Is there phosphine, and maybe life, on Venus after all?
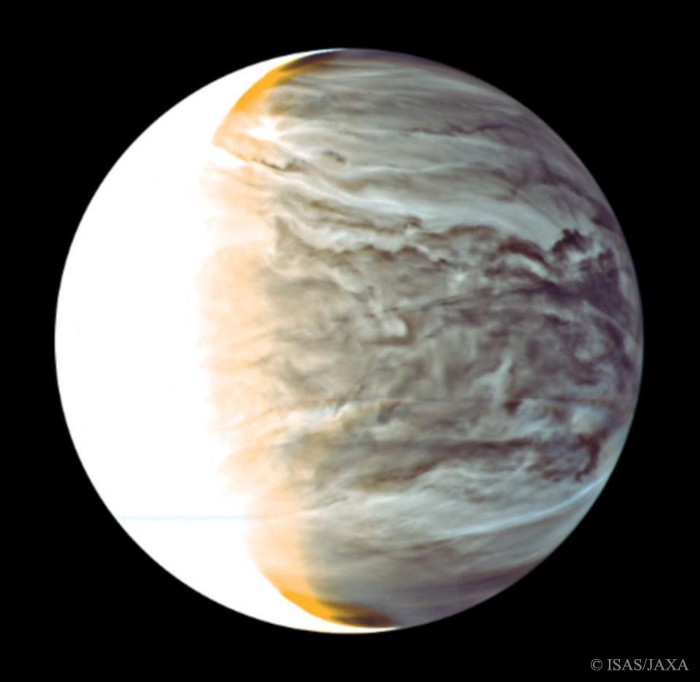
- In 2020, a team of astronomers looking at the atmosphere of Venus announced the discovery of a surprising molecule: phosphine.
- Phosphine, in such large abundance, has no known physical mechanism of generating it other than via biological activity.
- But now, two years later, phosphine’s presence is highly debated. The original discovery team is doubling down; let’s see how the evidence stacks up!
Of all the places where you can imagine that life might be hiding in this vast, immense Universe, Venus might be one of the last places you’d ever think about looking. As the hottest planet in the Solar System, hotter than even Mercury is in full sunlight, the surface of Venus is a continuous 475 °C (900 °F), day and night. These temperatures were caused by a runaway greenhouse effect that began billions of years ago, as a combination of layered cloud decks, a carbon dioxide-rich atmosphere, and rapidly circulating winds in a thick atmosphere all combined to irrevocably turn up the heat.
In 2020, a startling announcement was made: the cloud decks of Venus showed evidence for phosphine, a molecule formed as a by-product of life processes on Earth. Although its presence was hotly contested, the original team, bolstered by new data, has just presented a comprehensive case for robust abundances of phosphine in the cloud-decks of Venus. Here’s what that means, and what questions we should all be asking, today, about our nearest planetary neighbor.
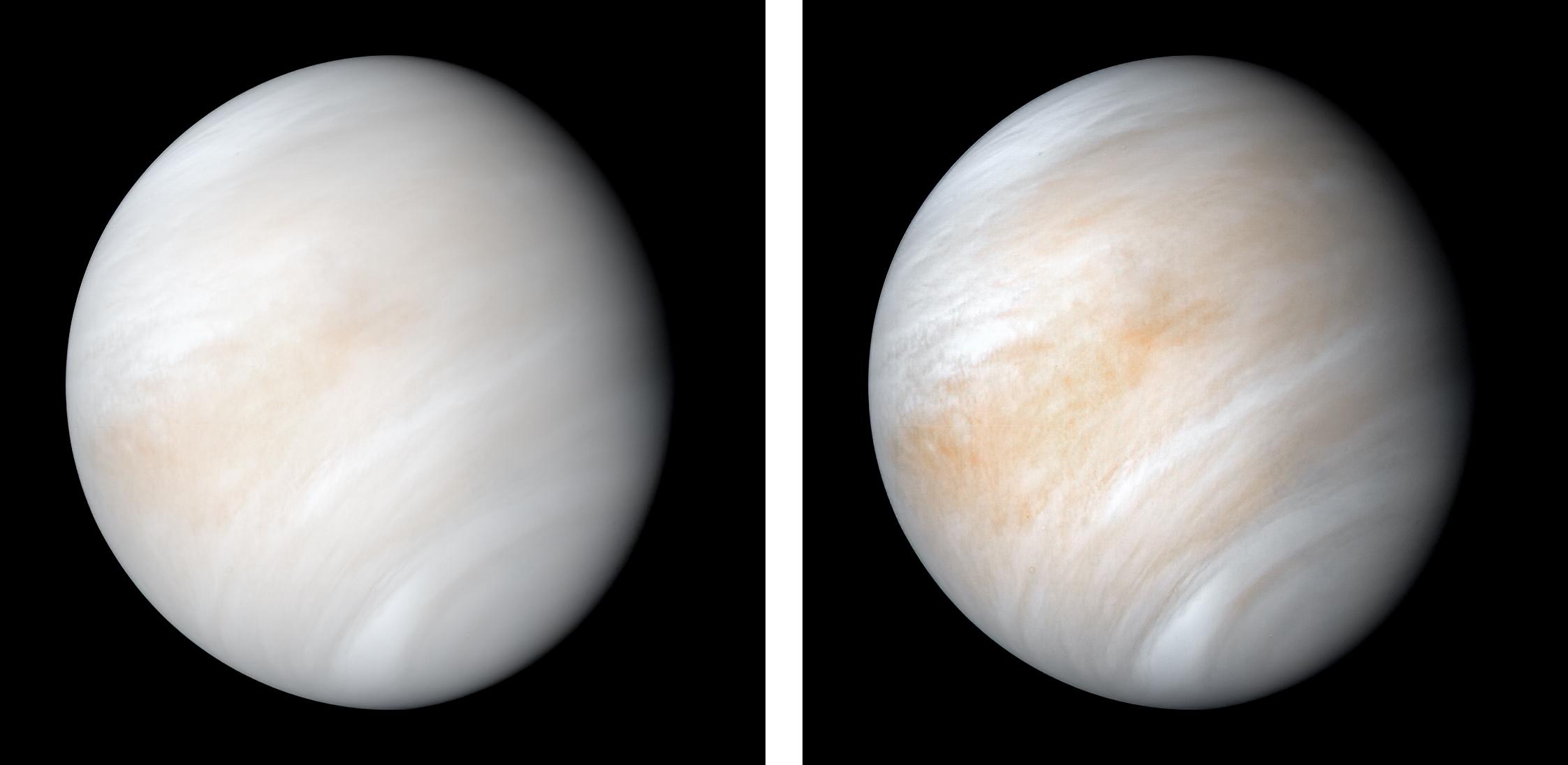
Venus, in a great many ways, is the most Earth-like planet we know of. Despite our present knowledge of more than 5000 exoplanets, Venus remains one of the only Earth-sized planets orbiting a Sun-like star at a distance where liquid water on its surface would be possible with the right atmospheric conditions. Although Venus doesn’t have the right atmospheric conditions today, having some ~90 times Earth’s atmospheric pressure, it’s possible when the Solar System was much younger, its atmosphere was much thinner. In addition, since the young Sun was cooler, the early Solar System really could have seen a temperate, wet surface on Venus early on: a potential place for life to arise, just as it did on Earth.
Despite the hostile nature of Venus’s surface, its various cloud layers — despite their strong acidity — retain the conditions of habitability. With Earth-like temperatures, atmospheric pressures similar to those found at Earth’s surface, and strong winds and chemical energy gradients, it’s possible that living organisms could be prospering within the cloud-decks of Venus. Whether life once succeeded on Venus and took refuge in the cloud-decks when the runaway greenhouse effect occurred, or whether an impact brought organisms originating on Earth to Venus’s clouds, where they survived and adapted, it remains a tantalizing possible location for potential life.
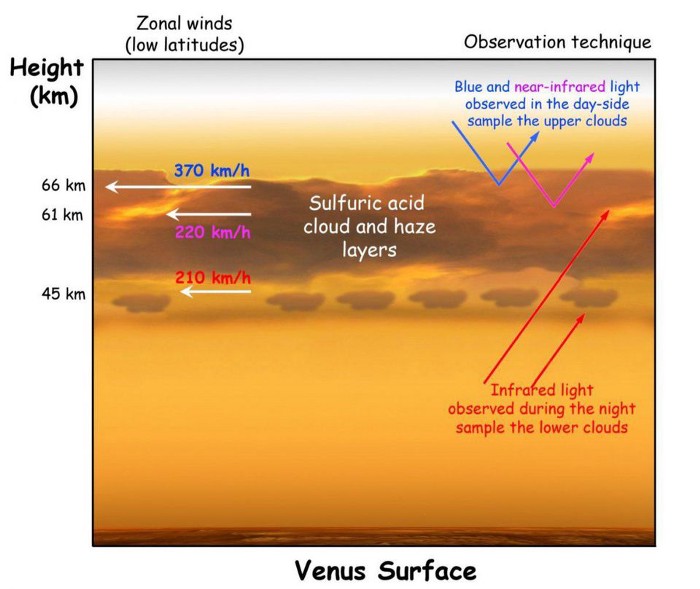
Phosphine is a molecule that would be excellent at absorbing light at particular wavelengths. Made with the same chemical composition as ammonia (NH3), except with phosphorus substituted for nitrogen, phosphine (PH3) is an asymmetric molecule that exhibits both rotational and vibrational states. If you have a molecule like phosphine that is:
- present in an energetic environment,
- silhouetted against a light-emitting background,
- and is abundant enough that its appearance can be detected above the background,
then absorption spectrocopy has a chance to reveal it.
At around a wavelength of 1.1 millimeters, phosphine exhibits exactly such a transition: from the first excited state down to the ground state. If there are clouds that emit radiation in a continuum, the phosphine present there will absorb a fraction of that radiation at that precise wavelength. Dependent on the depth of the phosphine in the cloud-decks and its overall abundance, the strength of the absorption feature tells you how that phosphine is distributed, as well as how much there is.
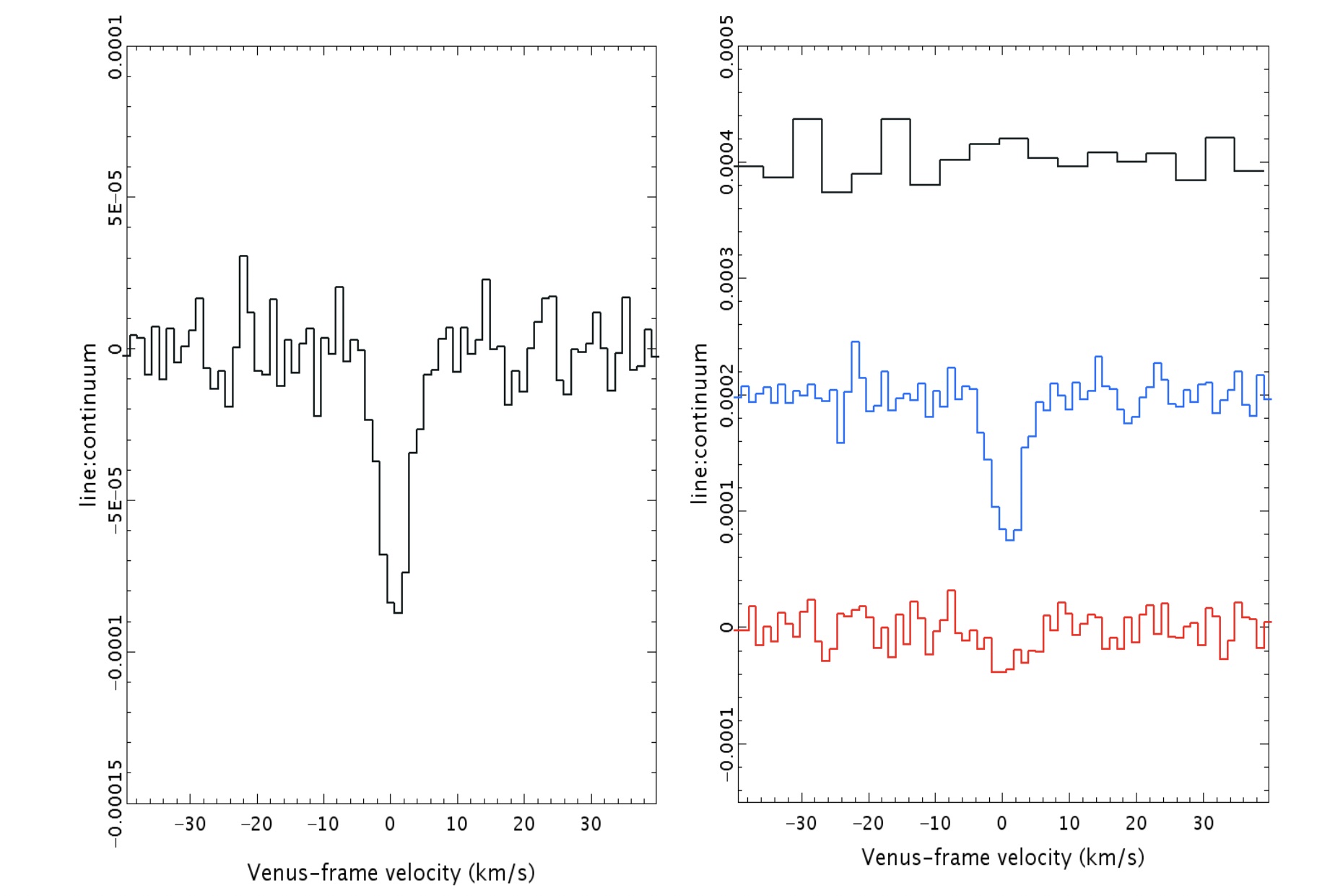
If phosphine is present, we’d then have to ask where it comes from. The element phosphorus has to come from somewhere, and finding it in a rocky planet’s atmosphere poses a bit of a challenge, since — all other things being equal — it’s a heavy molecule, and is quite difficult to make. In fact, we know of only three good ways that it’s made on Earth.
- It can be made from industrial activity, where certain chemicals are combined in the presence of one another under tightly controlled conditions. This seems unlikely for the phosphine on Venus.
- It can be made in volcanic plumes, where the phosphorus from beneath the ground is ejected high into the atmosphere. We know Venus is volcanically active, but we’ve only ever found evidence for one active volcanic flow and have never measured a positive detection for an overall phosphorus abundance in either the Venusian clouds or on the Venusian surface. If the phosphine on Venus comes from volcanoes, we’d expect a much greater amount of volcanic activity than has been suggested from the evidence so far.
- Or, there’s the third way: through the metabolization processes of anaerobic organisms.
It’s this last possibility that’s so tantalizing, and led to the proposition that phosphine could be used as a chemical marker for potential extraterrestrial life on exoplanets.
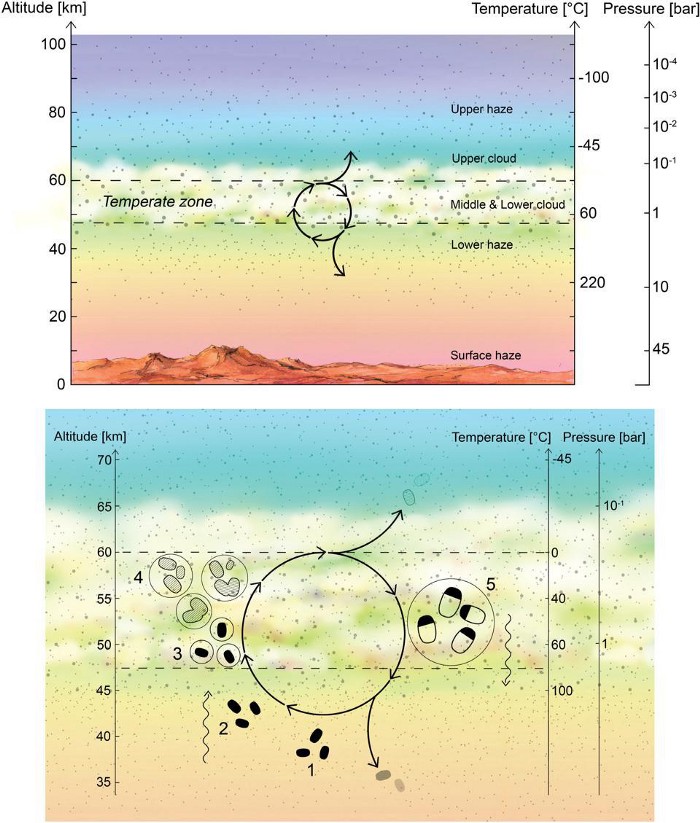
Phosphine, using radio astronomy, could be observable even from the ground if it’s present on Venus. It’s a potential biosignature of anaerobic organisms, and we know that Venus’s clouds provide a highly anaerobic envrionment. And, if it’s present at altitudes of ~55 kilometers and above, it will be capable of absorbing the light radiated from the cloud-decks found on Venus.
So, what does the data show?
There are two independent sets of data that are relevant to the problem: from the James Clerk Maxwell Telescope, the largest single-dish telescope specifically designed for sub-millimeter astronomy, and ALMA, the Atacama Large Millimeter/sub-millimeter Array, with 66 dishes all linked together. Although you won’t get spectacularly pretty images from either of these observatories, they can analyze the light coming from not just a planet, but different portions of a planet, enabling us to hone in on specific areas that might be richer or poorer in a particular molecule we’re trying to identify.
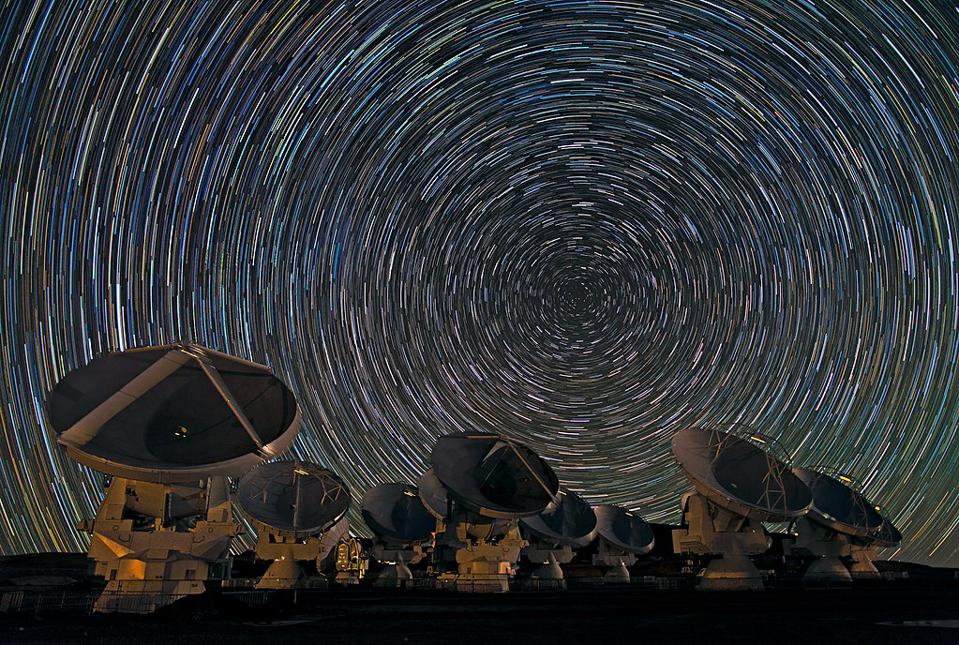
The way you get useful data about phosphine comes from performing spectroscopy: where you break up the light into a large number of individual “bins” that probe very narrow components of the electromagnetic spectrum. In each individual bin, there’s going to be an average value for the amount of signal you receive; that average value is what’s known as the “continuum.”
Each individual bin isn’t necessarily going have that precise, average value, as we perform spectroscopy by gathering individual photons and sorting them by energy/wavelength. Some bins will have greater or fewer numbers of photons than average, so if you want to say, “I’ve found a feature that corresponds to something physically real,” you have to demonstrate that, over the span of enough adjacent bins and relative to the strength of the surrounding continuum, you truly have either an absorption or emission feature.
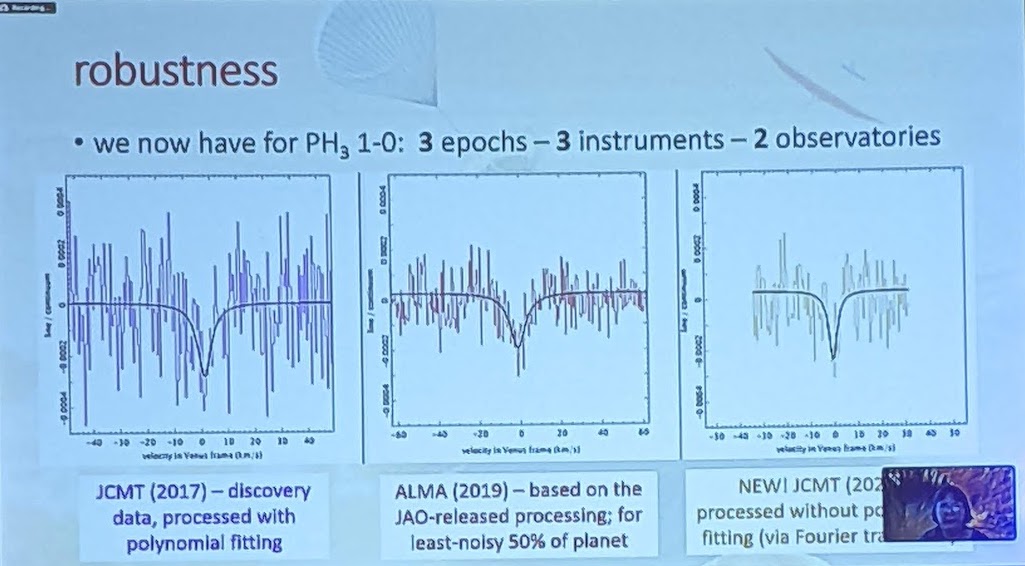
So, what did the researchers find, when they examined the data from these telescopes in great detail?
First off, there is a “dip” seen in both sets of data: from the James Clerk Maxwell Telescope and also from ALMA. In both sets of data, the dip is not only at the same wavelength, but it corresponds to the specific transition of phosphine that we’d hope to see at 1.1 millimeters. You’d be reasonable to worry that this “dip” could be a spurious feature inherent to the instrument, but that wouldn’t be the case across two different instruments.
Even though there were some questions about the polynomial fitting procedure used by the discovery team, the evidence for those absorption features still persist when one abandons the fitting procedure and instead does a simple Fourier analysis, indicating that it’s really there. And other molecules that could potentially be confounded with phosphine, like heavy water or sulfur dioxide, can be ruled out as potential contaminating sources for this signal. The James Clerk Maxwell telescope found an absorption signal that matches phosphine’s expected wavelength to 1-part-in-100,000; ALMA’s matches to 1-part-in-10,000,000.
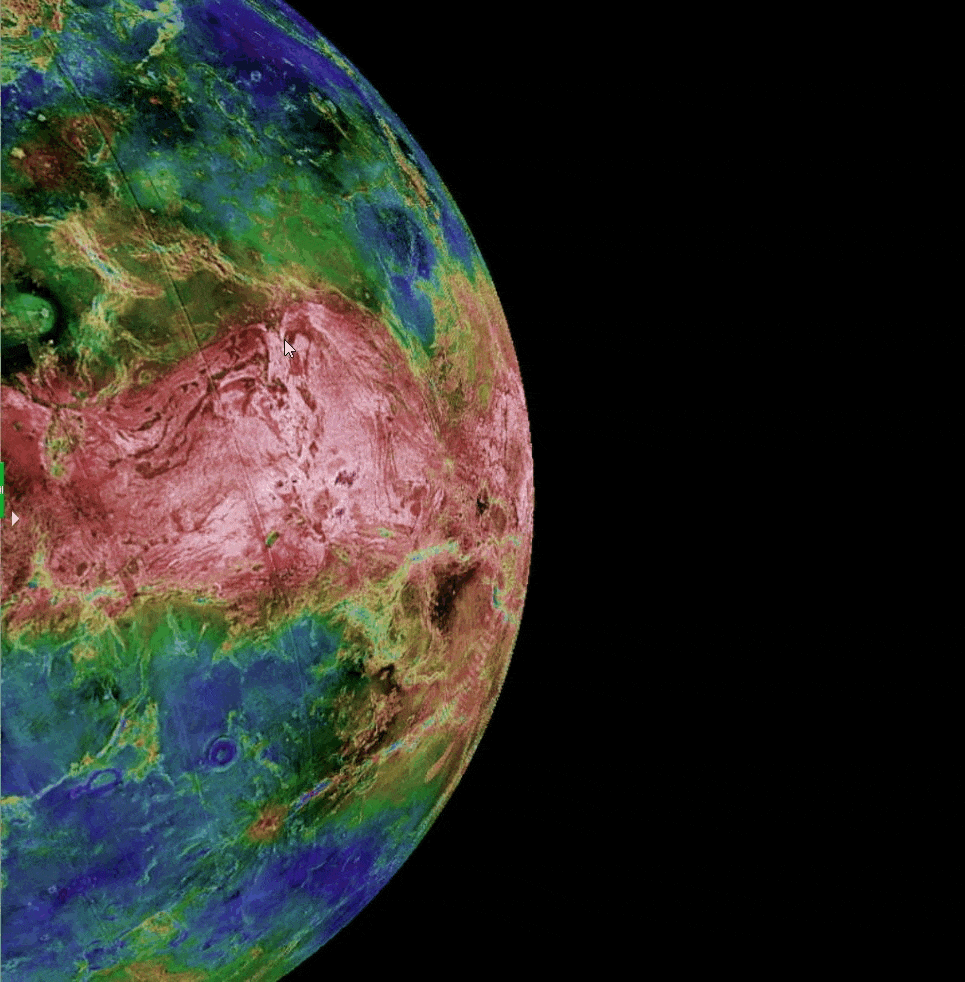
But the phosphine isn’t equally present everywhere. Infrared astronomy hasn’t detected phosphine, but has set limits on it: a maximum of a few parts-per-billion at mid-latitudes down to a few parts-per-ten-billion at polar latitudes. We also have yet to detect any other rotational or vibrational transitions of phosphine that could reveal its appearance.
But that doesn’t mean the phosphine detection isn’t to be trusted.
Consistent with the James Clerk Maxwell Telescope and ALMA data, mass spectroscopy data has given strong indications of phosphine’s presence. Sulfur dioxide, which has also been detected on Venus by the James Clerk Maxwell Telescope, and it was found using data that was taken in the same week that the phosphine data was taken. The abundance of sulfur dioxide — which many have advanced as a suspicious potential “confounding” molecule — is only about 10% what’s needed to explain the signal attributed to phosphine. This “mimicking” model has been shown to fail at reproducing the observed signal.
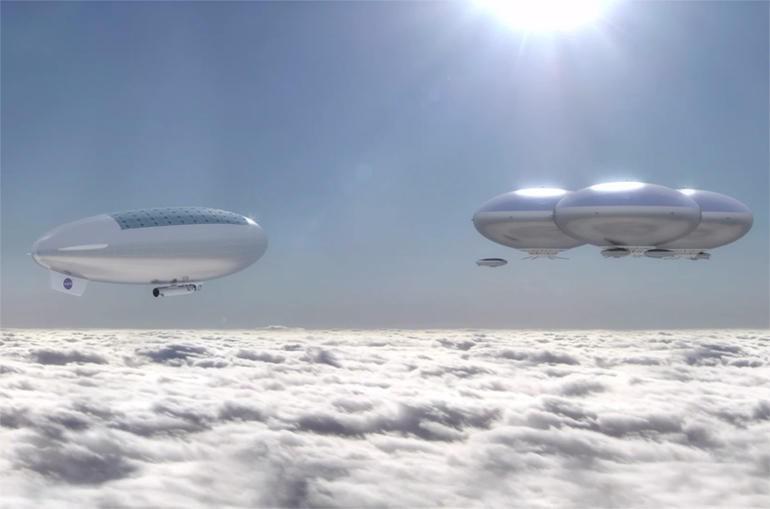
Of course, all of this suggests that there really is phosphine in Venus’s upper atmosphere, but that’s all it is: suggestive, not a certainty. You would expect, if phosphine were a biosignature, that it would follow the abundance of water, but it doesn’t. Instead, it looks like it follows the abundance of sulfur dioxide, which is unexpected, to say the least.
If you wanted to verify that this is, in fact, a phosphine signal you’d want a higher-order rotational transition from the phosphine molecules. NASA’s Stratospheric Observatory For Infrared Astronomy (SOFIA) has observed Venus, looking for precisely the 4-to-3 (instead of 1-to-0) transition. Preliminarily, they see a signal consistent with a phosphine abundance of up to ~3 parts-per-billion at altitudes of 65 km or above: potentially within the highest clouds.
Unfortunately, SOFIA is the only observatory suited to making these types of observations, and despite having a planned lifetime of 20 years, is being decommissioned on September 30th, 2022 due to costs. The requirements of needing:
- a pilot,
- a staff,
- and fuel for each flight,
translates to costs of $85 million per year for operations. Despite SOFIA’s unique capabilities, those costs are simply prohibitive when it comes to the development of new, superior missions that will be less expensive over the long-term.
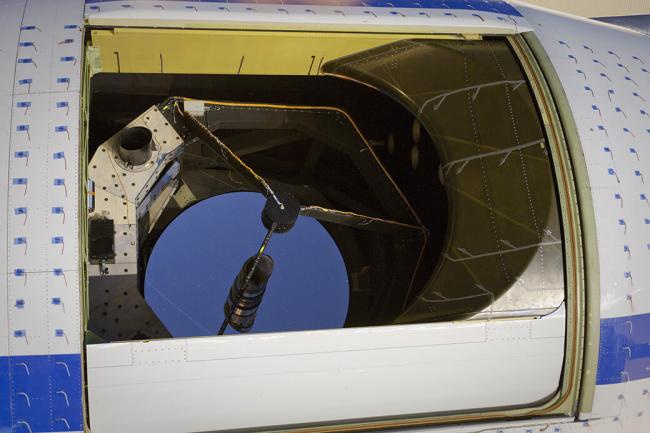
Many, both in the astronomy and planetary science communities as well as among the general public, are eager to move onto the potentially extraordinary implications of finding phosphine on Venus. If we can rule out volcanic activity or other geological sources of phosphorus as the origin of phosphine, and if we can confirm that it is, in fact, phosphine in the cloud-decks that we’re seeing, perhaps there really is some sort of anaerobic, metabolic process happening. Maybe there really is phosphine in those cloud-decks at the few parts-per-billion level, and maybe that really does imply life?
Regardless, we have no choice but to follow the data. It doesn’t prove, but it does suggest, that there may be an unexpected molecule present, in great abundance, in a potentially bio-friendly environment. Whether there turns out to be phosphine there or not — and whether it turns out to be related to life or not — it’s clear that we need a better look at Venus in order to find out. Venus is supremely important as a benchmark: there are going to be Venus-analogues out there among the exoplanets, and the more we find out about it, the more we’ll understand what we’re looking at when the time, and data, comes.