The grand paradox at the heart of Stephen Hawking’s cosmology
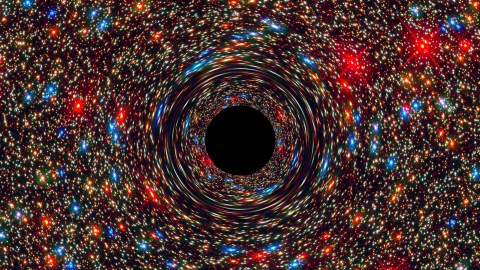
- The mind-scrambling paradoxes of black holes and the multiverse have been hotly debated by physicists in recent decades.
- Many attempts to align the two pillars of 20th-century physics — relativity and quantum theory — have been crushed by the jaws of contradiction.
- The multiverse paradox was central to Stephen Hawking’s quantum perspective on the cosmos.
Physicists say the multiverse saddles us with a paradox. Multiverse cosmology builds on cosmic inflation, the idea that the universe underwent a short burst of rapid expansion in its earliest stages. Inflationary theory has had a wealth of observational support for some time but has the inconvenient tendency to generate not one but a great many universes. And because it doesn’t say which one we should be in — it lacks this information — the theory loses much of its ability to predict what we should see. This is a paradox. On the one hand, our best theory of the early universe suggests we live in a multiverse. At the same time, the multiverse destroys much of the predictive power of this theory.
As a matter of fact, this wasn’t the first time Stephen [Hawking] was confronted with a mystifying paradox. Back in 1977 he put his finger on a similar conundrum having to do with the fate of black holes. Einstein’s general relativity theory predicts that nearly all information about anything falling into a black hole remains forever hidden inside. But Stephen discovered that quantum theory adds a paradoxical twist to this story. He found that quantum processes near the surface of a black hole cause the hole to radiate a slight but steady stream of particles, including particles of light. This radiation — now known as Hawking radiation — is too faint to be detected physically, but even its mere existence is inherently problematic.
The reason is that if black holes radiate energy, then they must shrink and eventually disappear. What happens to the huge amount of information hidden inside when a black hole radiates its last ounce of mass? Stephen’s calculations indicated that this information would be lost forever. Black holes, he argued, are the ultimate trash cans. However, this scenario contradicts a basic principle of quantum theory that dictates that physical processes can transform and scramble information but never irreversibly obliterate information. Once again we arrive at a paradox: Quantum processes cause black holes to radiate and lose information, yet quantum theory says this is impossible.
The paradoxes to do with the life cycle of black holes and with our place in the multiverse became two of the most vexing and hotly debated physics puzzles of the last decades. They are concerned with the nature and fate of information in physics and thus strike at the heart of the question as to what physical theories are ultimately about. Both paradoxes emerge in the context of so-called semiclassical gravity, a theoretical description of gravity pioneered by Stephen and his Cambridge gang in the mid-1970s, based on an amalgam of classical and quantum thinking.
The paradoxes come about when one applies such semiclassical thinking either over exceedingly long timescales (in the case of black holes) or out to exceedingly large distances (in the case of the multiverse). Together they embody the profound difficulties that arise when we try to get the two pillars of twentieth-century physics, relativity and quantum theory, to work in harmony. In this role they have served as mind-bending thought experiments, with which theorists have extrapolated their semiclassical thinking about gravity to the extreme to see where and how exactly it would break down.
Thought experiments were always a favorite of Stephen’s. Having renounced philosophy, Stephen loved to experiment with some of the deep philosophical questions — whether time had a beginning, whether causality was fundamental, and, most ambitious of all, how we as “observers” fit into the cosmic scheme. And he did so by framing these questions as clever experiments in theoretical physics. Three of Stephen’s landmark discoveries all resulted from ingenious, carefully configured thought experiments. The first of these was his series of big bang singularity theorems in classical gravity; second, his 1974 discovery in semiclassical gravity that black holes radiate; and third, his no-boundary proposal, also in semiclassical gravity, for the origin of the universe.
Now, while one could argue that the black hole paradox is of academic interest only — the fine details of Hawking radiation are unlikely ever to be measurable — the multiverse paradox bears directly on our cosmological observations. At the heart of the paradox lies the fraught relation in modern cosmology between the living world and observership, and the physical universe. The multiverse paradox became a beacon in Hawking’s quest to re-envision this relationship by developing a fully quantum perspective on the cosmos. His final theory of the universe, thoroughly quantum, redraws the basic foundations of cosmology and is Hawking’s fourth great contribution to physics. The grand thought experiment that lies behind the theory had in some sense been five centuries in the making. Carrying it out would be our voyage.