Fish aren’t as stupid as we thought — they can count (sort of)
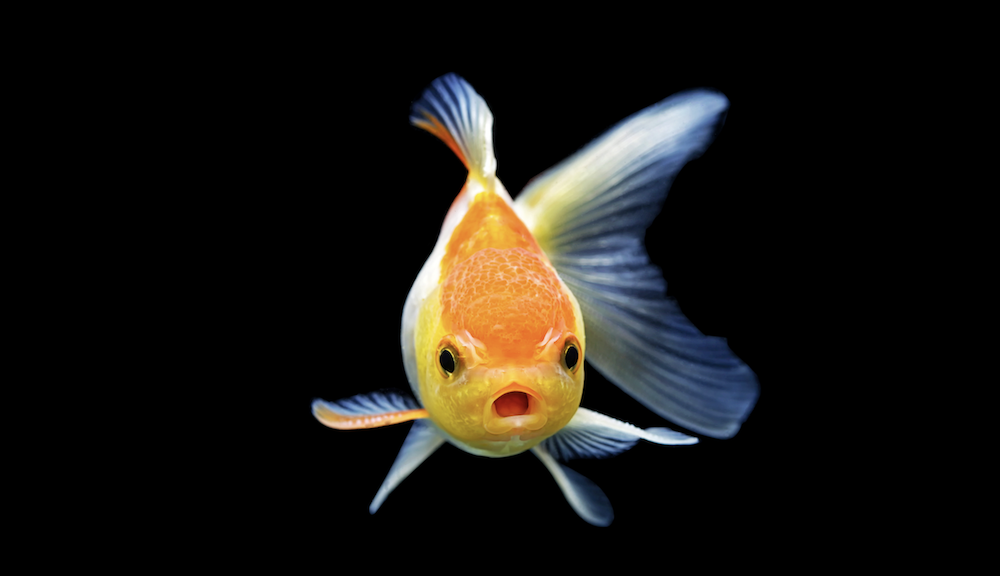
- We have long known that other mammals can count, as can some higher vertebrates such as reptiles, birds and mammals.
- Studies involving small shoaling fish, such as guppies, sought to judge whether fish can count. The ability to count indeed appears to be hardwired into the brains of fish from birth.
- It may turn out that the genes that make an individual fish good or bad at numbers are the same genes that predispose our mathematical skills.
Excerpted from Can Fish Count?: What Animals Reveal About Our Uniquely Mathematical Minds by Brian Butterworth. Copyright © 2022. Available from Basic Books, an imprint of Hachette Book Group, Inc.
Studies have shown that our near and distant mammal relatives have good or very good counting abilities. It is also important for the safety of newly hatched chicks and other birds to be able to count and to carry out calculations. Amphibians and reptiles, too, count when foraging or when choosing a mate. Apart from cetaceans with their enormous and complex brains, these are all land-dwelling creatures. Here we consider whether fish, whose environment is so different from our own, can count, and why they might need to.
Given the relatively smaller brains of fish, one might have expected that they have poorer cognitive abilities in general than ‘higher’ vertebrates – reptiles, birds, and mammals. Actually, some have better memory abilities both in the wild and in the laboratory. Salmon, for example, can remember the properties of the river in which they spawned for several years and successfully return for mating. The route through a maze can be remembered three months later.
It’s been known for many decades that joining a group, a shoal or school, can be beneficial. It will be easier to find a mate in a group. Species that feed on large particles improve their chances of finding them with many eyes looking; and being in a large group reduces the risk of an individual being eaten by a predator. The larger the shoal, the better it will be for reproduction, feeding and safety. Therefore, it would advantageous for fish to be able choose the larger shoal.
One of the earliest demonstrations of the possible use of numerical information in shoal choice was carried out with minnows (Pimephales promelas), sometimes in the presence of a predator, the largemouth bass (Micropterus salmoides). Each minnow was offered a choice of two shoals on opposite sides of the tank. The shoals ranged from one to twenty-eight minnows, and the test minnows chose the larger shoal right across the number range, with or without the presence of the predator. This suggests that joining the larger shoal is instinctive, but depends on the ability to assess the numerosities of the two shoals. Now, although numerosities were manipulated by the experimenters, it wasn’t clear that the test fish were responding to the numbers or to shoal density, since the different-sized shoals occupied the same tank volume.
Our friend the three-spined stickleback, when not in the mating season, also shoals. Like many other shoaling fish, it does respond to the density of the shoal when making a choice, since numerosity and density typically go together in real life. With the same numerosity of the two shoals, the stickleback will prefer the denser, but with the same density, it will prefer the more numerous. The standard set-up for recent studies of spontaneous – no training – numerical discrimination is shown in Figure 1.
Studies of many species of shoaling fish have shown that fish choose the bigger shoal, and it’s easy to vary experimentally the numbers on each side, so that one can calibrate the ability to estimate or compare numerosities.
Here’s one study that I did with my friends at Padua University using the apparatus shown in Figure 1. The subjects in our experiment were guppies (Poecilia reticula). We had a particular objective in this study to see if these small fish had two numerosity recognition systems, as it was claimed for other vertebrates including us humans: one small numerosity system and one for larger numerosities. As I noted in other chapters, the small numerosity system, sometimes called the ‘subitizing’ system for numerosities, has two interesting characteristics. First, it is virtually error-free, and in humans, very fast. Second, when comparing two numerosities there is no ratio effect. That is, it is just as easy to select the larger set with four objects compared with three objects, as compared with one object. For numerosities greater than four, the ratio effect kicks in, so that comparing nine with five objects is more accurate and faster than comparing nine with eight objects.
We first tested this hypothesis with Italian students. We didn’t, of course, immerse them in tanks of water, nor ask them to compare shoals of fish, but to select the larger of two arrays of dots presented successively. We measured their accuracy and the speed of these judgements.
We found what many other studies have reported, namely that for small numerosities there was no effect of the ratio between the two arrays on either accuracy or speed, while for larger numerosities there was a ratio effect for both of these measures. In humans, the brain processes large and small numerosities differently.
Are these two systems present in the guppy brain?
It turns out that they are. What is more, the two systems are present at birth. We tested 100 one-day-old fish and 140 ‘experienced subjects’. Here are the numerosities and the ratios we used:

The day-old fish performed identically to the adults. This suggests that the two systems are wired in and start functioning without the benefit of experience.
A variant invented by my friends at Padua University allows the test fish to see only one fish at a time. The Padua team used mosquitofish (Gambusia holbrooki ), a small freshwater shoaling fish. The baffles in the tank meant that the test fish could swim about freely, but could see only one fish at a time. These little fish were able to select the larger shoal when the contrast was in the small number range (3 vs 2) and also in the large number range (4 vs 8). This means the test fish had to sum the number of fish on each side of the tank, remember the sums, and carry out a numerical comparison between the two shoals in order to choose the larger shoal.
This numerical ability is importantly adaptive because it enables fish in the wild to choose the safety of the largest available shoal. Here, individual differences play a role. The fish with the best numerical ability will lead other fish, and perhaps their leadership role ensures that the shoal stays together by heading in the same direction. It may turn out that the genes that make an individual fish good or bad at numbers are the same genes that predispose us in the same way.