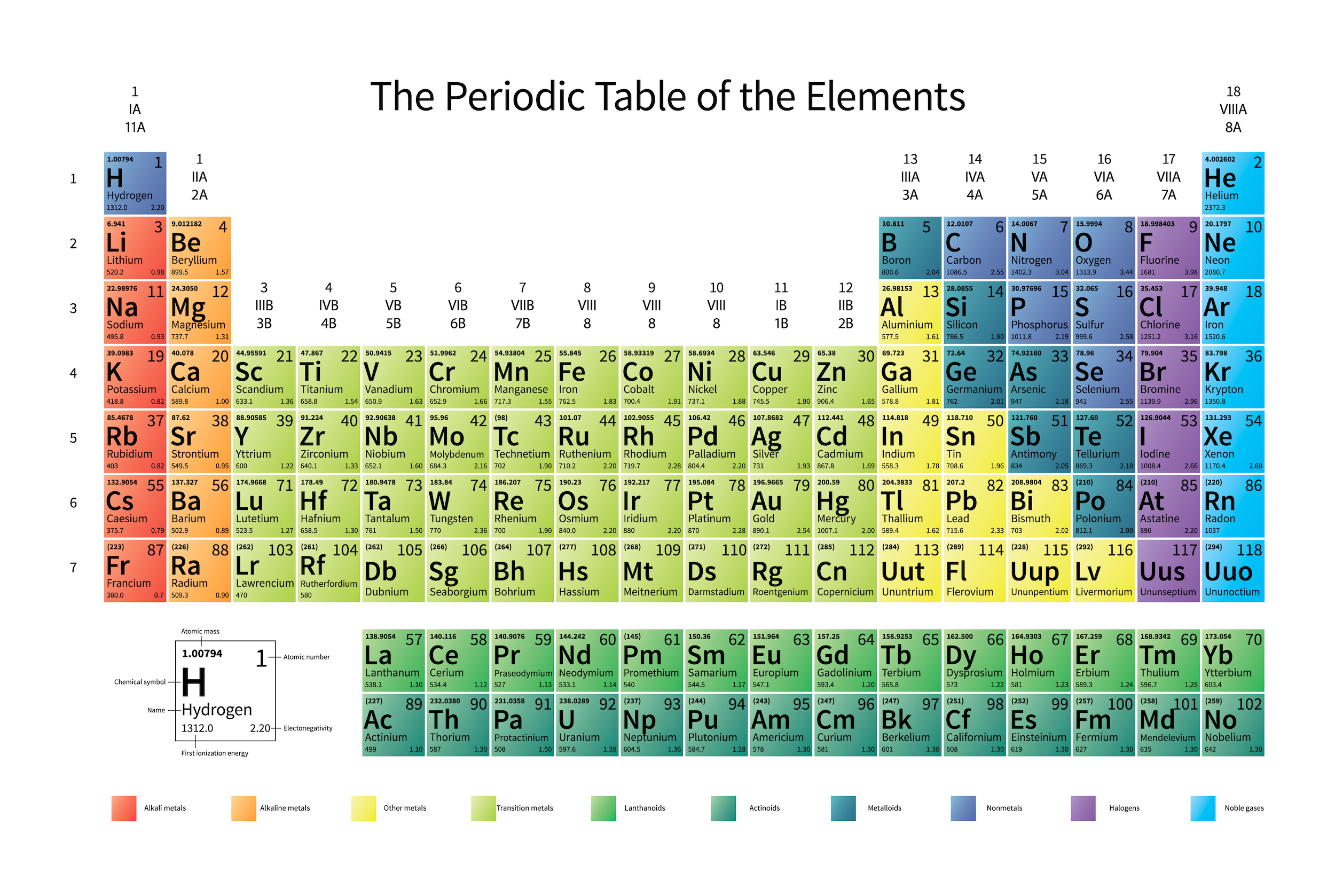
But the atom is also a sufficient key to take us beyond this Dalton-esque view of the world. Discovering that atoms could have different masses from one another but could still retain their elemental properties would lead not only to the discovery of isotopes, but would help investigators discover that atomic nuclei were composed of two different types of particles: protons (with positive charges) as well as (uncharged) neutrons.
This is more profound than almost anyone realizes, at first pass. Within the atomic nucleus, there are:
- two types of component particle,
- of almost-but-not-quite identical masses to one another,
- where the lighter one has a positive charge and the heavier one has a neutral charge,
and that the full nucleus is orbited by electrons: particles that have the equal-and-opposite charge that a proton has, and that have a smaller mass than the mass difference between the proton and the neutron inside the nucleus.
Where, if you take a free proton, it will be stable.
And if you take a free electron, it, too, will be stable.
And then, if you take a free neutron, it won’t be stable, but will decay into a proton, an electron, and (perhaps) a third, neutral particle.
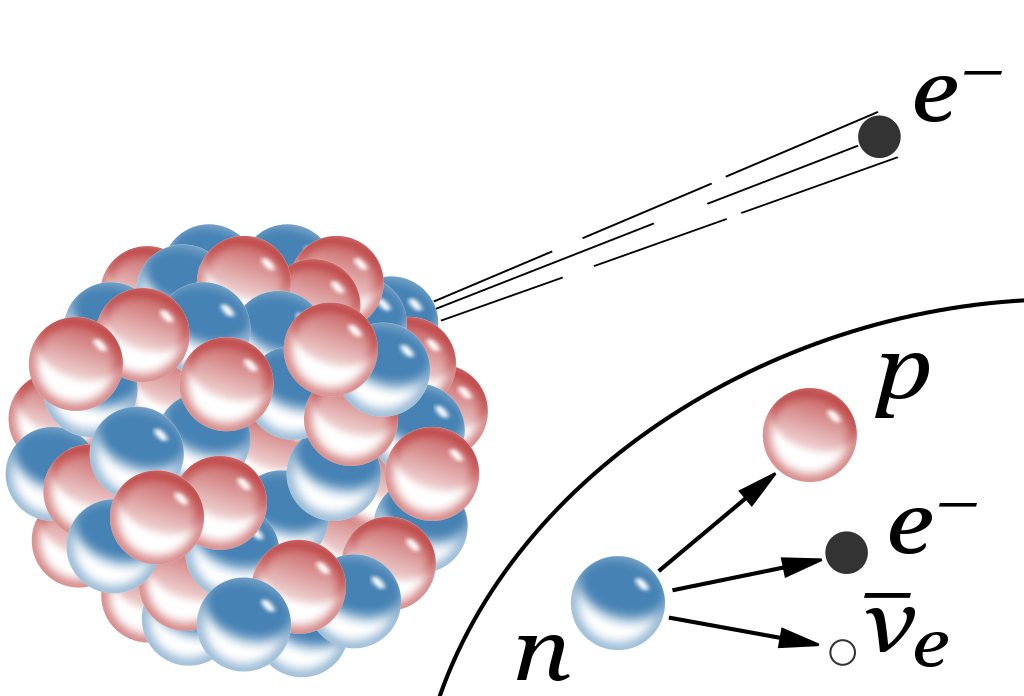
That small realization, all of a sudden, would teach you a tremendous amount about the fundamental nature of reality.
First, it would immediately tell you that there must be some additional force that exists between protons and/or neutrons than the electromagnetic force. The existence of deuterium, for example (an isotope of hydrogen with 1 proton and 1 neutron) tells us that some sort of attractive force between protons and neutrons exists, and that it cannot be explained by either electromagnetism (since neutrons are neutral) or gravity (because the gravitational force is too weak to explain this binding). Some sort of nuclear binding force must be present.
This force must, at least over some small distance range, be able to overcome the electrostatic repulsion between protons within the same atomic nucleus: in other words, it must be a stronger nuclear force than even the (quite strong in its own right) repulsive force between two protons. Because there are no stable atomic nuclei made solely out of two (or more) protons, the neutron must play a role in the stability of the nucleus.
In other words, just from discovering that atomic nuclei contain both protons and neutrons, the existence of the strong nuclear force — or something very much like it — becomes a necessity.
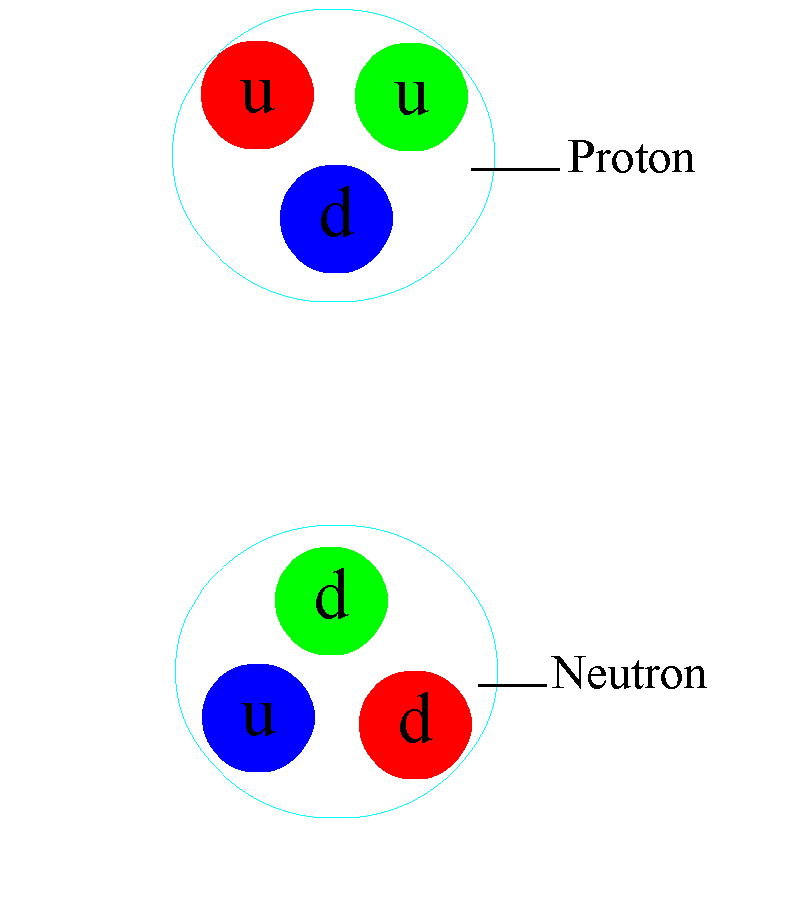
In addition, once one either:
- discovers that the free neutron can decay,
- or discovers radioactive beta decay,
- or discovers that stars are powered by nuclear fusion in their cores,
the implication is immediate for the existence of a fourth fundamental interaction in addition to gravity, electromagnetism, and the strong nuclear force: what we call the weak nuclear force.
Somehow, some sort of interaction must occur that allows one to take multiple protons, fuse them together, and then have it transform into a state that is less massive than the original two protons, where one proton gets converted into at least a neutron and a positron (an anti-electron), and where both energy and momentum are still conserved. The ability to convert one type of particle into another that’s different than “the sum of its parts” or than “the creation of equal amounts of matter-and-antimatter” is something that none of the other three interactions can accommodate. Simply by studying atoms, the existence of the weak nuclear force can be deduced.
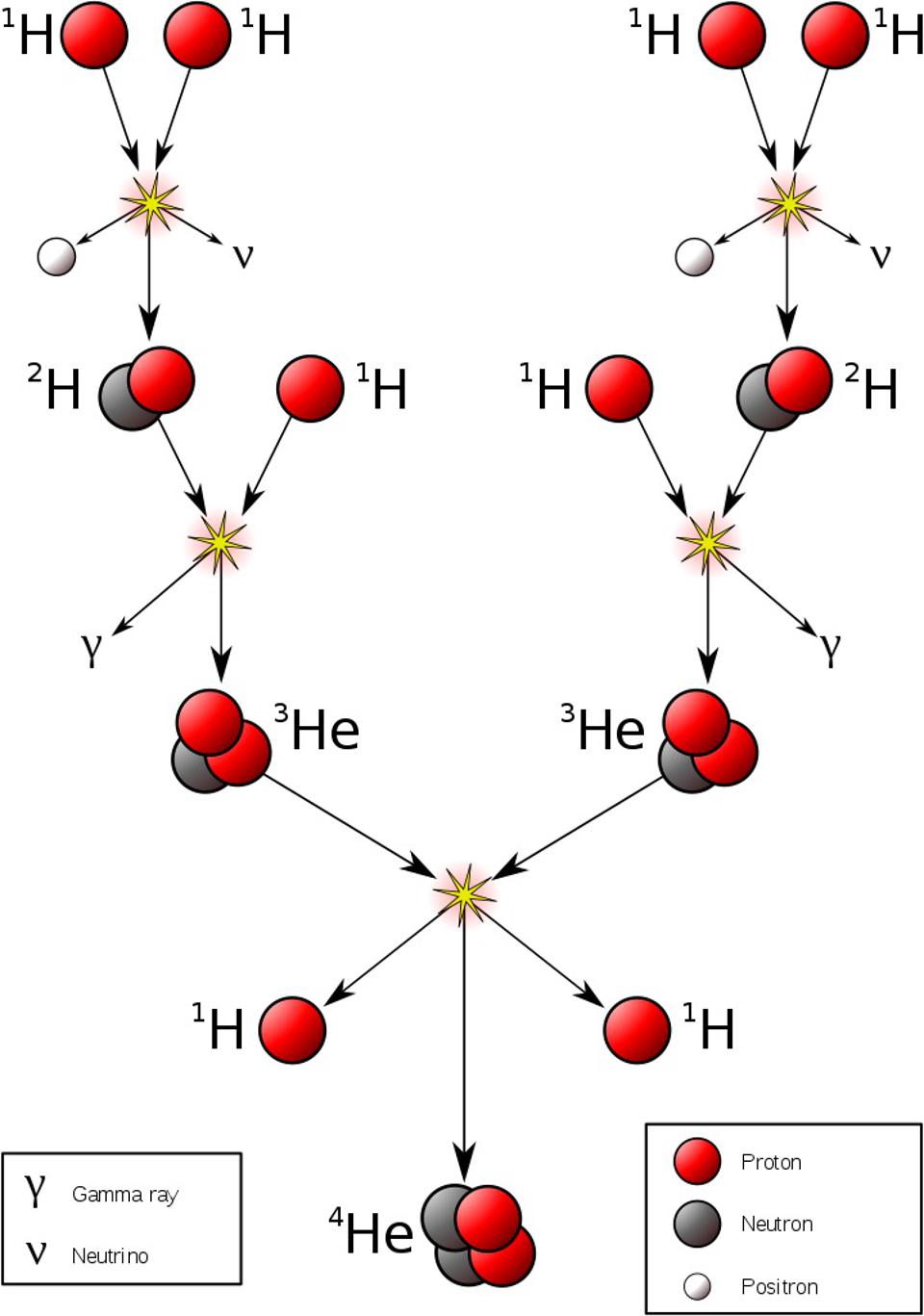
In order to have a Universe with many types of atoms, we needed our reality to exhibit a certain set of properties.
- The proton and neutron must be extremely close in mass: so close that the bound state of a proton-and-neutron together — i.e., a deuteron — must be lower in mass than two protons individually.
- The electron must be less massive than the mass difference between the proton and the neutron, otherwise the neutron would be completely stable.
- Furthermore, the electron must be much, much lighter than either the proton or neutron. If it were of comparable mass, atoms would not only be much smaller (along with all the associated structures built out of atoms), but the electron would spend so much time inside the atomic nucleus that the spontaneous reaction of a proton fusing with an electron to produce a neutron would be fast and likely, and that nearby atoms would spontaneously fuse together even under room-temperature conditions. (We see this with laboratory-created muonic hydrogen.)
- And finally, the energies achieved in stars must be sufficient for the atomic nuclei inside them to undergo nuclear fusion, but it cannot be the case that heavier and heavier atomic nuclei are always more stable, otherwise we’d wind up with a Universe filled with ultra-heavy, ultra-large atomic nuclei.
The existence of a Universe rich with a variety of atoms, but dominated by hydrogen, demands all of these factors.
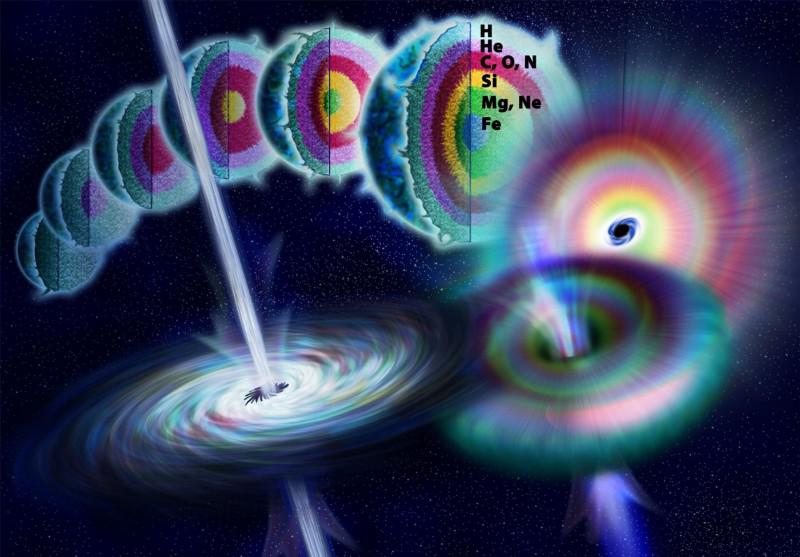
If an intelligent being from another Universe were to encounter us and our reality for the very first time, perhaps the very first thing we’d want to make them aware of was this fact: that we’re made of atoms. That within everything that’s composed of matter in this Universe are tiny, little entities — atoms — that still retain the essential characteristic properties that belong only to that specific species of atom. That you can vary the weight of the nuclei inside these atoms and still get the same type of atom, but if you vary their charge, you’ll get an entirely different atom. And that these atoms are all orbited by the number of negatively charged electrons required to precisely balance the positive charge within the nucleus.
By looking at how these atoms behave and interact, we can understand almost every molecular and macroscopic phenomenon that emerges from them. By looking at the internal components of these atoms and how they assemble themselves, we can learn about the fundamental particles, forces, and interactions that are the very basis of our reality. If there were only one piece of information to pass on to a surviving group of humans in a post-apocalyptic world, there might be no piece of information as valuable as the mere fact that we’re all made of atoms. In some sense, it’s the most miraculous property of all pertaining to our Universe.