The truth about wormholes and quantum computers
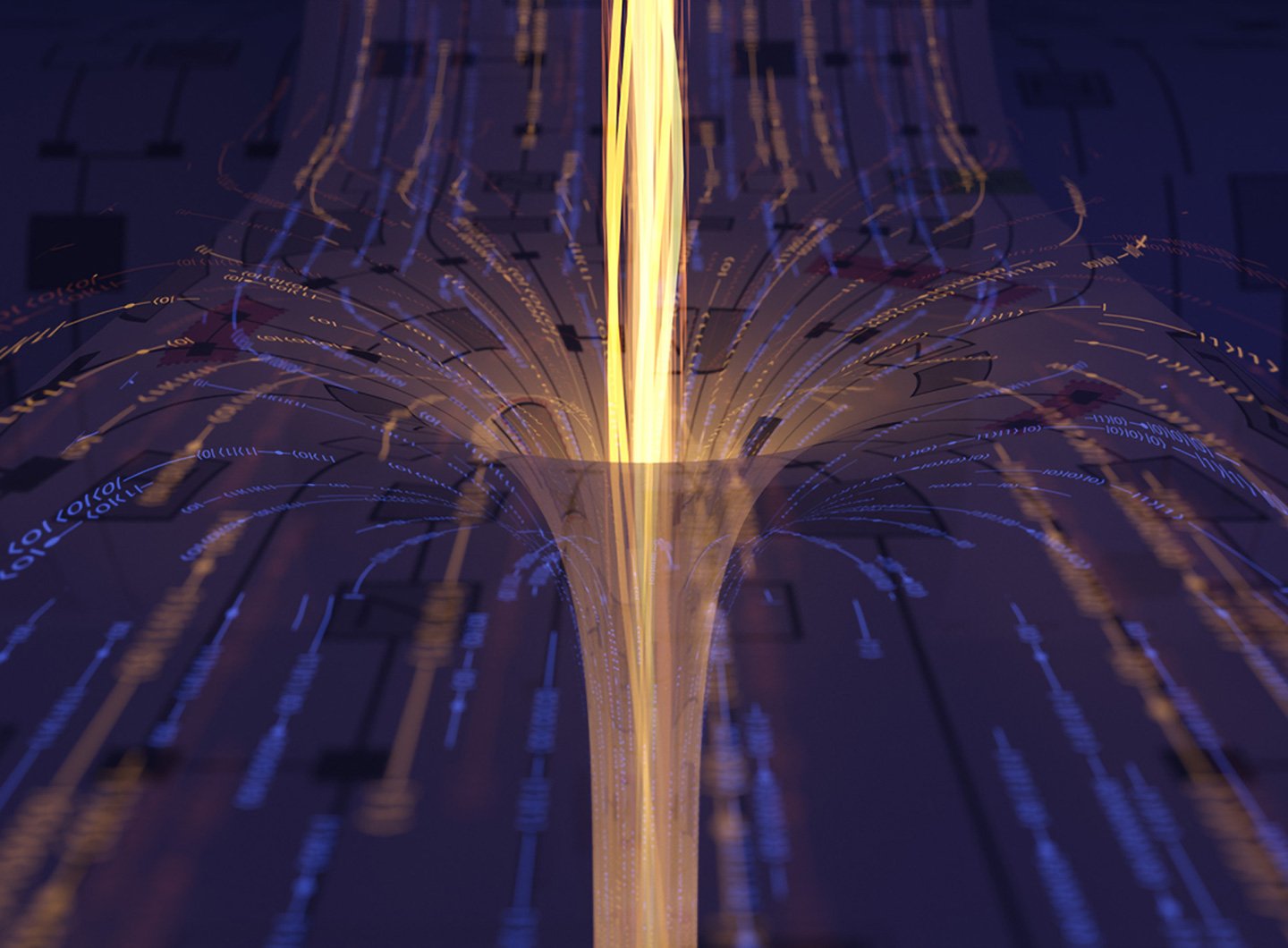
- The notion of a wormhole suggests that two well-separated regions of space could be connected through a bridge, enabling instantaneous travel of information or even possibly matter from one location to another.
- Whether this is possible in our Universe or not depends on the existence and stability of negative mass/energy in the context of our theory of gravitation: General Relativity.
- Something interesting may have been recently simulated on a quantum computer, but is there actually a connection to wormholes? Get the actual truth instead of the hype.
There should be one question you ask yourself anytime you encounter a claim that can be answered by science, “What is true?” Only by looking at the answer to that question — and, in particular, what can be and has been established to be scientifically true by the full suite of available evidence — can you draw a responsible conclusion. If we look at anything else, including what we hope, what we fear, or what unsupported speculations can’t be ruled out, we’re practically guaranteed to lead ourselves astray. After all, if the evidence isn’t enough to convince those with expert knowledge, it should be insufficient for the rest of us as well.
On November 30, 2022, a paper was published in Nature that claimed that a wormhole was simulated on a quantum computer, claiming that the observed features could be linked to real, traversable wormholes that could exist within our own Universe. There are three parts to this story:
- the physics of wormholes within General Relativity,
- the actual simulation conducted on a quantum computer,
- and the link between our real Universe and the quantum computation,
and we have to get all three parts correct if we want to separate what’s true from the speculative, unsupported claims that many — including some of the study’s authors — have been publicly making. Let’s dive into all three.
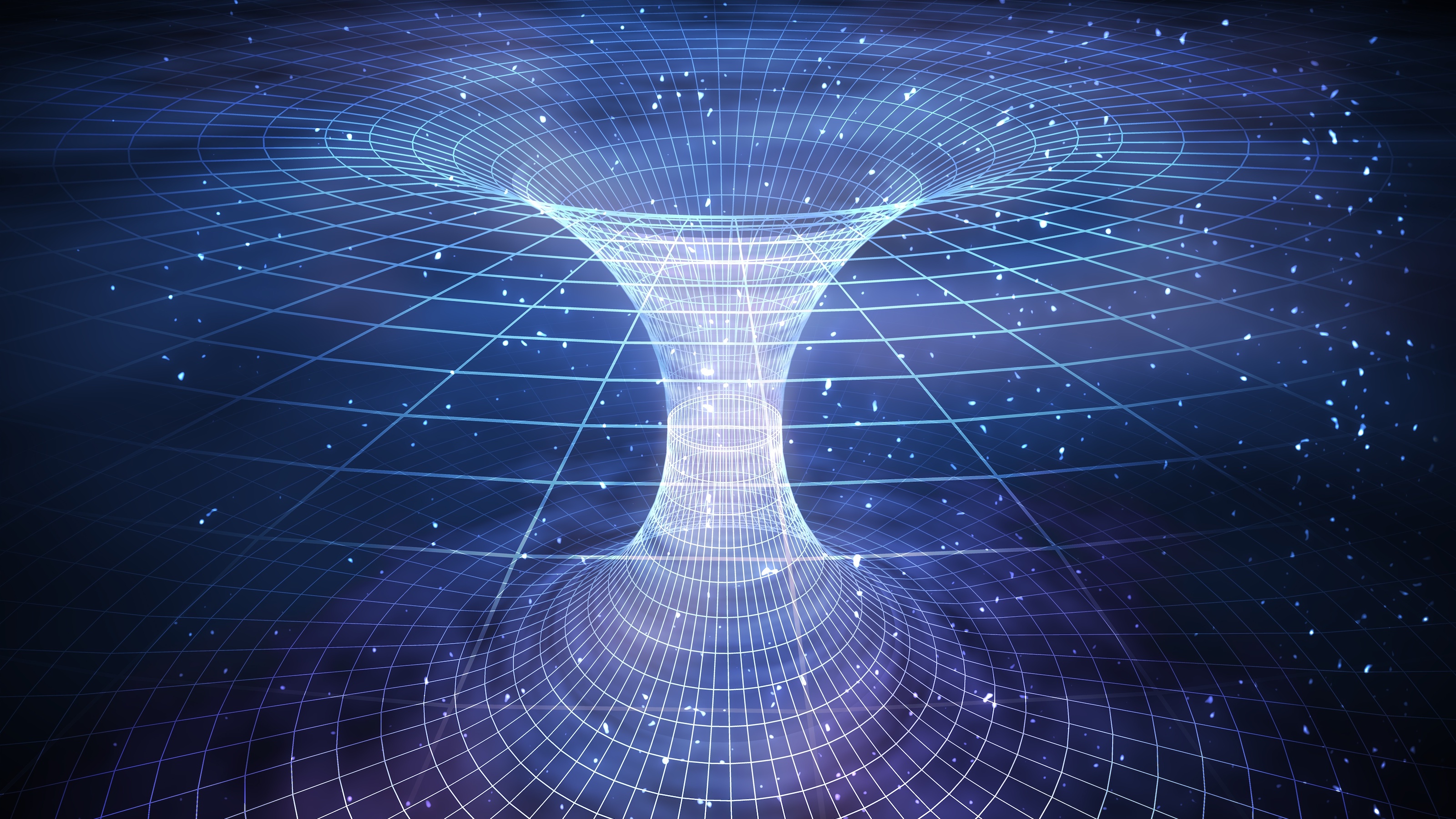
The physics of wormholes
The idea of a wormhole was born very shortly after the discovery of the first exact, non-trivial solution in General Relativity: the Schwarzschild solution, corresponding to a non-rotating black hole. To obtain this solution, all you have to do is take completely flat, empty space and place down one object of infinitesimal volume, but finite mass. Wherever you place that down, you’ll have a black hole of a certain mass, surrounded by an event horizon of a specific radius determined by that mass. Einstein finished formulating General Relativity toward the end of the year in 1915, and in early 1916, Karl Schwarzschild published this early, remarkable solution that’s still relevant and widely-used today.
It was realized by a number of people — independently of one another — that if you were able to connect a Schwarzschild black hole (with a positive mass) at one location in the Universe to its negative mass/energy counterpart at another location, you could theoretically “bridge” those two locations. That bridge, in modern parlance, is now known as a wormhole. Originally, this theoretical solution was found by Flamm in 1916, then again by Weyl in 1928, and most famously once more by Einstein and Nathan Rosen in 1935.
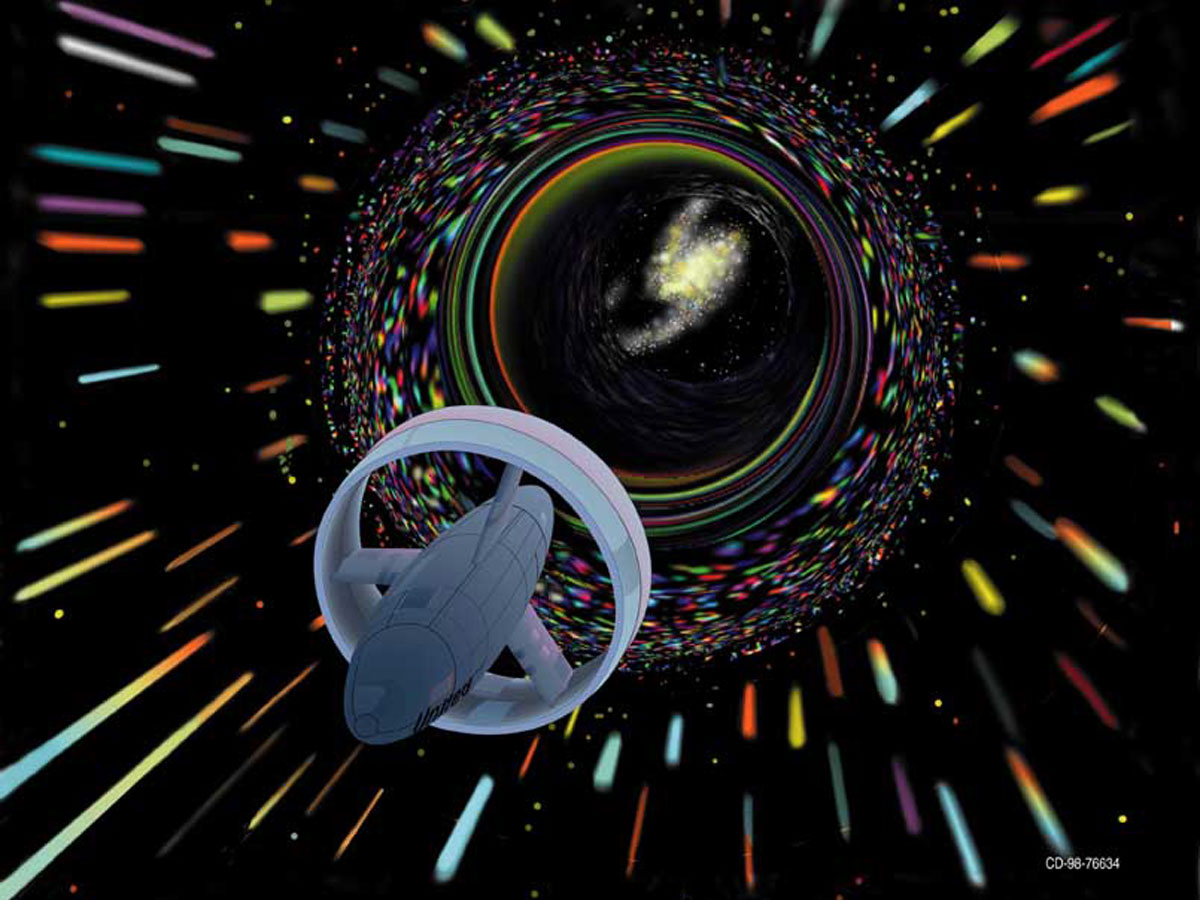
Also known as Einstein-Rosen bridges, this early theoretical work paved the way for our modern understanding of wormholes within the context of General Relativity. While these early wormholes had a pathology to them in the sense that they would rip apart and destroy any matter that dared enter them, there have been a number of extensions that have been proposed to help “hold these wormholes open” as matter attempted to pass through it. We generally refer to this species of wormhole as a traversable wormhole, and most of the wormholes we encounter in science fiction are precisely of this flavor.
Whether or not wormholes can physically exist or not is a question that’s still hotly debated. Yes, we can mathematically write down solutions to Einstein’s equations that include them, but mathematics is not the same as physics. Mathematics tells you what’s within the realm of physical possibility, but only the actual, real Universe itself is going to reveal to you what’s physically true. The places we’d look for such physical evidence have all come up empty so far.
- We’ve observed real black holes; there are no signals from them suggesting they’re wormholes.
- We’ve observed lots of systems with positive energy; there are no systems with intrinsically negative energy.
- And we’ve observed lots of systems that possess three or fewer spatial dimensions; there’s yet to be a shred of evidence for a fourth (or higher) spatial dimension.
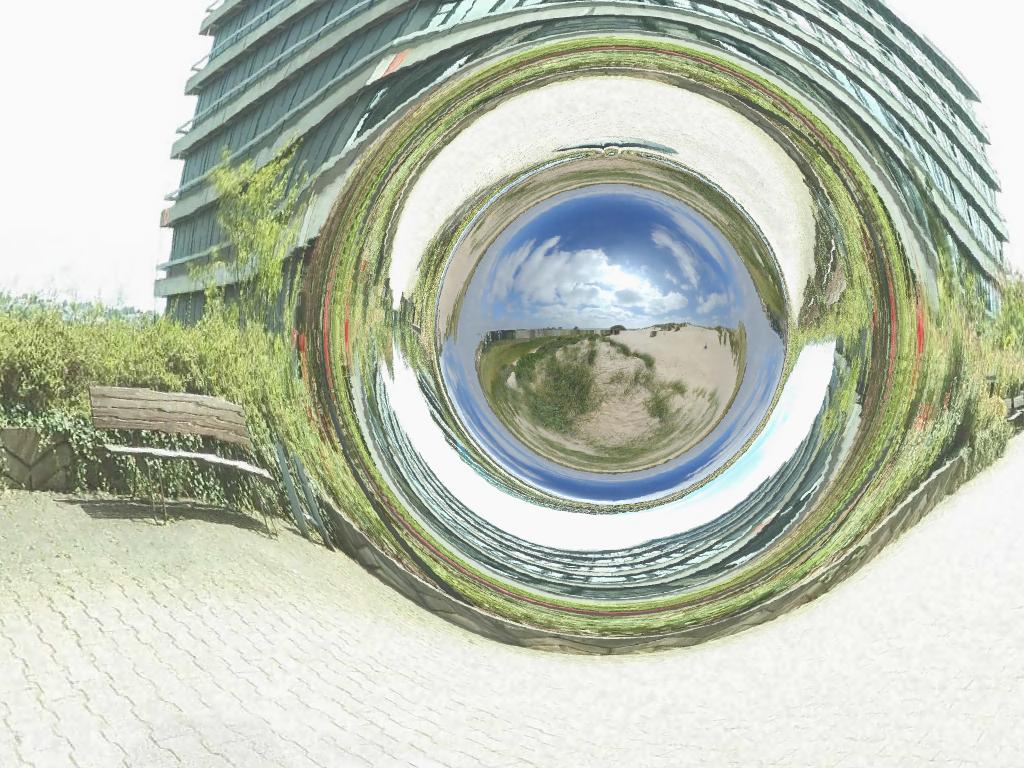
The big dealbreaker for our Universe, as far as we know today, seems to be the lack of what one might call “exotic” matter. The simplest way of looking at the situation is to think of space as having an average energy density from all sources: matter, radiation, and even the (positive, non-zero) zero point energy of empty space itself. Where you have positive energy, space curves in response to that; this is why massive particles exhibit the phenomenon of gravitational attraction. So far, all we’ve ever detected in the Universe is matter-and-energy with positive values to it.
But if you want to have a traversable wormhole, you need some type of matter and/or energy that has a negative value to it, at least negative relative to the average energy density of the Universe. Although we can create small regions of space that have this property — e.g., the empty space between two parallel conducting plates, such as a setup exhibiting the Casimir effect — there are no species of negative energy quanta known to exist.
If they truly don’t exist at all, extra spatial dimensions, extra fields, or some sort of Planck-scale bridge (perhaps only allowing for the transfer of information, not matter) are the only ways that wormholes could physically arise within General Relativity.
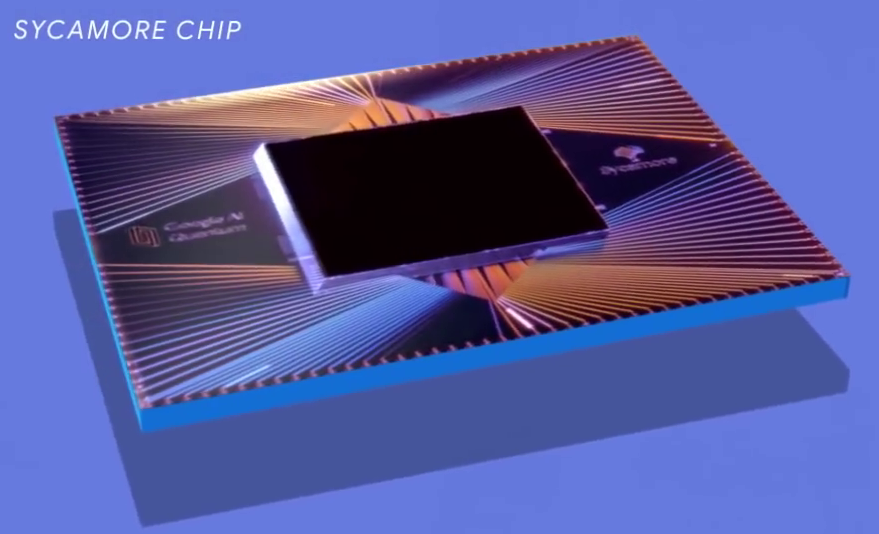
The quantum simulation
In their recent paper, what the authors created was not an actual wormhole itself, but rather a quantum circuit that possesses some analogue behaviors and properties to a gravitational wormhole. This builds on earlier work, some of which needs to be recounted in order to understand the importance of this latest work.
Previously, some members of this team had concocted a scenario where a negative-energy pulse was transmitted between two topologically connected points, and that pulse was used for the purposes of quantum teleportation: to transfer the quantum state from one “side” of the two connected points to the other.
This is an interesting application, but it’s hard to see how it’s connected to wormholes and gravity. The only suggestion of a connection — and it’s important to emphasize that it’s only a suggestion — is that in 2013, Juan Maldacena and Leonard Susskind conjectured that a wormhole, or an Einstein-Rosen bridge, is equivalent to a pair of maximally entangled black holes. This connection is sometimes referred to as ER = EPR, to note that a wormhole (or Einstein-Rosen bridge) is connected to quantum entanglement, as the first paper on entanglement was authored by EPR: Einstein, Boris Podolsky, and Rosen.
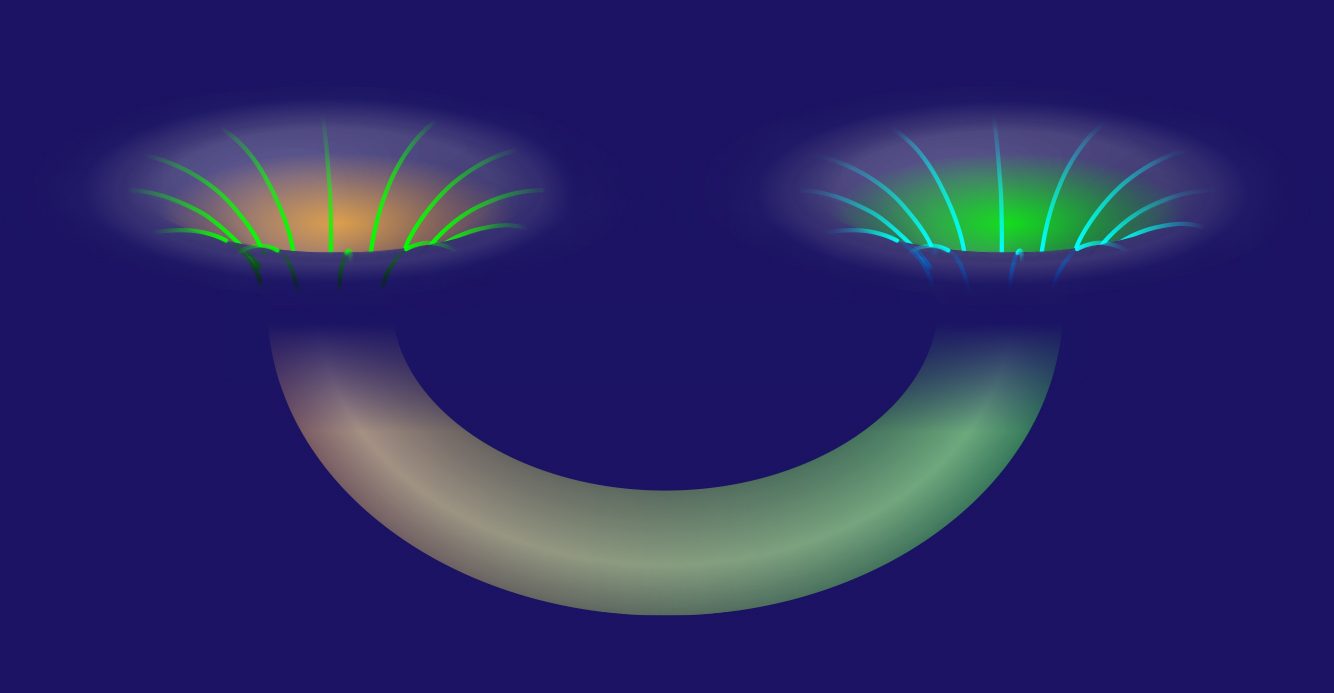
We know that the full physical system is too difficult and complex to simulate with any sort of robust accuracy, so the authors did what practically all theoretical physicists do: they modeled a simpler approximation of the full problem, with the idea being that by simulating the simple approximation, many of the key properties of what would be a “true wormhole” would still persist. Partially because of the limitations of what we can actually simulate with current technology, and partially because of how limited human beings are in terms of the quality of models we can create, machine learning was used to design the experimental setup. According to Caltech’s Maria Spiropulu, coauthor of this paper:
“We employed learning techniques to find and prepare a simple [analogue] quantum system that could be encoded in the current quantum architectures and that would preserve the [needed] properties… we simplified the microscopic description of the [analogue] quantum system and studied the resulting effective model that we found on the quantum processor.”
The experiment showed that, once again, just as in the earlier experiment, quantum information traveled from one quantum system to the other: another example of quantum teleportation.
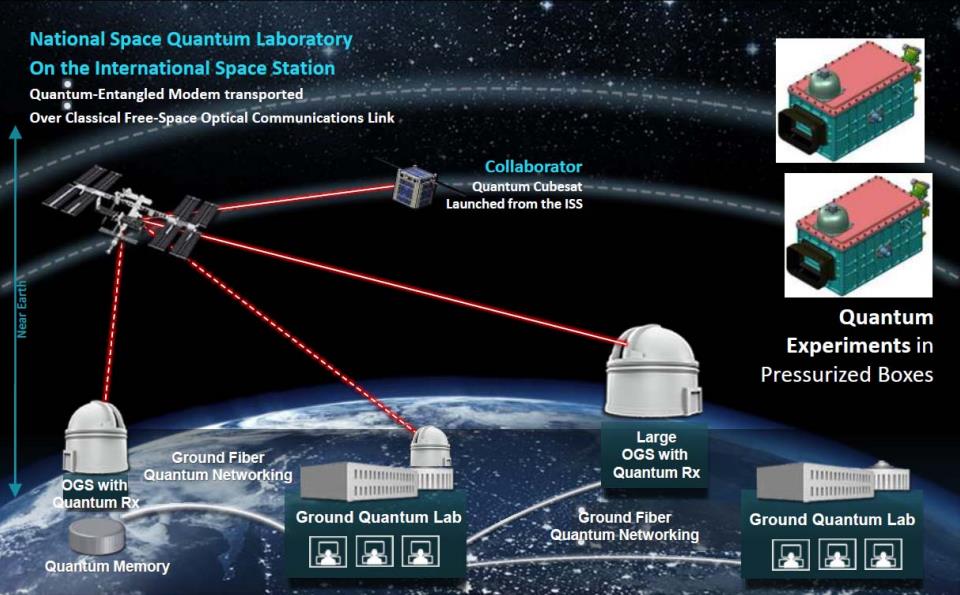
The link between the real Universe and this “quantum wormhole” simulation
Why should we care about this work, and what, if anything, does it teach us about the connection between wormholes and the types of simulations that a quantum computer can do?
The normally-sober Quanta magazine gave an accurate, in-depth account of the simulation performed on the quantum computer, but missed the boat entirely on this front, as many others were quick to correctly point out.
First off, the use of a quantum computer taught us nothing that we couldn’t learn (and didn’t already know in advance!) from using classical computers and hand calculations. In fact, the only novel thing that was accomplished by this team of researchers — a mix of quantum computation specialists and theoretical physicists — was that they were able to use machine learning to successfully simplify a previously complex problem into one that could be simulated using just a small number of qubits on a quantum computer. That’s an impressive technical achievement, and one that deserves to be celebrated for what it is.
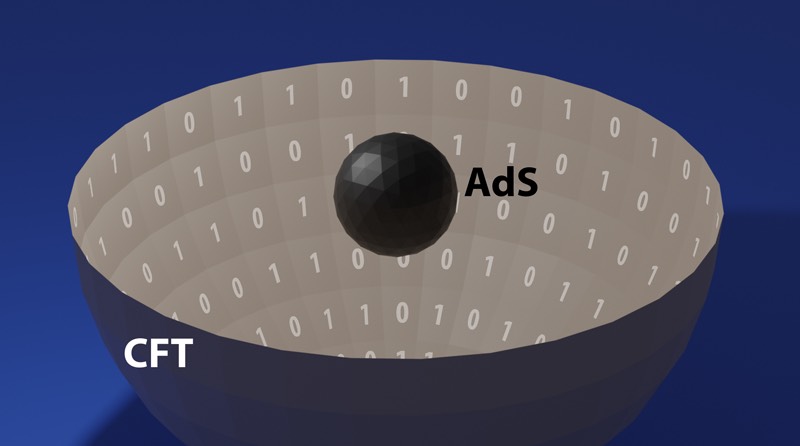
But instead, many are celebrating this achievement for what it isn’t: evidence that wormholes have any relevance to our physical Universe, and/or evidence that this quantum simulation provides a window into how wormholes would actually behave in our Universe.
Here are some true things that you should know about what the newly-touted research actually did (and didn’t) do.
It did only use 9 qubits in their simulation. 9 qubits means that the quantum wavefunction encoded could at most require 512 (because 29 = 512) complex numbers to describe it, which is a simple enough wavefunction that it could be easily simulated on a classical computer. In fact, it was simulated on a classical computer by these very researchers in advance of the simulation they performed on their quantum computer! (With identical results to the limits of the quantum errors that arise from quantum computation processes in 2022.)
In other words, there was nothing that was learned from performing this simulation on a quantum computer other than the behaviors that they were expecting to see persisted even in this simple, 9 qubit simulation. Although this bodes well for future simulations along the same lines, it doesn’t provide any profound, fundamental insights beyond showing some potential for quantum computers.
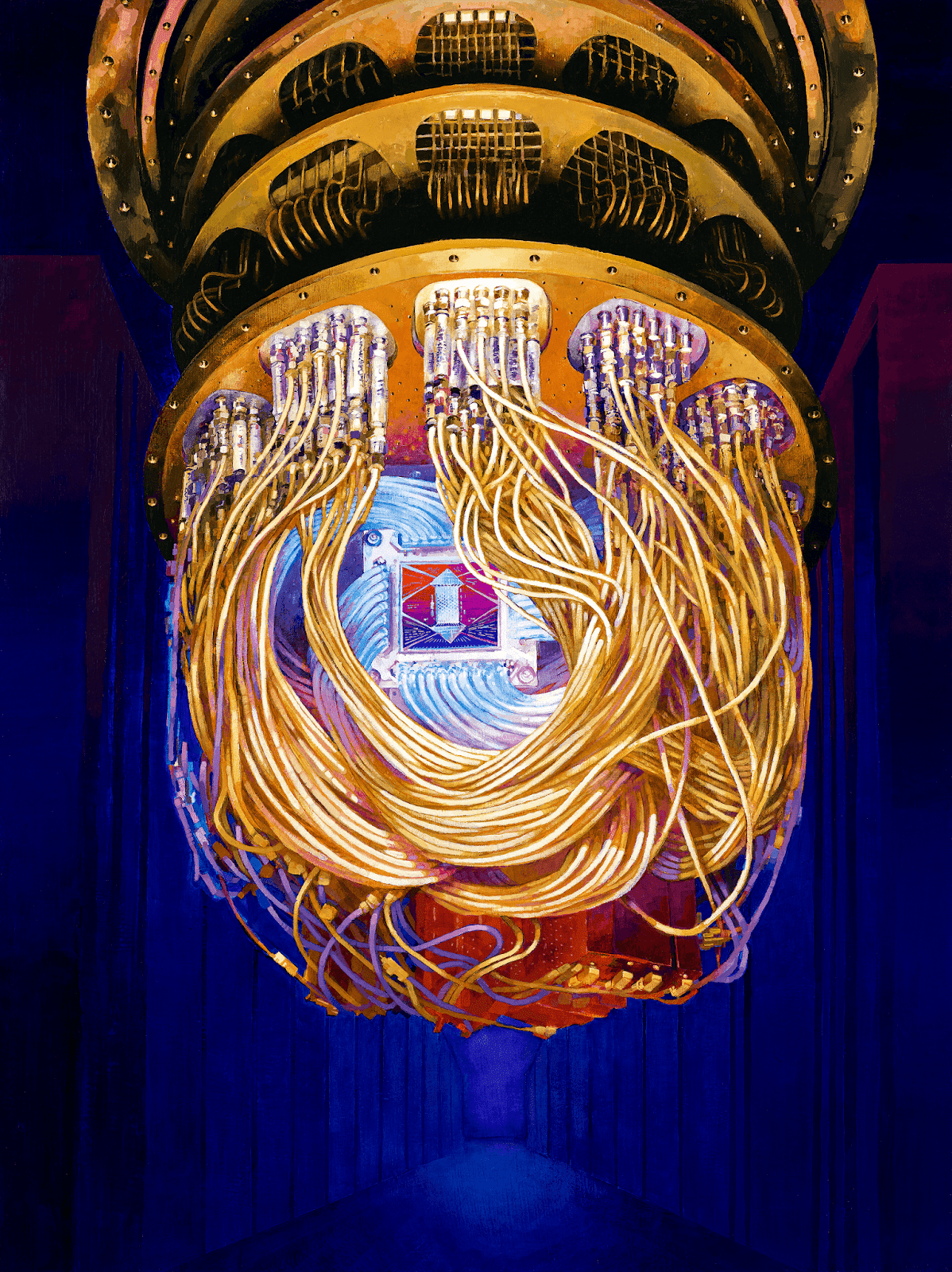
So what about the connection to wormholes? You know, gravity-based wormholes within General Relativity that might actually apply to our real, physical Universe?
It’s about as speculative as it can get. First, it assumes that the holographic principle — which states that all physical properties within a volume of space can be encoded on a lower-dimensional boundary of that space — is, in fact, a property of the yet-undiscovered quantum theory of gravity. Second, instead of using the AdS/CFT correspondence, which is the established mathematical equivalence between a 5D anti-de Sitter space and the 4D conformal field theory that defines the boundary of that space, they use the suggestive correspondence between the Sachdev-Ye-Kitaev model and a two-dimensional anti-de Sitter space.
That’s a mouthful, but what that means is that they model gravity in “our Universe” as having one time dimension, one spatial dimension, and a negative cosmological constant, and then take what might be a mathematically equivalent description (the Sachdev-Ye-Kitaev model) and simulated that instead. Some of the properties they observed were analogous with some of the behaviors a traversable wormhole is expected to exhibit, but this provides no insights into how a traversable wormhole in our actual Universe, governed by General Relativity (in three spatial and one time dimension with a positive cosmological constant), would behave.
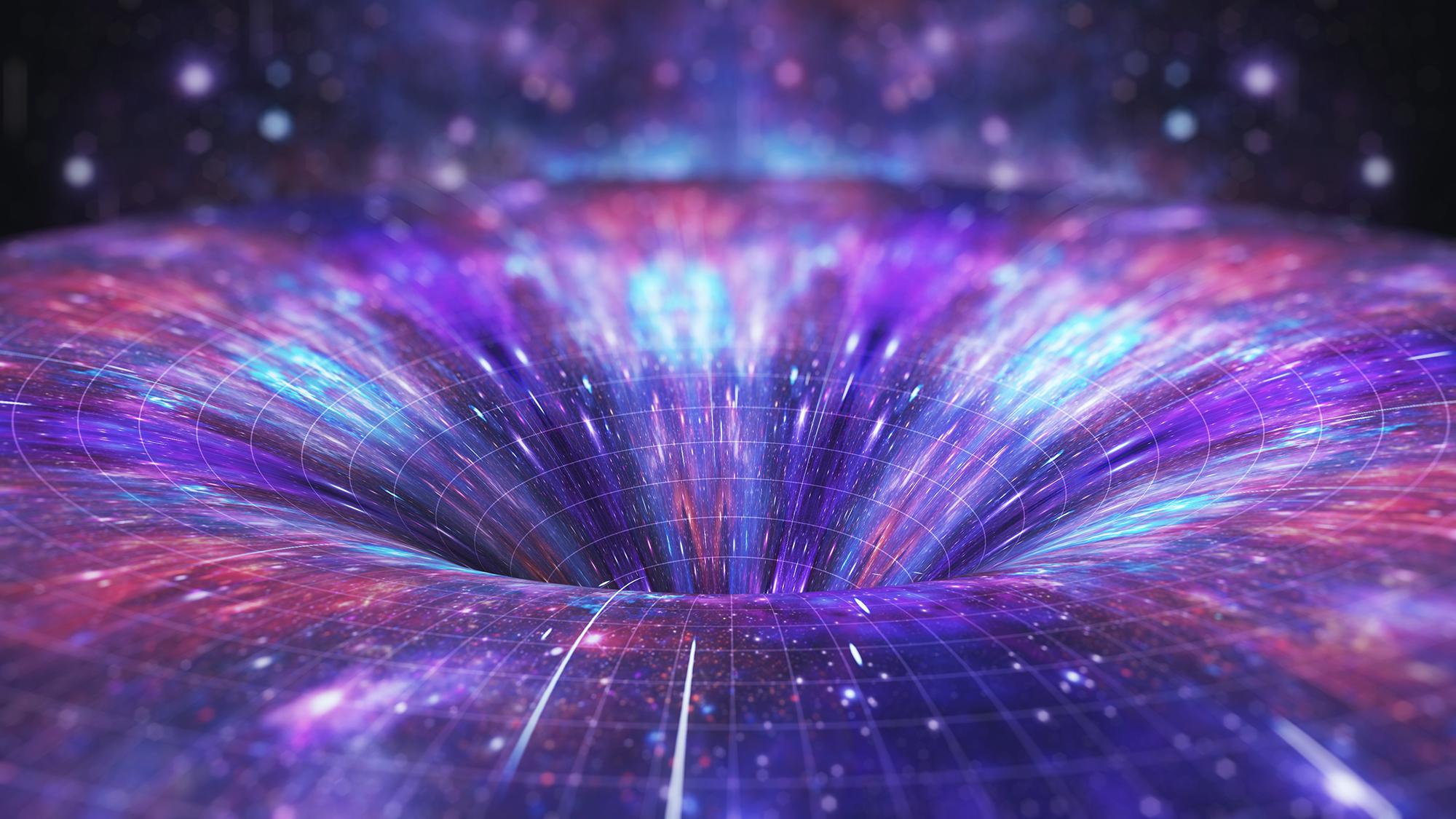
There are no lessons to be learned about quantum gravity here. There are no lessons to be learned about traversable wormholes or whether they exist within our Universe. There are not even any lessons to be learned about the uniqueness or capabilities of quantum computers, as everything that was done on the quantum computer can be done and had previously (without errors!) been done on a classical computer. The best that one can take away is that the researchers, after performing elaborate calculations of the Sachdev-Ye-Kitaev model through classical means, were able to perform an analogous calculation on a quantum computer that actually returned signal, not simply quantum noise.
But it’s time to get real. If you want to study something relevant for our Universe, then use a framework that our Universe is actually analogous to. If you’re only making an analogue system, be honest about the limitations of the analogue and the system; don’t pretend it’s the same as the thing you’re oversimplifying. And don’t lead people down the path of wishful thinking; this research will never lead to the creation of a real wormhole, nor does it suggest “wormholes exist” any more than spin-ice experiments suggest “magnetic monopoles exist.”
Wormholes and quantum computers will likely both remain topics that are incredibly interesting to physicists, and further research into the Sachdev-Ye-Kitaev model will likely continue. But the connection between wormholes and quantum computers is virtually non-existent, and this research — despite the hype — changes absolutely nothing about that fact.