Ask Ethan: What’s so “anti” about antimatter?
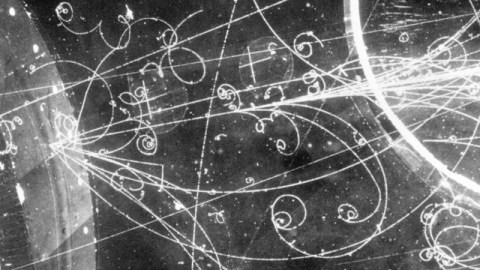
There are lots of properties inherent to particles, and while everyone has an antiparticle, not everyone is matter or antimatter.
For every particle of matter that’s known to exist in the Universe, there’s an antimatter counterpart. Antimatter has many of the same properties as normal matter, including the types of interaction it undergoes, its mass, the magnitude of its electric charge, and so on. But there are a few fundamental differences as well. Yet two things are certain about matter-antimatter interactions: if you collide a matter particle with an antimatter counterpart, they both immediately annihilate away to pure energy, and if you undergo any interaction in the Universe that creates a matter particle, you must also create its antimatter counterpart. So what makes antimatter so “anti,” anyway? That’s what Robert Nagle wants to know, as he asks:
On a fundamental level, what is the difference between matter and its counterpart antimatter? Is there some sort of intrinsic property that causes a particle to be matter or antimatter? Is there some intrinsic property (like spin) that distinguishes quarks and antiquarks? What what puts the ‘anti’ in anti matter?
To understand the answer, we need to take a look at all the particles (and antiparticles) that exist.
This is the Standard Model of elementary particles: the full suite of discovered particles in the known Universe. There are generally two classes of these particles, the bosons, which have integer spins (…, -2, -1, 0, +1, +2, …) and are neither matter nor antimatter, and the fermions, which have half-integer spins (…, -3/2, -1/2, +1/2, +3/2, …) and must either be “matter-type” or “antimatter-type” particles. For any particle you can think about creating, there are going to be a slew of inherent properties to it, defined by what we call quantum numbers. For an individual particle in isolation, this includes a number of traits you’re likely familiar with, as well as some that you may not be familiar with.
The easy ones are things like mass and electric charge. An electron, for example, has a rest mass of 9.11 × 10^–31 kg, and an electric charge of -1.6 × 10^–19 C. Electrons can also bind together with protons to produce a hydrogen atom, with a series of spectral lines and emission/absorption features based on the electromagnetic force between them. Electrons have a spin of either +1/2 or -1/2, a lepton number of +1, and a lepton family number of +1 for the first (electron) of the three (electron, mu, tau) lepton families. (We’re going to ignore numbers like weak isospin and weak hypercharge, for simplicity.)
Given these properties of an electron, we can ask ourselves what the antimatter counterpart of the electron would need to look like, based on the rules governing elementary particles.
The magnitudes of all the quantum numbers must remain the same. But for antiparticles, the signs of these quantum numbers must be reversed. For an anti-electron, that means it should have the following quantum numbers:
- a rest mass of 9.11 × 10^–31 kg,
- an electric charge of +1.6 × 10^–19 C,
- a spin of (respectively) either -1/2 or +1/2,
- a lepton number of -1,
- and a lepton family number of -1 for the first (electron) lepton family.
And when you bind it together with an antiproton, it should produce exactly the same series of spectral lines and emission/absorption features that the electron/proton system produced.
All of these facts have been verified experimentally. The particle matching this exact description of the anti-electron is the particle known as a positron! The reason why this is necessary comes when you consider how you make matter and antimatter: you typically make them from nothing. Which is to say, if you collide two particles together at a high enough energy, you can often create an extra “particle-antiparticle” pair out of the excess energy (from Einstein’s E = mc2), which conserves energy.
But you don’t just need to conserve energy; there are a slew of quantum numbers you also have to conserve! And these include all of the following:
- electric charge,
- angular momentum (which combines “spin” and “orbital” angular momentum; for individual, unbound particles, that’s only “spin”),
- lepton number,
- baryon number,
- lepton family number,
- and color charge.
Of these intrinsic properties, there are two that define you as either “matter” or “antimatter,” and those are “baryon number” and “lepton number.”
If either of those numbers are positive, you’re matter. That’s why quarks (which each have baryon number of +1/3), electrons, muons, taus, and neutrinos (which each have lepton number of +1) are all matter, while antiquarks, positrons, anti-muons, anti-taus, and anti-neutrinos are all antimatter. These are all the fermions and antifermions, and every fermion is a matter particle while every antifermion is an antimatter particle.
But there are also the bosons. There are gluons which have for their antiparticles the gluons of the opposite color combinations; there is the W+ which is the antiparticle of the W- (with opposite electric charge), and there are the Z0, the Higgs boson, and the photon, which are their own antiparticles. However, bosons are neither matter nor antimatter. Without a lepton number or baryon number, these particles may have electric charges, color charges, spins, etc., but no one can rightfully call themselves either “matter” or “antimatter” and their antiparticle counterpart the other one. In this case, bosons are simply bosons, and if they have no charges, then they’re simply their own antiparticles.
So what puts the “anti” in antimatter? If you’re an individual particle, then your antiparticle is the same mass as you with all the opposite conserved quantum numbers: it’s the particle that’s capable of annihilating with you back to pure energy if ever the two of you meet. But if you want to be matter, you need to have either positive baryon or positive lepton number; if you want to be antimatter, you must have either negative baryon or negative lepton number. Beyond that, there’s no known fundamental reason for our Universe to have favored matter over antimatter; we still don’t know how that symmetry was broken. (Although we have ideas.) If things had turned out differently, we’d probably call whatever we were made of “matter” and its opposite “antimatter,” but who gets which name is completely arbitrary. As in all things, the Universe is biased towards the survivors.
Send in your Ask Ethan questions to startswithabang at gmail dot com!
Ethan Siegel is the author of Beyond the Galaxy and Treknology. You can pre-order his third book, currently in development: the Encyclopaedia Cosmologica.