Winning a Nobel Prize from 2 km under the Earth
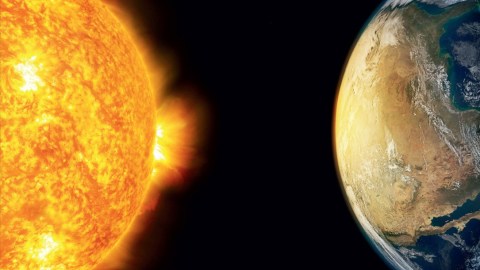
A live blog event from Nobel Prizewinner Art McDonald.
“It’s ironic: in order to observe the Sun, you need to go kilometers underground.” –Art McDonald
In the 1960s, a huge mystery began to unfold: the signals we were seeing from the Sun were only a third as strong as they needed to be to explain its energy output. We had known for some time that nuclear fusion was the process powering the Sun, and that at the incredible temperatures, pressures and densities at the Sun’s core, hydrogen nuclei were fused together in a chain reaction to eventually produce helium, releasing huge quantities of energy in the process. This was fundamentally powered by Einstein’s most famous equation, E = mc^2, where matter gets converted into pure energy, since helium nuclei are about 0.7% lighter than the four hydrogen atoms each one is created from. Yet there should be a by-product of that nuclear reaction we could detect on Earth: the emission of neutrinos.
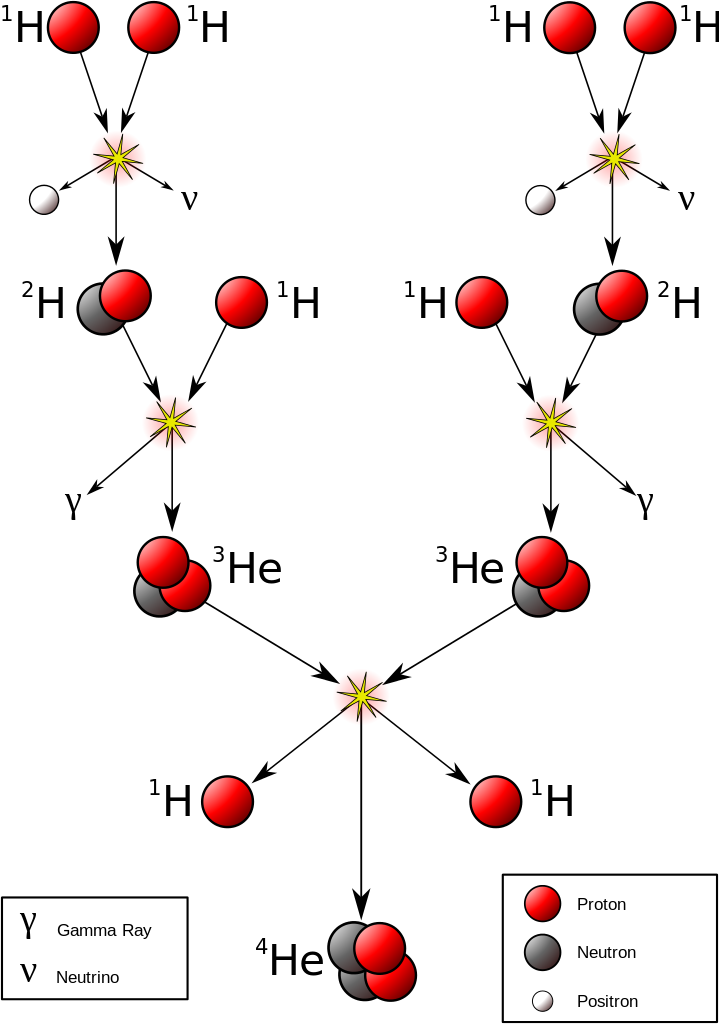
Specifically, it should have been electron neutrinos that were created, one of the three “flavors” that neutrinos come in. Yet when we made our greatest models of the Sun, calculated the overall energy from it and measured the neutrinos that arrived on Earth, there was a huge gap: we were seeing only a third of the neutrinos we predicted. For decades, people argued over whether the calculations were wrong, whether the models of the Sun were wrong, or whether our understanding of neutrinos were fundamentally wrong. We had assumed — as the Standard Model predicts — that neutrinos were massless, and hence should travel from the Sun’s core to Earth unperturbed.
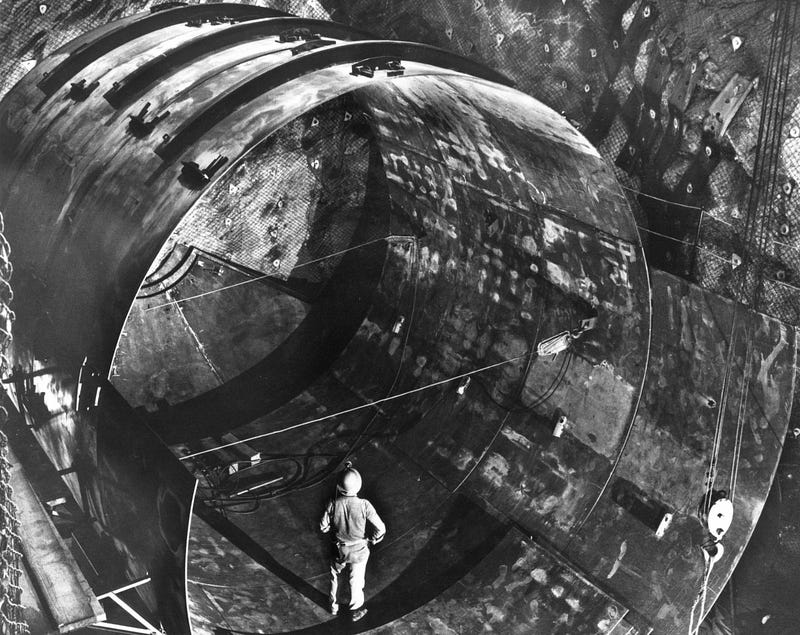
Yet as our experiments and our models got better and better, the same problem remained: only a third of the predicted neutrinos were arriving! One possible explanation, exotic though it seemed, could have accounted for it: perhaps the neutrinos weren’t massless at all, but had tiny, tiny masses that were over a million times lower than the electron, the next lightest particle. If they weren’t exactly massless, then when they traveled through space and, more importantly, through matter in space, they could oscillate from one species — electron, muon and tau — into another.
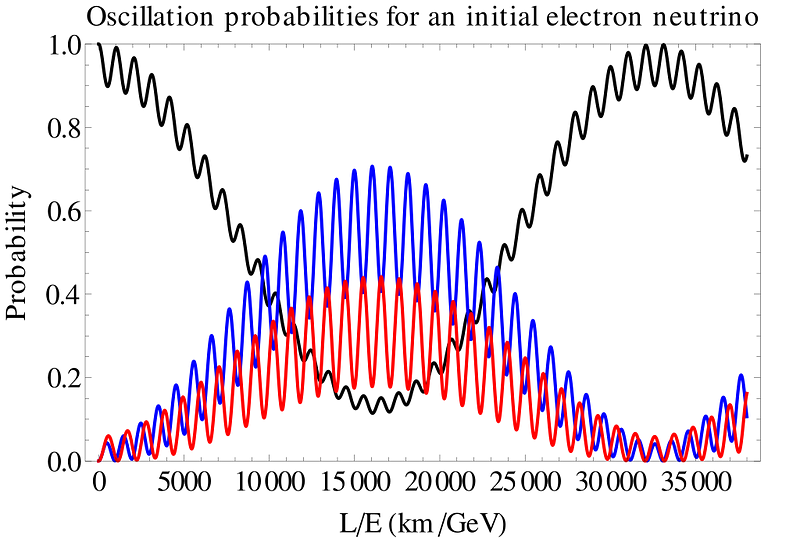
In other words, the neutrinos were being created exactly as predicted, but were “disappearing” because our detectors, at the end, were only sensitive to electron neutrinos, not the two other types! These limitations all changed with the advent of new neutrino detectors, such as the Sudbury Neutrino Observatory (among others), which allowed us to finally directly detect the missing neutrinos and confirm the neutrino oscillation picture.
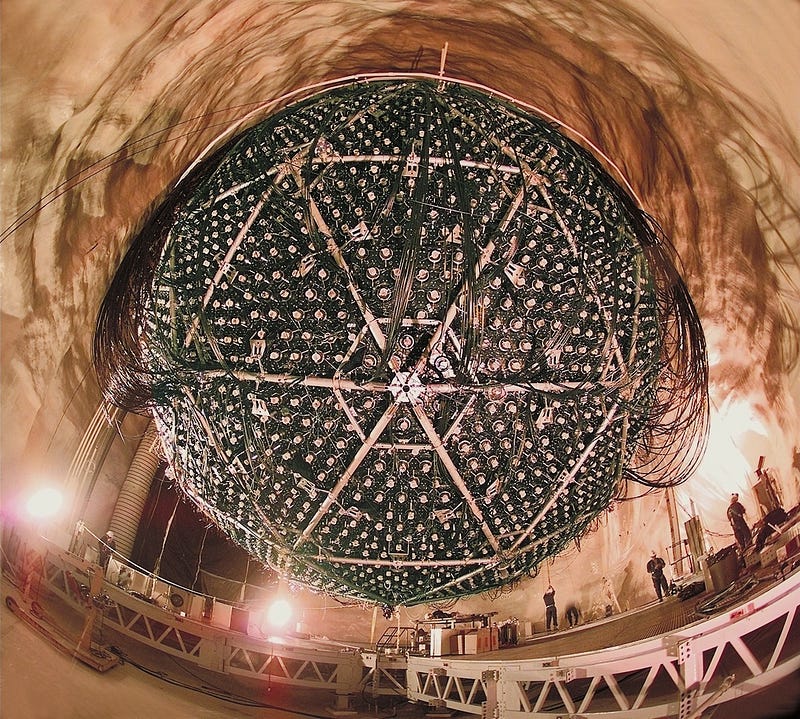
For his work on the Sudbury Neutrino Observatory, scientist Art McDonald was awarded the Nobel Prize in Physics in 2015. And not only that, but he’s giving today’s Perimeter Institute public lecture on his work, the discovery neutrino oscillations, and the future of neutrino physics.
What’s most amazing about this is that this is the first concrete, indisputable evidence that there is physics beyond the Standard Model — not even including gravity — that exists in our Universe. The possibilities include extra, heavy (Dirac) neutrinos, fourth (sterile) neutrinos, both of which are conceivably dark matter candidates. It’s even possible that the neutrino is its own (Majorana) antiparticle! Tune in at 7 PM ET / 4 PM PT (or any time after), below, andcatch Art McDonald’s public lecture, accompanied by my unique, professional-physicist live blog of the event.
https://www.youtube.com/watch?v=SrPLtIs4Dyg
See you soon!
Update, 3:47 PM #piLIVE Twitter TWTR +2.84% @startswithabang
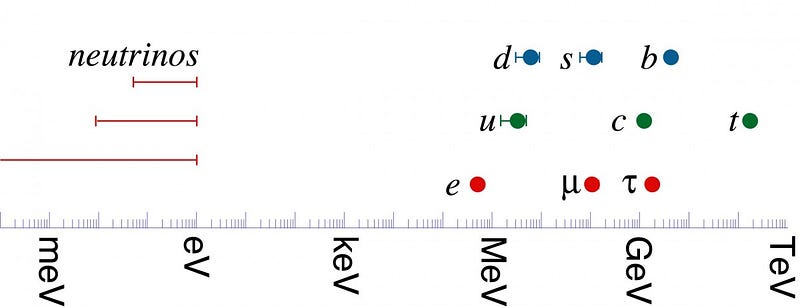
3:51 PM: How much lighter are neutrinos than all other particles? Limited to be ~0.1 eV at most for the heaviest one, compared to 511,000 eV for the electron, the next-lightest particle with a non-zero mass.
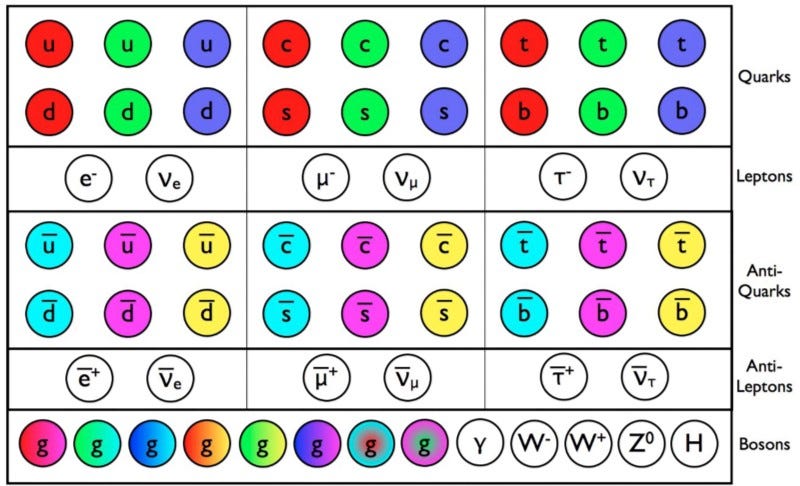
3:55 PM: See this? All the known particles in the Standard Model? Because neutrinos have mass, there has to be something else in the neutrino sector that isn’t part of this model. A fourth (sterile) neutrino? Super-heavy (Dirac) neutrinos? That neutrinos are maybe their own antiparticles (Majorana particles)? The quest continues!
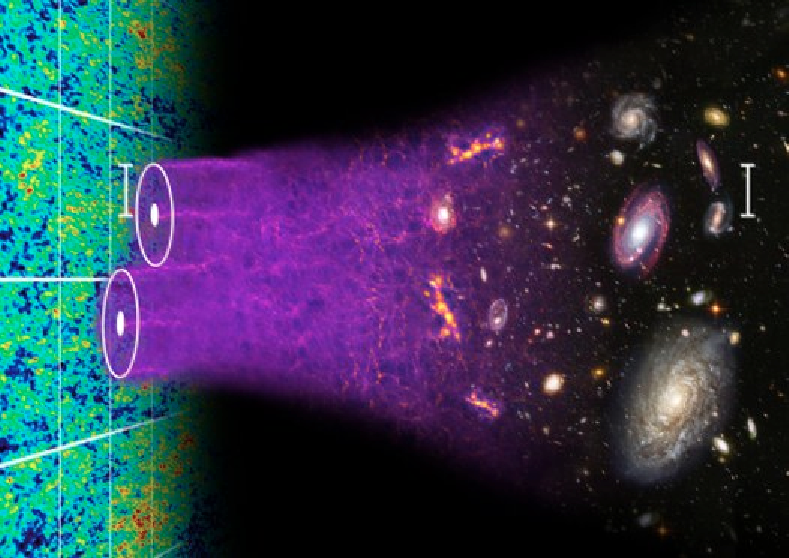
3:58 PM: Okay, last fun fact before the talk starts: the way we measure the masses of neutrinos, the best, is cosmologically. Their imprint in the Cosmic Microwave Background: the leftover glow from the Big Bang. The fact that they contribute at such a small level tells us their combined masses (of all three species) is less than 0.2 eV/c^2, total, while direct measurements of the electron neutrino from tritium (beta) decay is more than ten times worse. The oscillation measurements only tells us mass differences, not absolute masses of the neutrinos. For that, we need something extra, and cosmology is the best “extra” thing we have!
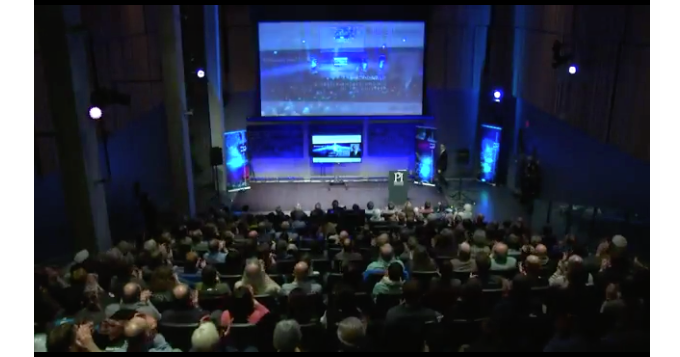
4:01 PM: Wow, what a packed house!
4:02 PM: How do you find out more about Sudbury Neutrino Observatory and the science it’s continued — and continuing — to do? Follow them on Twitter at @SNOLABscience!
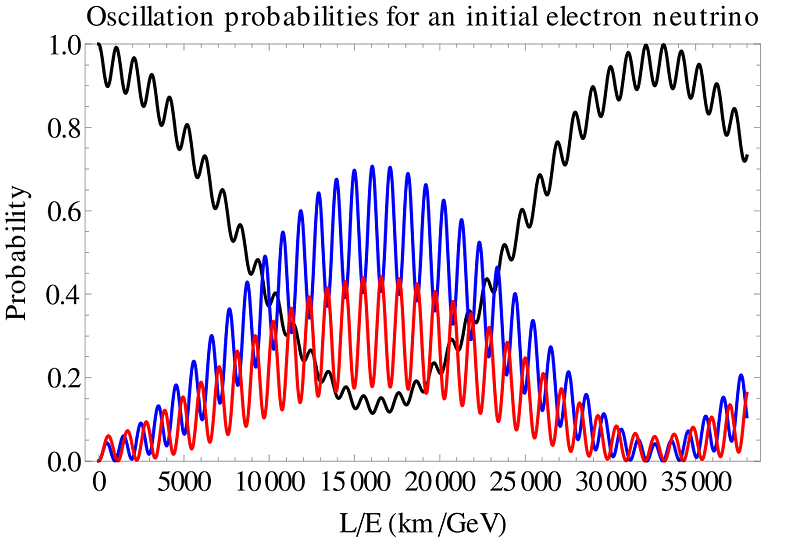
4:05 PM: First question for me from Rob Krol on Twitter — how fast are neutrino oscillations? — it actually depends on whether you are in vacuum (which are slower) or matter (which is faster), what the density is, and what the mass differences and absolute masses of the neutrinos are. The mixing is determined (a little technical) by the MNS mixing matrix, but if you want a distance scale, it takes tens of thousands of kilometers for the oscillations, but less through matter.
In other words you can tell the difference between when your neutrinos are on the “day side” and the “night side” of the Earth by whether they have to go through the Earth (and oscillate extra) or not!
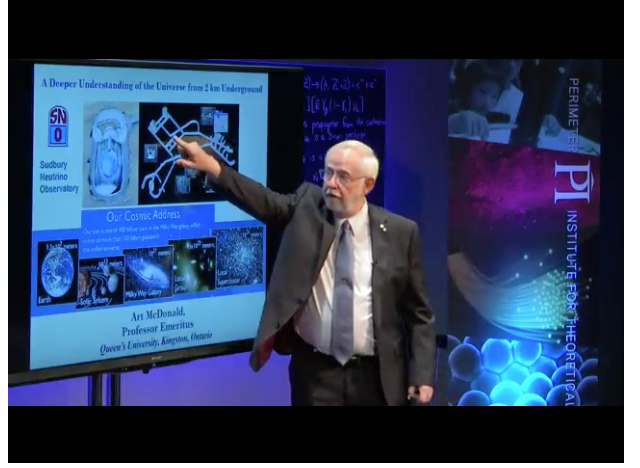
4:09 PM: Here’s what the current setup looks like. And yes, they aresearching for dark matter down there!
4:11 PM: Why be 2 km underground? Three reasons:
- Shielded from all the above-ground radiation: solar radiation, man-made, terrestrial activity, etc.
- Huge shielding against radioactivity. This is the lowest-level natural radiation background anywhere humans have gone on Earth.
- The Earth shields us from cosmic rays, including ultra-high-energy rays and muons, which penetrate large distances.
This is the best-shielded location anywhere humans have ever gone.
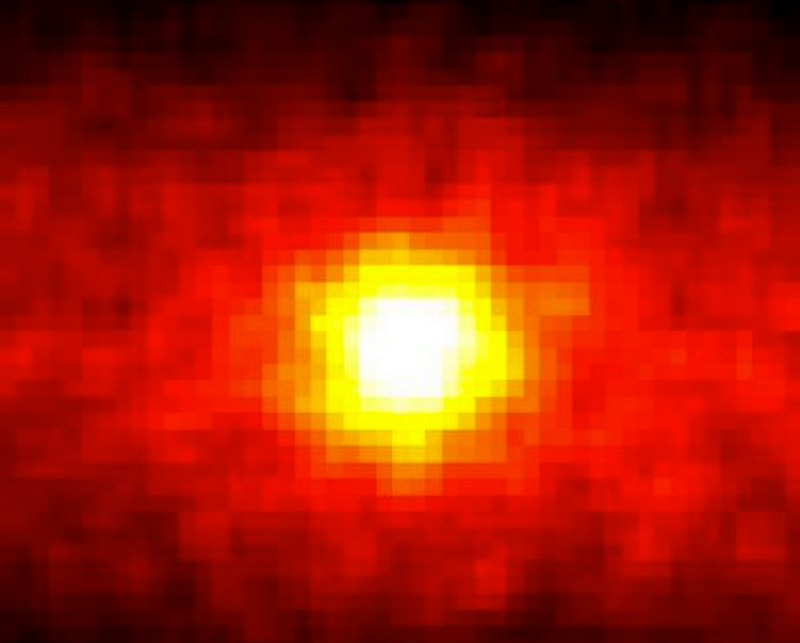
4:14 PM: Here’s a fun picture. Recognize that guy? It’s the Sun. At night. Taken from the night side of Earth, because it’s imaged in neutrinos, which travel through the Earth. In fact, they only stop if they hit the nucleus or an electron head-on, which requires about a light-year of lead to stop half of the neutrinos.
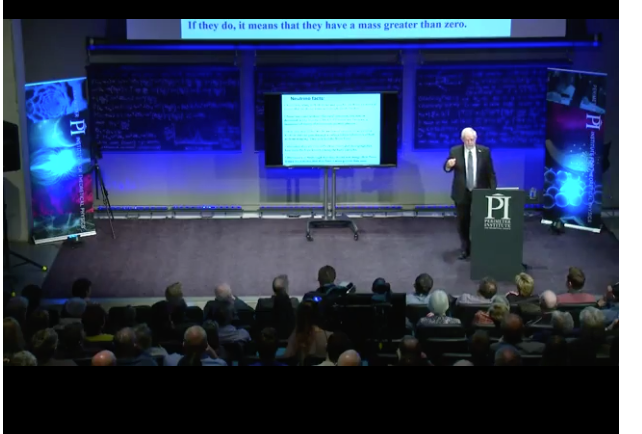
4:17 PM: Can you see the text at the bottom of his slide? “If [neutrinos oscillate], it means they have a mass greater than zero.” Not predicted by the Standard Model; this is something more. Like dark matter, dark energy, baryogenesis and more, this is new physics!
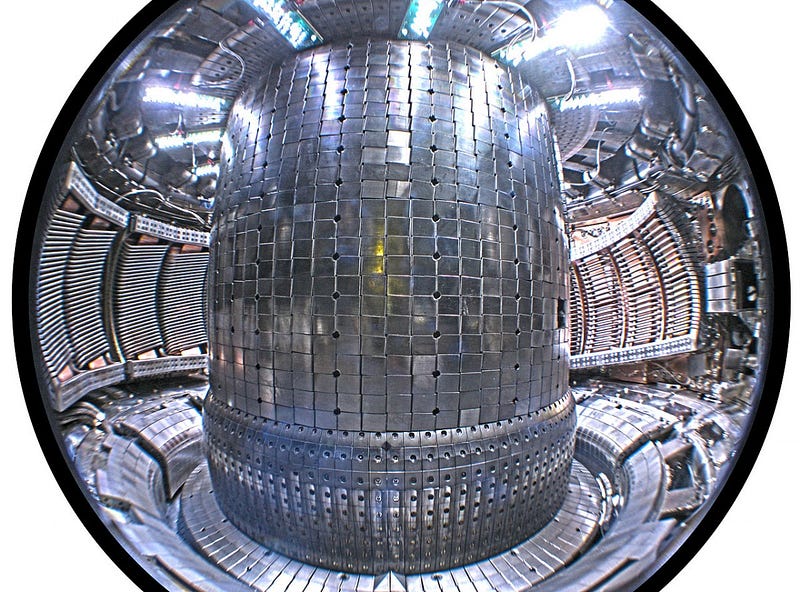
4:21 PM: Neutrinos from the core of the Sun show us the fusion process held in place by gravity, and when we try to undergo fusion on Earth, we don’t have gravity holding things together. We need magnetism (plasma fusion) or some external force (e.g., lasers, in inertial confinement), but gravity is out of the question. What we learned from neutrinos is that the fusion issues are well understood, and so confinement is the only real challenge left to solve to bring about nuclear fusion for humanity on Earth! (This is the holy grail of free energy, by the way!)
4:23 PM: Art McDonald talks about how, in the mid-1980s, either John Bahcall’s calculations about the Sun were wrong, Ray Davis’ Homestake experiments were wrong, or something like neutrino oscillations were happening. Prior to this, unfortunately, most physicists — for nearly 20 years — had heaped damnation on Ray Davis for his “lousy” experiments. As it turned out, Davis’ experiments were pretty perfect, and neutrino oscillations were real!
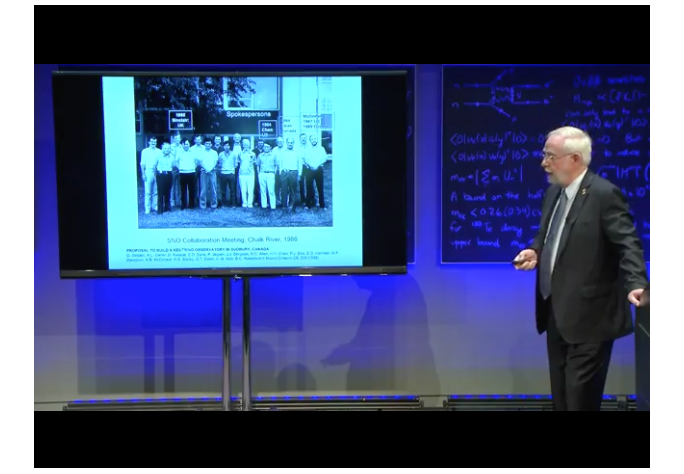
4:26 PM: The SNO collaboration started out as ~16 people, led by Herb Chen. Herb Chen took ill and died very young; Art McDonald took over as the U.S. lead for this experiment after that. If history were different, Herb Chen would’ve won the Nobel Prize, not Art. It’s worth pointing out that Nobel Prizes for large collaborations are hugely symbolic, but that perhaps for the future they should go to the entire collaboration rather than any individuals. Without these 16 people — and the hundreds that worked on it as it developed — this science doesn’t happen. They all deserve the accolades!
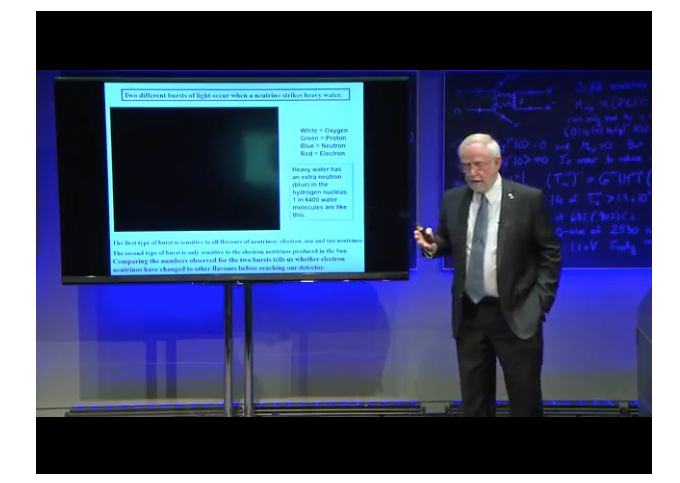
4:30 PM: When you use heavy water, you get a different signal from the interactions with electron neutrinos from the interactions with electrons, and that allows you to tell “what’s an electron neutrino and their interaction rate” apart from “what are the total neutrinos and their interaction rate.” This is how you look for oscillations!
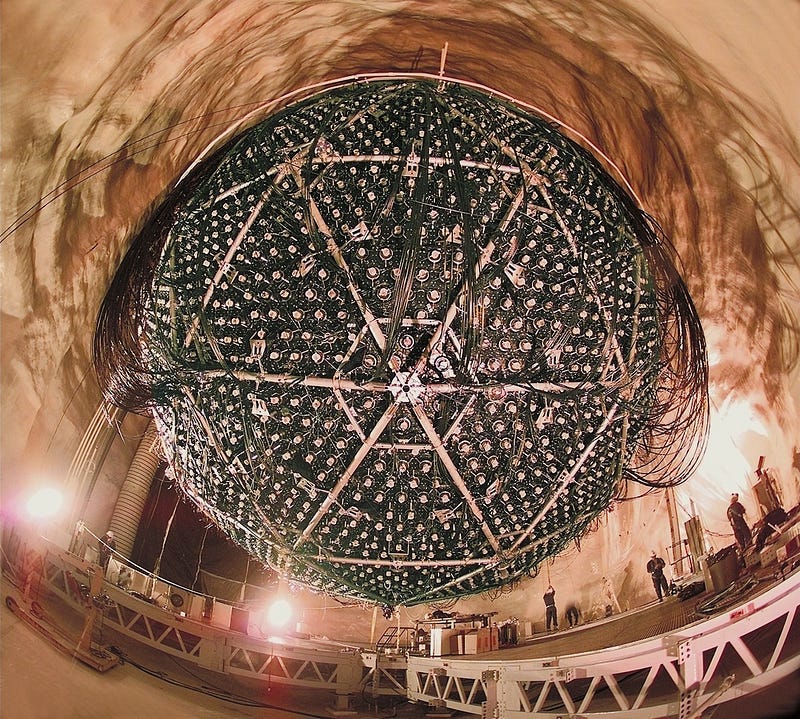
4:33 PM: How big is Sudbury Neutrino Observatory? So not only is it two kilometers underground in the mines, but it’s 34 meters (10 stories) in diameter, covered in lead (for further shielding) and photomultiplier tubes, so you can “see” the individual photons produced by neutrinos in the first place. By the way, here’s a fun fact: the whole reason those mines are there at all? A meteor strike and the interesting, rich, rare deposits that resulted!
4:36 PM: An important addition “at the end” to Sudbury Neutrino Observatory were neutron counters! Count the neutrons and you know a better value for your “noise” that you’re accounting for. (They’re also neutral, and can be confused with neutrinos.) What’s funny is they put on a yellow coat of paint to the remote submarine, but the yellow paint was radioactive, and they had to scrap it! (Green paint was okay.)
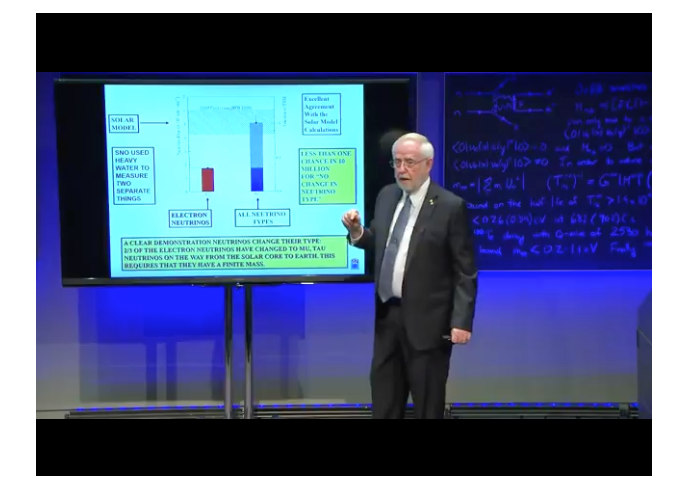
4:38 PM: Here’s the money shot! On the left, the electron neutrinos detected via one method. On the right, the “total” neutrinos detected via the other method. So they are oscillating, and this is the measurement that proves it!
4:40 PM: Theoretically, why do neutrinos need to be massive to oscillate? Think about what happens to time as you move closer to the speed of light: it slows down. If you move at the speed of light, it effectively stops entirely. So in order to oscillate — to change flavor — they need to experience time. And the only way they can do that is if they’re moving slower than the speed of light, and hence they need to have mass!
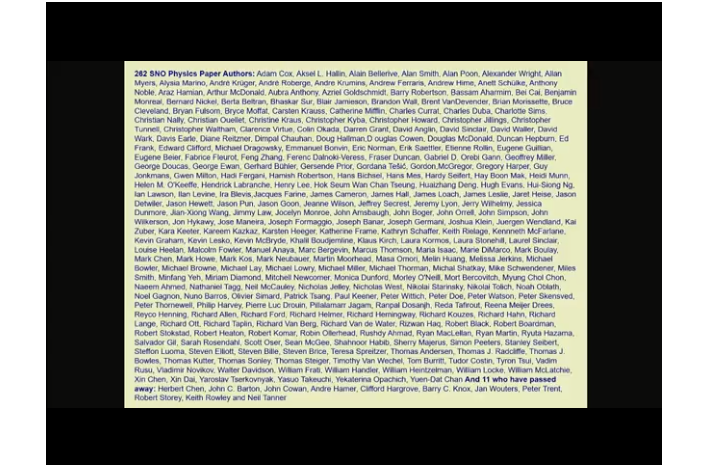
4:44 PM: The 262 living (and 11 deceased) members of the SNO collaboration who’ve contributed to this discovery. For me, the big discovery (4:38 and 4:40) is the most important part for all of us. But for Art — and you can tell this is personal — the hundreds of people who made it happen is the most important part of this talk and of this discovery. It’s hard to argue with that.
4:47 PM: One possibility for baryogenesis — of the matter/antimatter asymmetry and where it came from — is that the neutrino is its own antiparticle, that a special type of radioactive decay (neutrinoless double beta decay) occurs, and that ultra-heavy particles that might be related to the neutrinos we see play a role in that baryogenesis scenario. That’s one of the things SNO+, the Sudbury Neutrino Observatory’s current/future project, is looking for now.
4:51 PM: Double beta decay can happen, by the way, with two neutrinos, and that has been observed! Double beta decay with no neutrinos hasn’t been seen, and occurs at a rate at least 10,000 times slower than the rate with neutrinos. If we don’t see it down to a factor of about 10–100 million, then neutrinos aren’t, in fact, their own antiparticles.
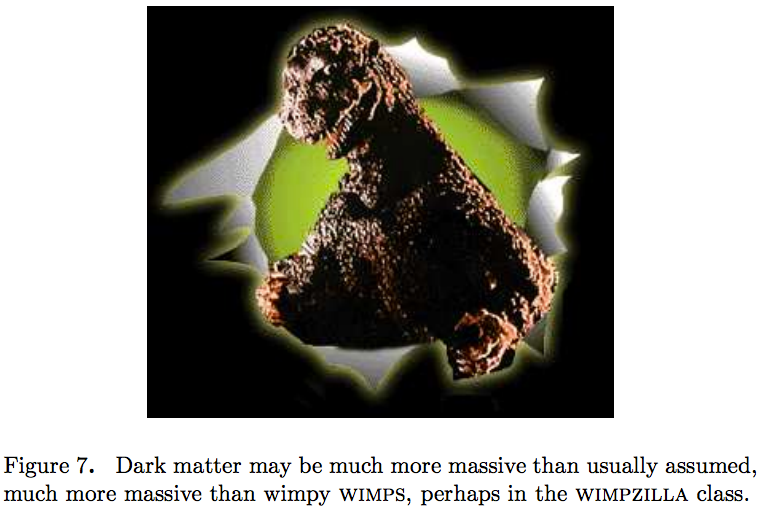
4:53 PM: From a dark matter perspective, the ultra-heavy neutrinos that give our neutrinos their tiny mass might be a good dark matter candidate: WIMPzillas!
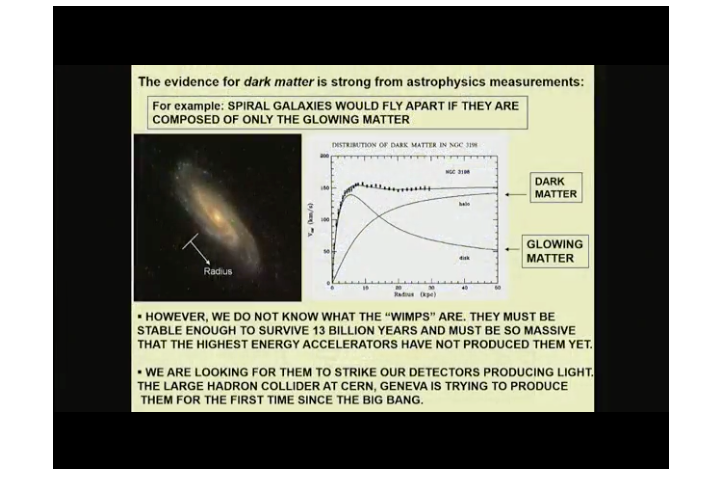
4:55 PM: A more mundane candidate are regular WIMPs, which would interact with the same types of things that neutrinos do, but with different masses and cross-sections. SNOLAB is looking for this type of dark matter in a similar fashion — the nuclear recoil fashion — to one of the detection mechanisms for neutrinos used in its earlier incarnation.
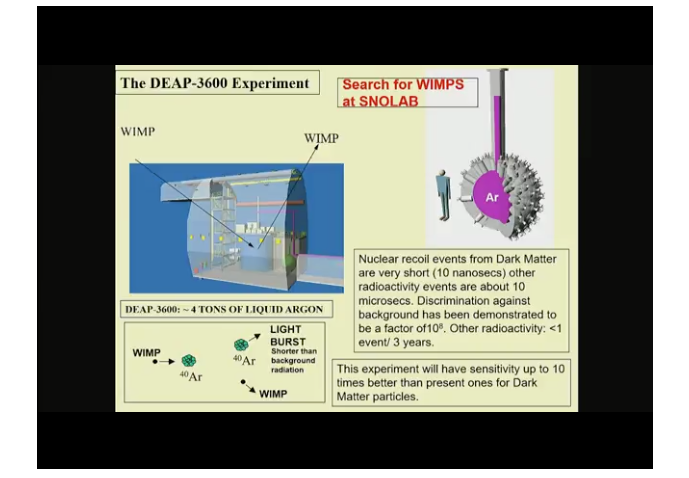
4:58 PM: The key setup for one of the WIMP experiments is by filling the cavity (a smaller cavity, mind you) with liquid argon, ruling out all the standard, radioactive events that occur, getting the background down to zero events in three years (!), and then searching for WIMPs that give a different, unique signal. If they find it, amazing! But this is highly dependent on what type of dark matter we have; they have to be:
- of a particular mass (~100–1,000 GeV),
- interacting through the Weak interactions (and they may not),
- and that the neutrino background doesn’t scatter at a greater cross-section than the WIMPs do even if those interactions do occur.
It’s ambitious, but it’s possible and even probable that this will return a null result. High reward science is often high risk, too!
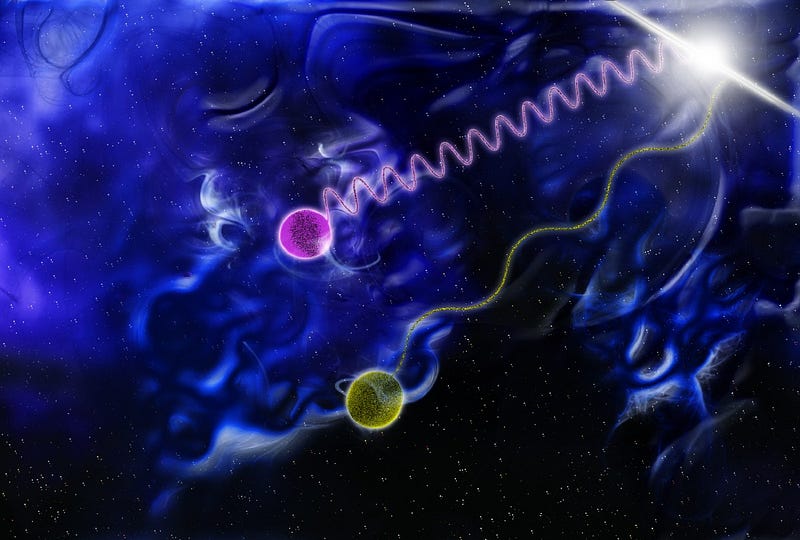
5:00 PM: Another question for me from Twitter — when neutrinos oscillate, do they change speed? — and the answer is yes, but good luck seeing it. These particles are created with energies of ~MeV to GeV, while their masses are ~milli-eV, a factor of billions to trillions difference. So yes, there might be a difference in speed, but the difference is between 99.9999991% and 99.9999992% the speed of light. Good luck seeing that.
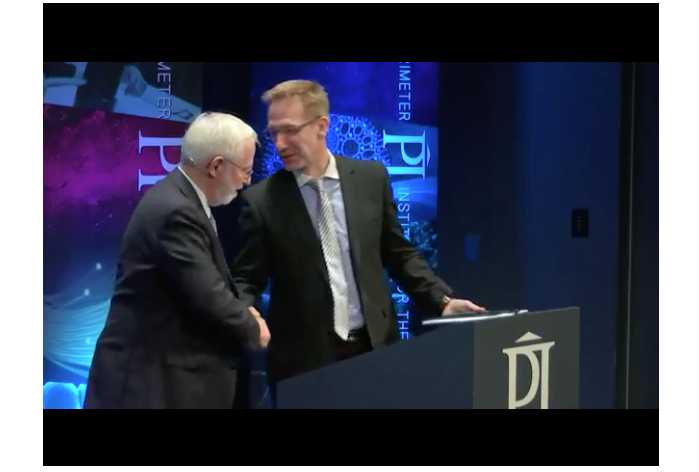
5:04 PM: This was a really great, accessible talk, and not only recounted some remarkable history, but some outstanding science still occurring today. We have a chance to see neutrinos:
- outside of our own galaxy,
- from supernovae or gamma-ray bursts,
- from the atmosphere (from cosmic rays) or from the Sun,
and to see exotic signals beyond neutrinos, and to distinguish neutrinos from them as well. Things are really exciting for neutrino physicists and neutrino astronomers!
5:07 PM: There’s still no absolute mass measurement, by the way, but the neutrinoless double beta decay experiment, if it’s successful, will tell us the absolute mass. So we don’t have it yet, but it might be coming down the road!
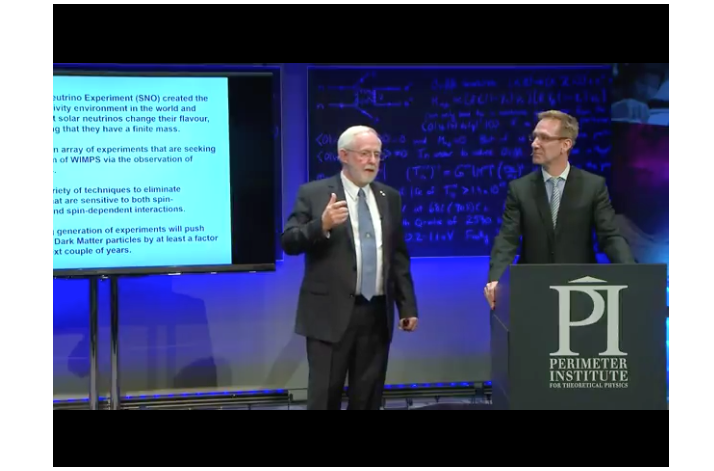
5:09 PM: The best question so far comes from the youngest question-asker: wouldn’t you have to worry about using radioactive materials (Tellurium, for example) in an experiment designed to shield you from radioactivity? You’d worry that would contaminate your results, but you’re only looking for results in a particular energy range, and so if the energy range you’re looking at is higher than the energies of the radioactive decays, you’re safe.
5:12 PM: SNO isn’t the biggest detector; Super-Kamiokande in Japan was much bigger and made huge contributions to neutrino physics. But SNO was sensitive to both solar and atmospheric neutrinos, and that’s what made it so powerful in terms of its science.
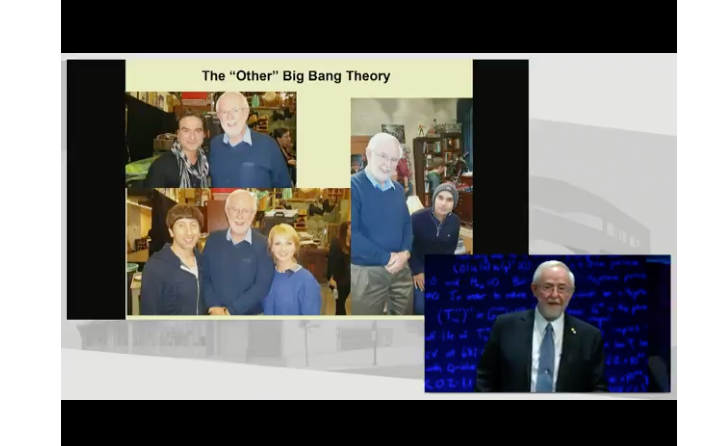
5:14 PM: Art McDonald was very impressed with the level of scientific accuracy (minus the fanciful-ness) of the show, but the commitment to accurate blackboards, the professional nature and the attention to detail of real problems (like black-market purchases of liquid helium!) were for him the best part.
Thanks for a great talk, everyone, and thanks for tuning in. You can always (re-)watch the video and re-read the live blog at any time and follow along: 4:00 PM corresponds to the video’s start!
This post first appeared at Forbes. Leave your comments on our forum, check out our first book: Beyond The Galaxy, and support our Patreon campaign!