Why are dark matter and modified gravity in such conflict?
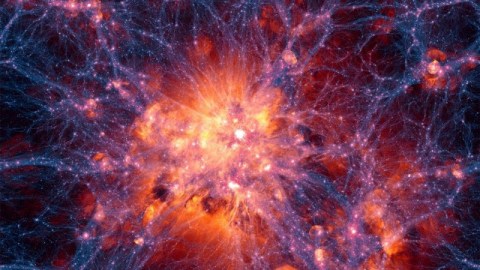
Something isn’t right about the Universe without something extra. So why can’t scientists agree on what that “extra” thing is?
“All we know so far is what doesn’t work.” –Richard Feynman
The laws of gravity are some of the most well-established and best-tested physical laws of all-time. If you made an observation of a massive object in space — a planet, star, galaxy or something even bigger — that didn’t appear to line up with what gravitation predicted, you’d be crazy not to double-and-triple-check what you saw. But every once in a while, either our laws of physics or our understanding of what’s in the Universe turn out to be incomplete, and it’s up to us to figure out the way forward. Right now, a huge academic battle is taking place between two camps seeking to fix the gravitational problems of the Universe: the dark matter camp and the modified gravity camp. This is a battle that’s played out before, with each side having historical victories to point to.
In 1781, the planet Uranus was discovered. The first large Solar System object ever discovered beyond Saturn, it was the first planet discovered with a telescope, rather than with the naked eye. Newton’s laws of gravity made very explicit predictions for how quickly a planet at Uranus’ distance from the Sun ought to move in its orbit, and so the discovery of a new world gave us a new opportunity for testing Newton’s laws. Which is why it was such an unexpected surprise when, after more than sixty years of observation, scientists found that:
- for the first 20 years, Uranus appeared to be moving too quickly for what the laws of gravity predicted,
- for the next 20 years, Uranus then appeared to move at just the right speed, matching Newton’s predictions,
- and then for all the time since, it moved too slowly, again failing to match the predictions.
What was going on? Was Newton wrong? Or was there some extra mass out there, responsible for the unexplained deviations in Uranus’ motion?
Theorists went to work on both sides of this, but the “unseen mass” idea was correct here. In 1846, Urbain Le Verrier calculated the necessary mass, location and orbital properties of what an additional, outer world beyond Uranus would cause this anomalous motion. He communicated his calculations to one of the world’s leading observatories, and on the first night they looked for it, they found a new world — Neptune — within 1º of Le Verrier’s predictions. The “unseen mass” idea held up.
But right around the same time, a new problem was noticed: one with the innermost planet: Mercury. Every planet has a perihelion, or closest’s approach to the Sun, and due to effects like the precession of the equinoxes and the gravitational pull of the planets, moons and asteroids in the Solar System, that perihelion precesses, or rotates, over time. Mercury was predicted to precess at 5557″ per century, but actually precessed at 5600″ per century: a tiny but significant difference.
Again, theorists argued over whether there would need to be a new mass — in this case, a planet interior to Mercury, code-named Vulcan — to account for the effects, or whether Newton’s laws would need to be modified. While the latter half of the 19th century was filled with calculations and searches for Vulcan, no interior world was ever found. On the other hand, the breakdown of Newton’s laws close to the speed of light, the null result of the Michelson-Morley experiment and the development of special relativity hinted at the need to go beyond Newton. In late 1915, Einstein presented the final form of General Relativity, and not only was Mercury’s extra precession of 43″ per century explained, but additional predictions were set forth as well.
As of 2016, every one of Einstein’s predictions that’s been tested has been confirmed, from gravitational time delays to lensing to pulsar orbital decays to gravitational waves. But what about the current problem, first identified in the 1930s in galaxy clusters and then more robustly in individual galaxies in the 1970s? The problem is that the internal motions of galaxies show them moving both more quickly and with a different profile than you’d expect from the normal matter and the laws of physics that we currently have. What we know, quite simply, doesn’t account for what we see.
So what’s the solution this time? Is it time to modify the laws of gravity? If you do, you can explain how pretty much every galaxy in the Universe rotates with just one simple, modified law. You can reproduce features and details that are superior to any other method, and you can even make predictions about tiny, satellite galaxies down to very, very low masses, where the matter/gravity discrepancies are greatest.
Last month, a new correlation was noticed providing a universal framework and relation between the observed, normal matter and the acceleration observed, applying to every galaxy seen and measured. For individual galaxies, modifying gravity works. But there are huge, huge drawbacks to modified gravity once you start looking at larger scales in the Universe.
When galaxy clusters collide, modified gravity makes very different pre-collision and post-collision predictions, where a single modification can’t simultaneously explain both. When you try and explain the motion of individual galaxies in clusters, modified gravity doesn’t give results that match the observations. When you look at gravitational lensing, or the bending of distant light due to gravity, modified gravity gives values that are too small. And when you look to the largest structures — the great cosmic web at late times and the fluctuations in the Big Bang’s leftover glow at early times — modified gravity’s predictions are catastrophically incompatible with what’s already been seen.
The other option is dark matter. Instead of modifying Newton’s or Einstein’s laws, you keep them intact, and you simply add an extra ingredient: some invisible, unseen mass that only interacts gravitationally. This can also explain how individual galaxies rotate, by adding in this extra source of gravitation that isn’t accounted for with normal matter. It’s a difficult, messy solution for a number of reasons, but the biggest reason is we can only simulate how this dark matter ought to behave, and our simulations have limits.
The largest scales are easier, because the Universe is more uniform, fluctuations are coarser and gravity is by far the dominant effects. You can also get away with fewer numbers of particles in your simulation to extract the relevant behavior. Dark matter, on the largest scales, reproduces the Cosmic Microwave Background, large scale structure, galaxy cluster collisions, gravitational lensing and the motions of galaxies bound into groups. Their properties are all explained perfectly by dark matter, in gory detail.
Dark matter simulations often contain trillions of particles now, and try and take into account photon pressure, star formation, supernovae and other feedback effects. But each individual galaxy is estimated to contain somewhere between 10⁶⁰ and 10⁸⁰ dark matter particles; a trillion is only 10¹². The new correlation that was found by the modified gravity camp can be explained by dark matter, but only for the most massive galaxies: those about 10% the mass of the Milky Way and larger. But galaxies less massive than that require more particles than modern computational power can deliver, and — in a new paper just out on Monday — the modified gravity team showed that there’s more than a factor of a million to go.
Most in the dark matter camp are convinced that the full suite of their successes to date mean that a better understanding of the nature of dark matter and improved computational power will lead to galaxy rotation falling into line. Similarly, most in the modified gravity camp are equally convinced that the failure of dark matter on these small scales is a catastrophe, and that the correlations they’ve discovered are a natural law that’s a precursor to a revolution even bigger than Einstein’s was 100 years ago. The great challenge for modified gravity is to reproduce the successes on large-scales of modern cosmology; the challenge for dark matter is to reproduce the details of the smallest scales correctly.
The reason there’s so much tension is that most people in the dark matter camp (full disclosure: including me) believe that what the modified gravity people call a “natural law” will someday be shown to merely be a consequence of dark matter’s existence, while the modified gravity people believe that dark matter doesn’t exist. If you want to explain the full suite of evidence we have today, you must have dark matter; there’s no known modification of gravity that can explain either the large-scale structure of the Universe or the cosmic microwave background at all. But if there is no dark matter, there must be a way to modify gravity to explain all of what the Universe gives us.
In the meantime, both sides will continue to challenge themselves and one another. Until the day dark matter is directly detected, or until the “natural laws” uncovered by modified gravity schemes are shown to arise from dark matter simulations, a little bit of uncertainty will hover over the dark matter hypothesis. As well it should. It doesn’t mean we can’t conclude “dark matter is real,” as that deserves to be the default position. But it does mean that tiny voice-of-doubt, the one that shows up on galactic scales and below, needs to be sufficiently addressed before a reasonable skeptic has no ground left to stand on.
This post first appeared at Forbes, and is brought to you ad-free by our Patreon supporters. Comment on our forum, & buy our first book: Beyond The Galaxy!