Five Years After The Higgs, What Else Has The LHC Found?

Sure, we found the Higgs Boson at the LHC earlier this decade. But what else has, and more importantly, hasn’t turned up?
It’s now just over five years since the two major collaborations at the Large Hadron Collider — CMS and ATLAS — jointly announced the discovery of a new particle with never-before-seen properties: the Higgs boson. It was the first fundamental scalar particle ever discovered, the first particle with spin = 0, the first particle with a rest energy of 126 GeV, and the last predicted, missing particle from the Standard Model of particle physics. With the discovery of the Higgs boson, that Standard Model was finally completed. All the other particles and antiparticles had previously given way to direct detection, and with the Higgs, we’ve now found every particle that we can predict ought to exist. Yet there are a huge number of unsolved mysteries in physics, and over five years later, the LHC has shown us no new hints of what’s next. Here’s a recap of what the LHC has and hasn’t found, and what it means for what’s next.
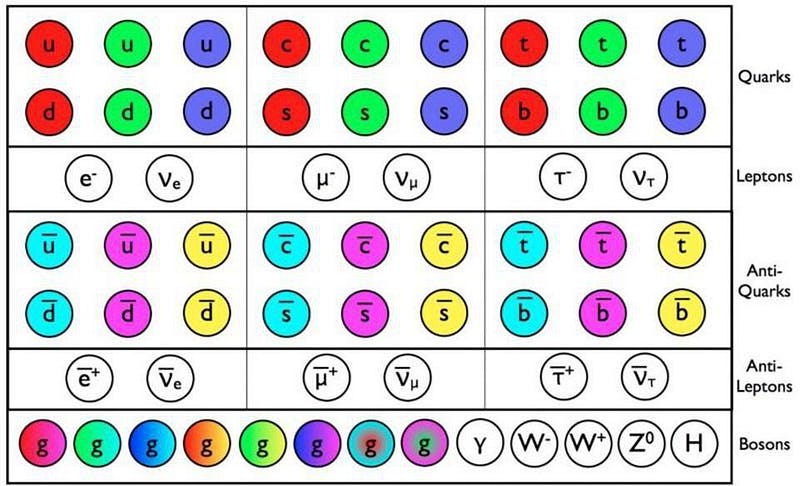
Found: The Standard model is really, really good. Every particle we’ve created at the LHC, how it’s decayed, what it’s interacted with, and what its intrinsic properties are all point to the same conclusion: everything we’ve ever seen in a collider is in 100% agreement with the Standard Model. There are no exotic decays; there are no fundamental rules that are violated; there is no indirect evidence that something more must be out there for any particles, from the Higgs to the top quark to the neutrinos. For better or for worse, there are no deviations we’ve seen from the Standard Model.
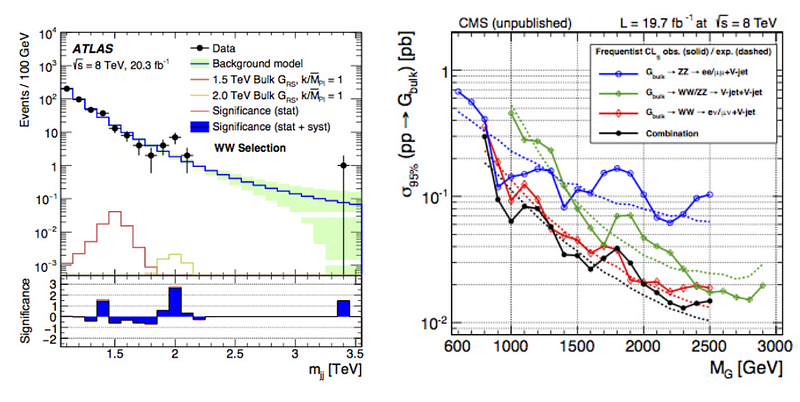
Not found: Any evidence for additional particles. There’s no sugar-coating this one: this was perhaps the greatest hope of most physicists. New particles at scales between 100 GeV and ~2 TeV were sorely hoped for, and at various times, some statistically suggestive evidence emerged for a few candidates. Unfortunately, with more and better data, this tentative evidence evaporated, and now, with Run I and Run II complete, there are not even any good suggestions of where such a new particle might be.
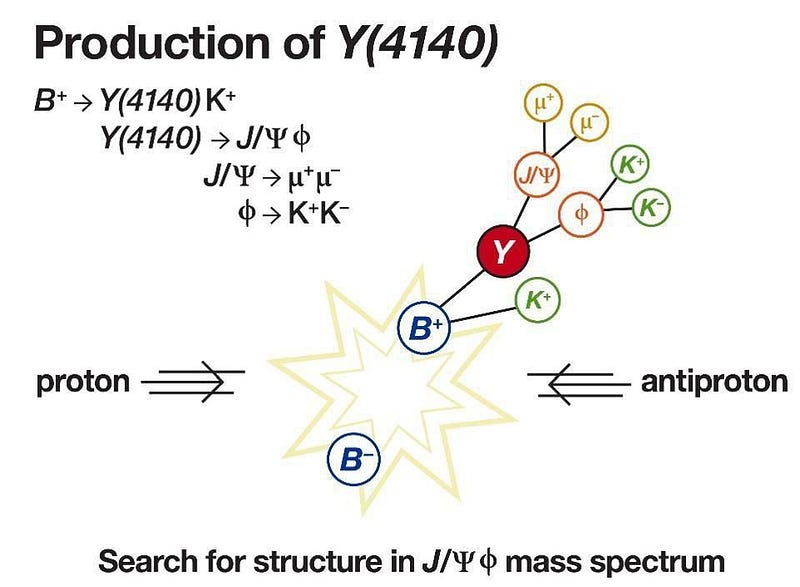
Found: New bound states of exotic particles. The rule for composite particles that are made of quarks — like the proton (up, up, down) and the neutron (up, down, down) — is that they have to be colorless: made of combinations like 3 quarks, 3 antiquarks, or a quark-antiquark combination. Since quarks come in three colors (red, green, blue) and antiquarks come in three anticolors (cyan/antired, magenta/antigreen, yellow/antiblue), and all three colors (or anticolors) together give you a colorless combination, we fully expect baryons (3 quarks), antibaryons (3 antiquarks) and mesons (quark/antiquark pairs) to exist. But we’re also starting to find tetraquark (2 quarks/2 antiquarks) and pentaquark (4 quarks/1 antiquark) states! This is a huge victory for quantum chromodynamics: the theory of the strong interactions. But, again, these are all predictions that come from the Standard Model and nothing more.
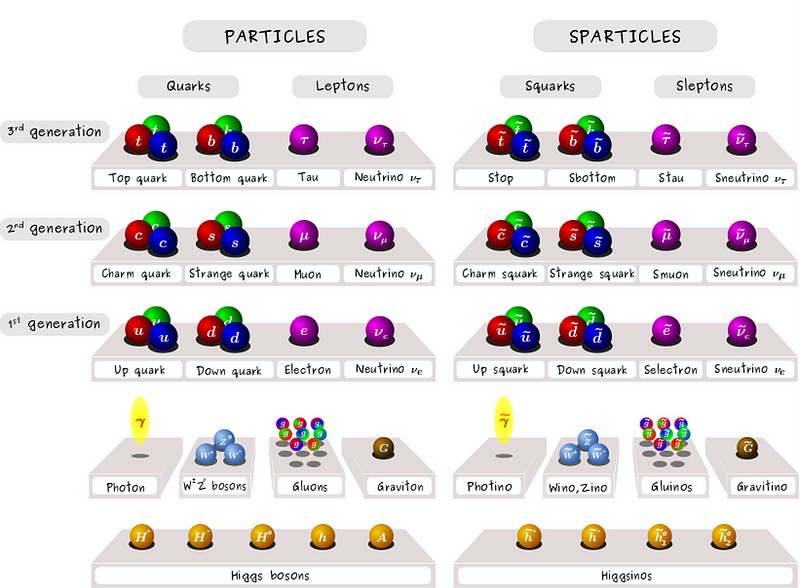
Not found: Supersymmetry. Extra dimensions. Direct creation of dark matter. These were the big theoretical hopes that many had for the LHC, and not only have direct detection efforts not panned out at the LHC, but many (or even most) of the models that were designed to solve some of the biggest problems (like the hierarchy problem) in physics have been ruled out. Nature still might have supersymmetric particles, extra dimensions, or particle-based dark matter, but the most promising versions of these extensions to theory have failed to show up at the LHC. They still might, of course, but there’s not even indirect evidence suggesting that further data will reveal them at the LHC’s energies.

Found: CP-violating decays. Sure, we had seen these before in small amounts, but the LHC is bringing us evidence of additional CP-violation in composite particles involving the strange, bottom, or even charm quarks. CP-violation is a measure of how particles behave differently, in certain ways, from their antiparticles. One of the intriguing differences is that if particles can decay via two different pathways, their antiparticles must decay by their anti-pathway counterparts, but can prefer one pathway over the other in a different way from what particles prefer. The amount of CP-violation in the b-quarks in particular is larger than we expected, which could be important for matter/antimatter differences in the Universe. But that said…

Not found: An answer to the baryogenesis problem. Is there new physics that happens at the electroweak scale? Is there hope for the Affleck-Dine mechanism? If either of these are correct, the LHC could reveal these potential hints. The lack of such hints tells us that the origin of the matter/antimatter asymmetry may exist in a different scenario, like leptogenesis or through the existence of superheavy bosons, but there is still a lot of TeV-scale physics to explore. With the earlier hints of much more CP-violation in the b-quark sector than we’d realized, the LHC may yet shed some important light on this great unsolved problem in physics.
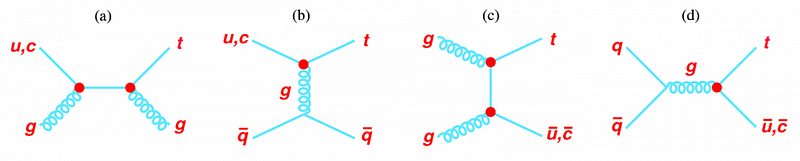
Found: Neutral current conservation. This was a huge prediction of the Standard Model that tightly constrains many beyond-the-Standard-Model extensions. If you could turn a bottom quark into a strange or down quark, a top into a charm or up quark, or a tau into a muon or electron through the exchange of a neutral boson (like the Z⁰), that would be an example of a flavor-changing neutral current. The Standard Model forbids these; they only exist in theories that add additional particles and interactions, such as Grand Unified Theories. So far, all neutral currents are still shown to be conserved, a huge victory for the Standard Model. This may disappoint some people who’ve invested heavily in particular variants of beyond-the-Standard-Model physics, but understanding the Universe better is good news for physicists everywhere.
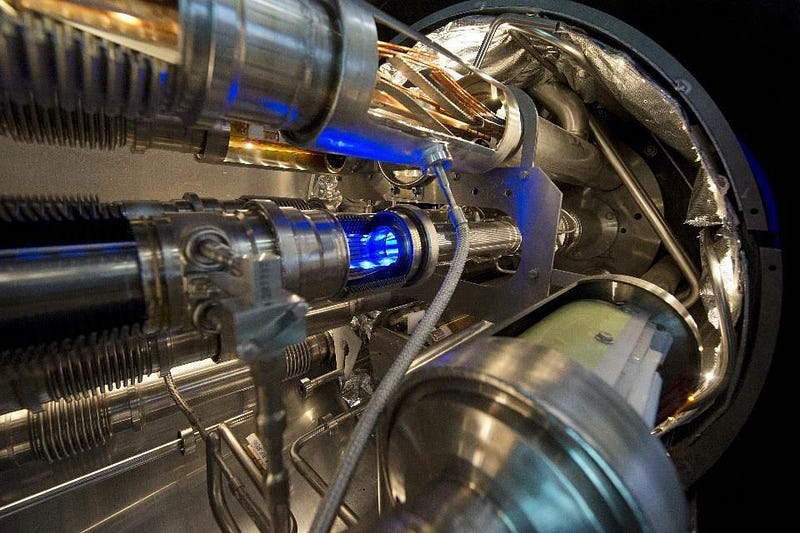
But here’s the biggest thing you have to remember about the LHC: even five years after we’ve discovered the Higgs boson, we’ve still only collected approximately 2% of the data it’s going to collect over its lifetime. If there are unusual decays, additional particles, new physics at the electroweak scale, a coupling between heavy particles and new physics (sterile neutrinos, the dark sector, exotic/undiscovered matter), etc., we’ll have 50 times as much data coming in over the coming 15–20 years to look for it. The biggest worry, perhaps, is that there is new, interesting physics here, but because we can only save about 0.0001% of the collision data, we’re unknowingly throwing it away.
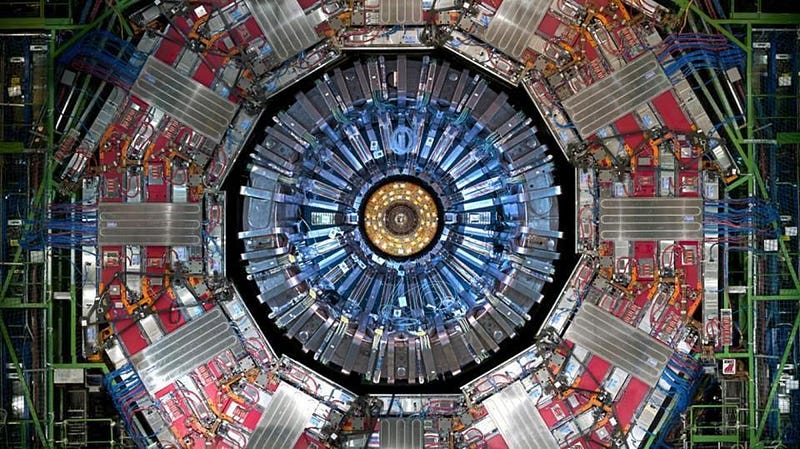
A lot of physicists are understandably concerned that the LHC hasn’t yet turned up evidence for physics beyond the Standard Model, and that the Higgs boson itself looks depressingly in line with exactly what these well-established predictions would indicate. But this shouldn’t be a surprise! We already know there is physics beyond the Standard Model, and we know it isn’t easy to find. As Tim Gershon wrote in the CERN Courier:
So far the Higgs boson indeed looks SM-like, but some perspective is necessary. It took more than 40 years from the discovery of the neutrino to the realisation that it is not massless and therefore not SM-like; addressing this mystery is now a key component of the global particle-physics programme. Turning to my own main research area, the beauty quark — which reached its 40th birthday last year — is another example of a long-established particle that is now providing exciting hints of new phenomena… One thrilling scenario, if these deviations from the SM are confirmed, is that the new physics landscape can be explored through both the b and Higgs microscopes.
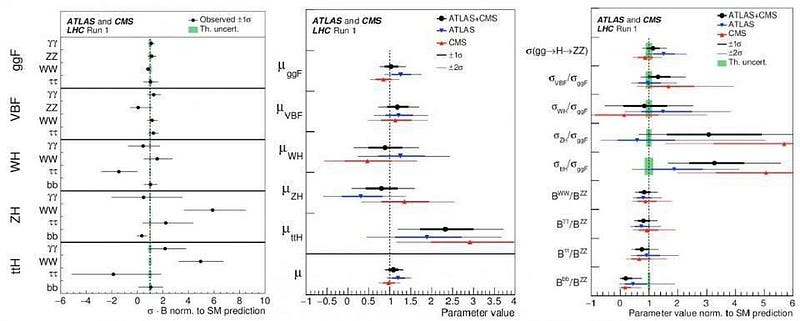
There is every reason to be optimistic, since the LHC will produce tons of b-mesons and b-baryons, as well as more Higgs bosons than every other particle source combined. Sure, the biggest breakthrough we could hope for would be the detection of a brand new particle, and evidence for one of the great theoretical breakthroughs that have dominated particle physics in recent decades: supersymmetry, extra dimensions, technicolor, or grand unification. But even in the absence of that, there is plenty to learn, at a fundamental level, about how the Universe works. There are plenty of indicators that nature plays by rules we have not yet fully discovered, and that’s more than enough motivation to keep looking. We already have the machine, and the data will be on its way in unprecedented amounts very soon. Whatever new hints are hiding at the TeV scale will soon be within reach.
Ethan Siegel is the author of Beyond the Galaxy and Treknology. You can pre-order his third book, currently in development: the Encyclopaedia Cosmologica.