How Did The Universe Make Our Existence Possible?
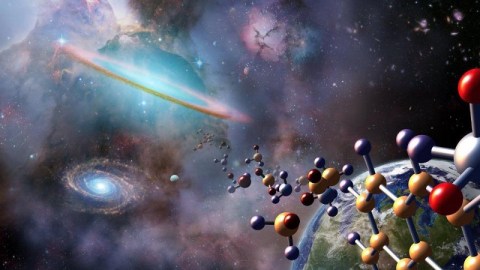
The history of the Universe is forever imprinted in our own bodies.
We can learn a lot about the history of the Universe just by looking at each of our own bodies. A fully grown adult human is an incredibly complex system, made up of trillions of cells and somewhere in the neighborhood of 1028 atoms: the building blocks of all matter on Earth. The scientific story of what it takes to make a human teaches us an enormous about about not only the evolution and history of life on Earth, but of the entire Universe as well.
It wasn’t merely billions of years of life surviving, thriving, and filling every ecological niche possible on our planet that brought us into existence, but an entire Universe. The story of how we came to be requires all sorts of cosmic predecessors, from previous generations of stars to the mergers of ancient galaxies to the Big Bang itself. Even dark matter plays an enormously important role in enabling human beings to exist in this Universe. It took 13.8 billion years for humans to come into being on Earth, and we’ve finally reconstructed the cosmic story of how we got here.
On a very basic level, we can learn what a human being is simply by looking at the tiny components — the atoms — that make up our bodies. Oxygen is the most abundant element in our body, followed by carbon, hydrogen, nitrogen and calcium. All told, there are at least 56 different elements from the periodic table that make up at least 0.1 milligrams of a typical human, with both light and heavy elements playing important roles in the body’s biological activities.
For the past 200,000 years or so, human beings have walked upon this Earth, with each generation of modern humans descended from the prior one. This is how every living creature works: it’s descended from its parent organism (or from multiple parents), with the genetic material passed on — plus whatever mutations occur — from parent to child. In an unbroken string of life going back more than four billion years on Earth, this is where every organism in existence today comes from.
All the various forms of life that ever were, however, all rely on those same ingredients that humans do: those same atoms and those same elements. They all require a stable home where they can assemble into life forms that reproduce and sustain themselves for billions of years: a rocky planet like Earth around a relatively stable star like our Sun. There’s no guarantee that the evolution of something like human beings would be inevitable, but for every planet in the Universe with similar conditions to Earth, we have to recognize that it might be possible.
The question is, then, what needed to happen in the Universe for an Earth-like planet around a Sun-like star with the right raw ingredients for life to arise? You can’t just say, “the Universe was created this way,” because that’s not how science works. In science, if you want to know the answer to a question about the Universe, you have to interrogate the Universe itself. The way we do that is through formulating hypotheses, performing experiments, making observations, and drawing conclusions.
Fortunately, that method is remarkably successful at providing the answers we seek.
The first ingredient we need are the elements required for life: the various atoms that make up the periodic table. When we look at the Earth and the other bodies in our Solar System in detail — including foreign meteorites which fall to Earth — we can determine which elements are present in which ratios, and this includes all of the elements needed for life.
By then studying the Universe, including:
- large, massive stars,
- supernova events,
- small, Sun-like stars,
- stellar remnants like white dwarfs and neutron stars,
- cosmic rays,
- and even the Big Bang itself,
we can determine where the majority of each element comes from. In order to make a Universe that allows for humans, therefore, we can conclude what’s required.
Perhaps surprisingly, the answer is all of these. Only, you can’t get them right away.
If our Universe begins with the hot Big Bang, the only elements that get created there are hydrogen, helium, and a tiny bit of lithium (element #3); nothing else. The reason is simple but restrictive: in the earliest, hottest stages, you have plenty of protons and neutrons at high energies, but you also have enough photons — or particles of light — that anytime the protons and neutrons bind together, the light comes in and splits them apart.
Only once the Universe expands and cools off sufficiently can protons and neutrons bind together to form heavier elements, and that takes time. But by that time, things are so much less dense and energetic that the electric force repelling two helium atoms is so strong that the particles can’t overcome it. We can make the lightest elements in the Big Bang, but not the heavier ones. For those, we have to wait a very, very long time: for stars to form.
It takes tens or even hundreds of millions of years for the Universe to cool enough and for gravitation to attract enough matter into individual locations to trigger the formation of stars for the first time. For that to happen, the Universe needs to:
- have been born with tiny imperfections in it, where some regions have more matter than others,
- cool enough so that stable atoms can form from the ionized atomic nuclei and free electrons,
- attract enough matter into one place so that gas clouds can collapse to form stars,
- and for that collapsing matter to radiate enough energy away so that nuclear fusion can start occurring in a star’s core.
The first part is one of the key pieces of evidence for cosmic inflation; the second part is where the cosmic microwave background that we see comes from; the third is what takes all that time — tens to hundreds of millions of years — to occur; but the fourth is a challenge.
Why?
Because normally, the way gas cools off to form stars involves radiating that energy away through their heavy elements. Without any of them present, the only way to cool off is by hydrogen gas radiating, which is horribly inefficient. As a result, the very first stars in the Universe, what astronomers call Population III stars, were very different from the stars we form today.
On average, the Universe forms a few large, heavy, massive, blue stars whenever new stars are formed, but the average new star is small: about 40% the Sun’s mass. Because of the lack of heavy elements, however, the average Population III star should be about 10 times as massive as the Sun, meaning they’re all short-lived and likely to die in a supernova explosion.
This is good, in a sense, because supernovae not only create a large fraction of heavy elements, but they also lead to the formation of neutron stars, which themselves can then merge together to produce the heaviest elements of all: elements like iodine, gold, platinum and tungsten. These first stars are important, and the fact that they make supernovae remains important, too.
But it also presents a challenge, because these early star clusters only have a little bit of matter in them, while supernovae expel material at incredibly fierce speeds. If you run the math and add up “how much material is there to form the first stars” and compare it with “how fast do supernovae eject material,” you run into a puzzle.
The ejected material is too fast for the amount of mass that’s present, meaning that these heavy elements should overwhelmingly be ejected into the intergalactic medium.
That’s bad! We need to hang onto that material so it can participate in future generations of star formation. We need it to help form:
- subsequent generations of stars, so we can get low-mass stars,
- rocky planets, so that we can have a terrestrial world like Earth rather than gas-dominated planets alone,
- and life, because we need the chemistry that these heavy elements make possible.
The normal, atom-based matter in the Universe alone isn’t enough to do this. All the gas, dust, and black holes that exist simply don’t provide us with enough gravitational force to hang onto this material. In a Universe made of atoms alone, the more massive structures that we see — structures like the one we inhabit, the Milky Way galaxy — would be impossible. In order to form them, we need an extra ingredient: dark matter.
With dark matter, these early star clusters and proto-galaxies can have enough gravitation to hang onto the ejected material from supernovae and other cataclysms, all while drawing more and more matter into them. Over time, enough heavy elements are built up that more evolved stars — with substantial fractions of heavy elements — can begin to form. These stars are lower in mass, and not only help produce many of the elements in our periodic table, but also white dwarfs, which merge and explode, leading to the formation of atoms like carbon, nitrogen, and calcium: vital elements for our bodies.
Eventually, after billions of years have gone by, individual galaxies like the Milky Way will be rich enough in these heavy elements that when new stars form, they’ll also be capable of forming rocky, Earth-like planets around them. It’s thought that, some 9.2 billion years after the Big Bang, a star-forming region in our Milky Way created a wide variety of stars, one of which would grow into our Sun. Its proto-planetary disk would wind up forming four inner, rocky planets, as well as a system of outer, gas giant planets. The third planet from that Sun, Earth, would eventually form life and lead to human beings arising.
None of this was a predestined conclusion. If we were to rewind the clock to the initial formation of our Solar System and run the clock forward again a billion times, it’s extraordinarily unlikely that human beings would arise even once. But if we were to rewind the clock back to the early stages of the hot Big Bang, a Universe filled with stars, galaxies, rocky planets, Sun-like stars, and trillions upon trillions of chances at life would be all but inevitable.
The reason is simple: the laws and raw ingredients of the Universe are always the same. A Universe born with normal matter will produce the light elements; a Universe with density imperfections will produce a first generation of stars; a Universe with dark matter will hang onto that ejected material and form stars with heavy elements; a Universe with a second generation of stars will form rocky planets and Sun-like stars; and a Universe with rocky, Earth-like planets will enable life to exist, survive, and thrive for billions of years. The rest might all be up to chance, but that’s what made our existence possible. It’s up to all of us not to squander it.
Ethan Siegel is the author of Beyond the Galaxy and Treknology. You can pre-order his third book, currently in development: the Encyclopaedia Cosmologica.