Will Humanity Achieve Interstellar Travel And Find Alien Life?
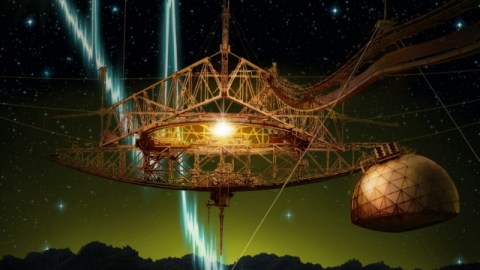
Two of our biggest science-fiction dreams might not remain fiction for much longer. Here’s how 21st-century science could make it real.
For as long as human beings have looked up at the stars in the sky, two questions have captured our collective imaginations: are there other life forms out there on any of their worlds, and will we ever realize the dream of traveling to one of them? Although both tasks appear to have enormously daunting technical challenges, recent advances in science suggest that not only might humanity be capable of overcoming them, but we might even do so later this century.
While faster-than-light travel and visitations from aliens — whether benign or malevolent — are staples of our science-fiction stories, it’s plausible that our real-life scientific advances may legitimately be more profound than any fictional stories humans have dreamed up. On the edge of both frontiers, humanity may be on the cusp of achieving a dream as old as humanity itself.
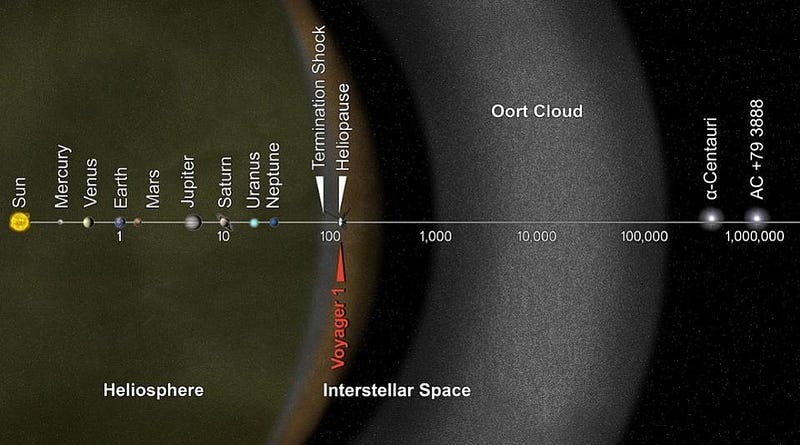
The biggest problem with the idea of interstellar travel is scale. The distances to even the nearest stars are measured in light-years, with Proxima Centauri being our nearest neighbor at 4.24 light-years away, where one light-year is approximately 9 trillion kilometers: some 60,000 times the Earth-Sun distance. At the speed of the fastest space probes humanity has ever sent on their way out of the Solar System (the Voyager 1 and 2 spacecraft), covering the distance to the nearest star would take approximately 80,000 years.
But all of this is based on current technology, which uses chemical-based rocket fuel for propulsion. The biggest downside of rocket fuel is its inefficiency: one kilogram of fuel is capable of generating just milligrams’ worth of energy, as measured by Einstein’s E = mc². Having to carry that fuel on board with you — and requiring that you accelerate both your payload and the remaining fuel with that energy — is what’s hamstringing us right now.
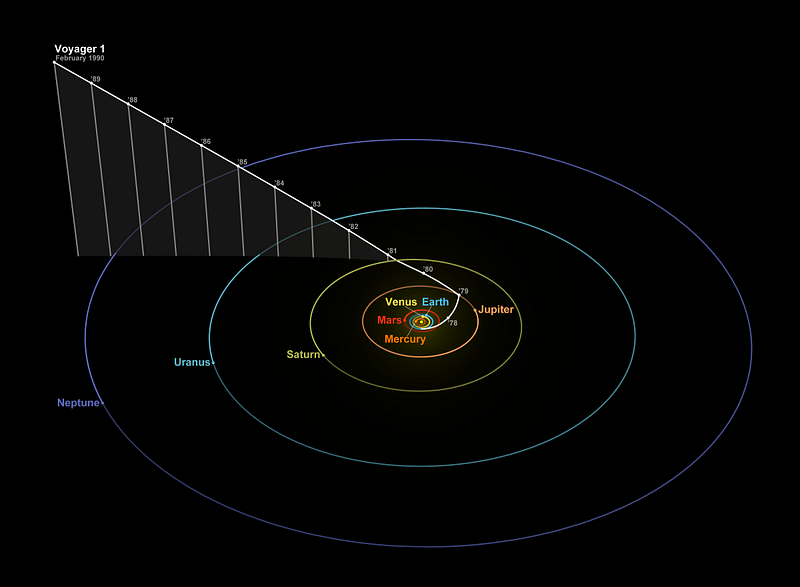
But there are two independent possibilities that don’t require us to dream up Warp Drive-like technologies that would rely on new physics. Instead, we can pursue the routes of either using a more efficient fuel to power our journey, which could increase our range and speeds tremendously, or we can explore technologies where the thrust-providing source is independent of the payload that’s going to be accelerated.
In terms of efficiency, there are three technologies that could vastly outperform chemical-based rocket fuel:
- nuclear fission,
- nuclear fusion,
- and matter-antimatter propulsion.
Whereas chemical-based fuels convert a mere 0.0001% of their mass into energy that can be used for thrust, all of these ideas are far more efficient.
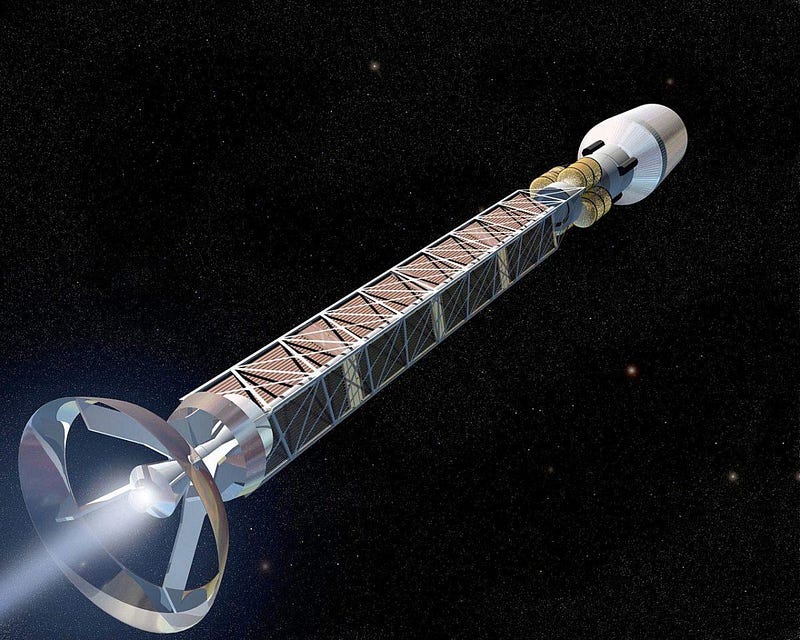
Fission converts approximately 0.1% of the mass of fissile materials into energy; approximately one kilogram of fissionable fuel yields about one gram’s worth of energy, via E = mc². Nuclear fusion does a superior job; fusing hydrogen into helium, for example, is 0.7% efficient: one kilogram of fuel would yield 7 grams’ worth of usable energy. But far-and-away the most efficient solution is matter-antimatter annihilation.
If we could create and control 0.5 kilograms of antimatter, we could annihilate it at will with 0.5 kilograms of normal matter, creating a 100% efficient reaction that produced a full kilogram’s worth of energy. We could conceivably extract thousands or even a million times as much energy from the same amount of fuel, which could propel us to the stars on timescales of centuries (with fission) or even just decades (with fusion or antimatter).
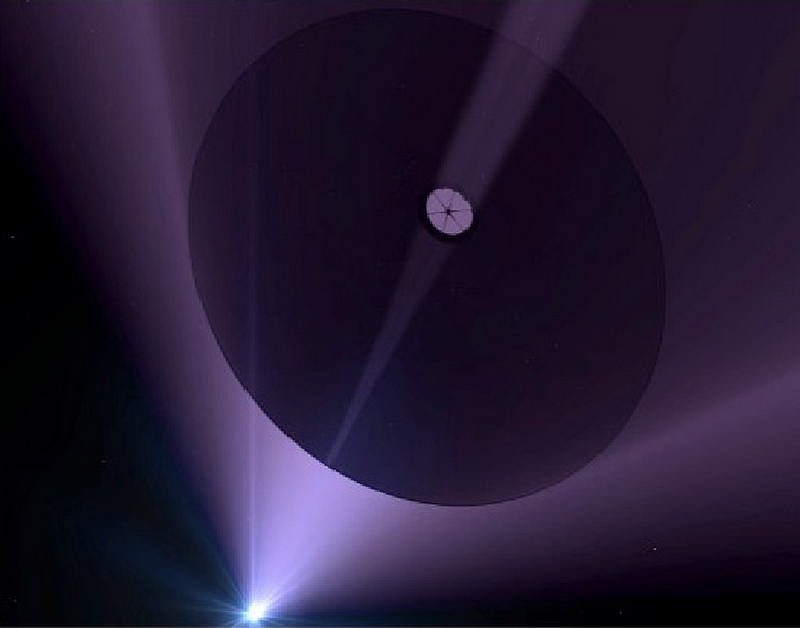
On the other hand, we could work to achieve interstellar travel via a completely different route: by placing a large power source capable of accelerating a spacecraft in space. Recent advances in laser technology have led many to suggest that an enormous, sufficiently collimated array of lasers in space could be used to accelerate a spacecraft from low-Earth orbit to tremendous speeds. A highly reflective laser-sail, like a solar sail except specifically designed for lasers, could do the job.
If a large-enough, powerful-enough array of in-phase lasers were constructed, potentially reaching gigawatt power levels, it could not only impart momentum to a target spacecraft, but could do so for a long period of time. Based on calculations performed by Dr. Phil Lubin a few years ago, it’s possible that speeds up to 20% the speed of light could be reached. While we don’t yet have a plan for decelerating such a spacecraft, reaching the nearest star in a single human lifetime is within the realm of possibility.
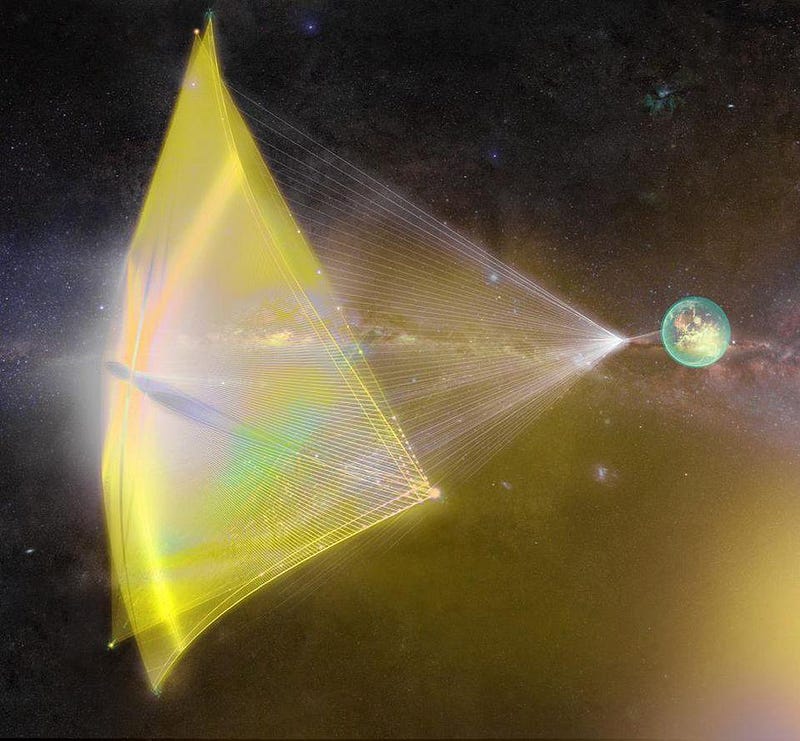
By the same token, the search for extraterrestrial life is no longer restricted to either waiting for an alien visit or searching the Universe with radio signals for intelligent aliens, although the latter is certainly still an active scientific field spearheaded by SETI. Although no signals have been found, this remains a stunning example of high-risk, high-reward science. If a positive detection is ever made, it will be a civilization-transforming event.
However, as exoplanet astronomy continues to advance, two techniques that have already been demonstrated could bring us our first signatures of life on other worlds: transit spectroscopy and direct imaging. Both of these involve using the light from a planet itself, with transit spectroscopy leveraging the light that filters through a planet’s atmosphere and direct imaging taking advantage of the sunlight directly reflected off of the planet itself.
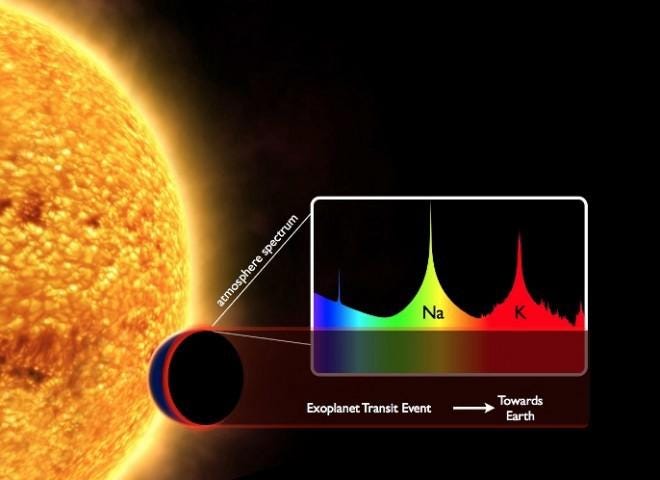
Transit spectroscopy relies on us having a serendipitous alignment of our observatory with both a target exoplanet and its parent star, but these alignments do occur. Whereas a small fraction of the star’s light will get blocked by the transiting planet, an even smaller fraction of starlight will transmit through the planet’s atmosphere, similar to the sunlight that gets transmitted through Earth’s atmosphere and lights up the Moon (in red) during a total lunar eclipse.
This enables us, if our measurements are good enough, to decode what elements and molecules are present in the target planet’s atmosphere. If we could discover biological signatures or even technosignatures which could be an oxygen-nitrogen atmosphere, complex biomolecules, or even something like a chlorofluorocarbon (CFC) molecule we would immediately have a strong hint of a living world that would tantalizingly await confirmation.
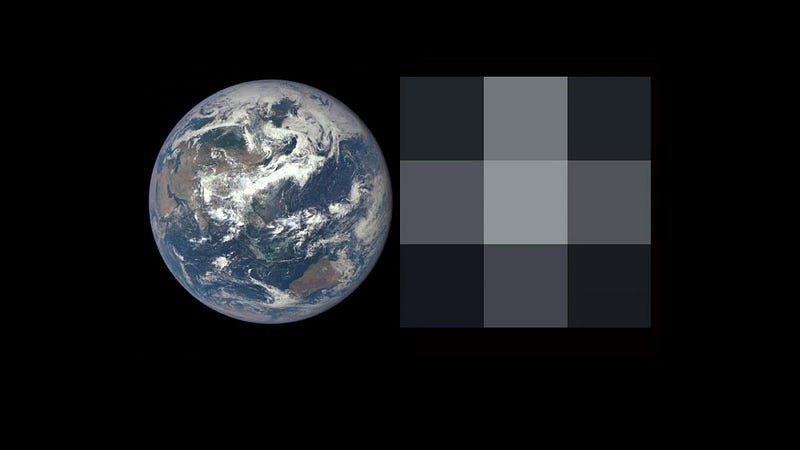
Direct imaging could provide exactly that sort of confirmation. Although our first image of an Earth-sized exoplanet likely won’t be very visually impressive, it will contain a ton of information that can be used to reveal indicators of life. Even if the planet itself is just one pixel in a detector, we could not only break its light apart into individual wavelengths, but can look for time-varying signatures that could reveal:
- clouds,
- continents,
- oceans,
- plant life greening with the seasons,
- icecaps,
- rotation rates,
and much more. If there are light-emitting signatures at night, just as planet Earth has our light that illuminate the world at night, we could conceivably even detect those as well. If there’s a civilization out there on a nearby Earth-like planet, the next generation of telescopes might be able to find them.
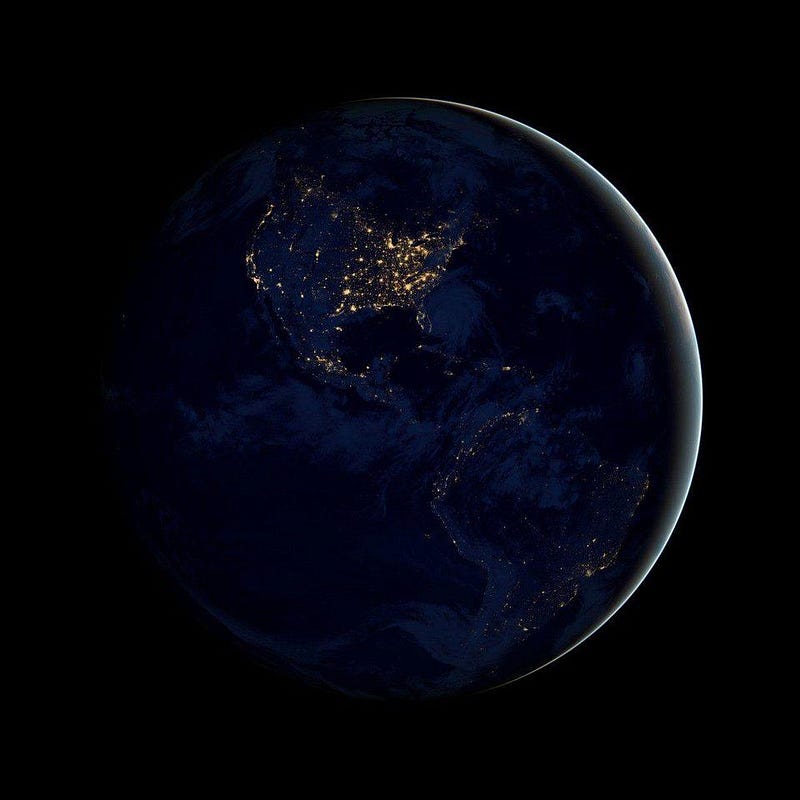
All of this, together, points to a picture where a spacecraft or even a crewed journey to the stars is technologically within our reach, and where the discovery of our first world beyond the solar system with possible life on it could occur in a decade or two. What was once solely in the realm of science-fiction is quickly becoming possible due to both technical and scientific advances and the thousands of scientists and engineers who work to apply these new technologies in practical ways.
On February 5 at 7 PM ET (4 PM PT), Dr. Bryan Gaensler, director of the Dunlap Institute for Astronomy and Astrophysics at the University of Toronto, will be delivering a public lecture at Perimeter Institute on exactly this topic. Titled Warp Drive and Aliens: The Scientific Perspective, it’s available to watch from anywhere on Earth, and I’ll be following along with a live-blog in real time, below.
How close is humanity to achieving this dream that’s spanned innumerable generations? The answer is closer than you might think, so tune in here and follow along below (updates every 3–5 minutes) to find out what lies just beyond the known frontier. It could be the revolution we’ve all been hoping for!
Live blog begins at 3:50 PM Pacific Time, with all timestamps below shown beginning from that starting point.
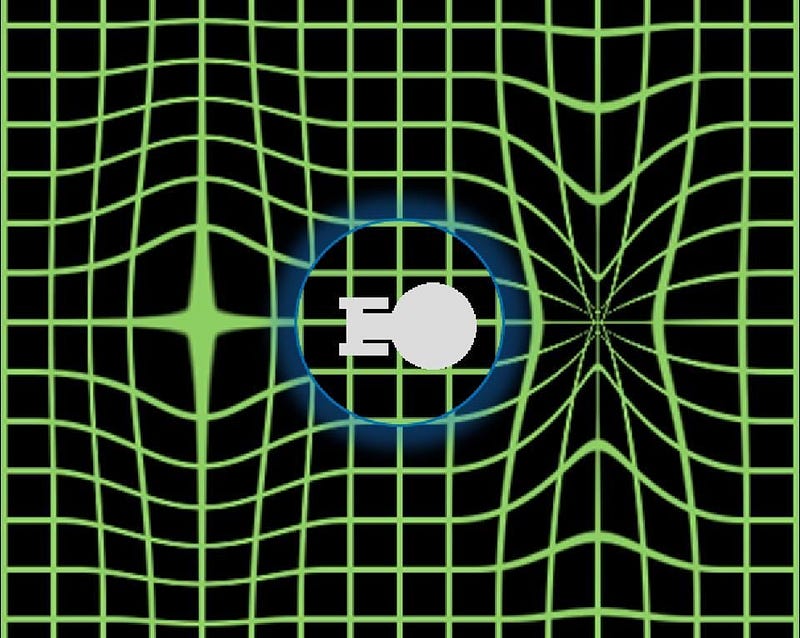
3:50 PM: Okay, warp drive fans, here we go! The first thing you might be wondering is about whether warp drive itself is really feasible or not. And the answer, believe it or not, is maybe, but not unless we figure out a source of energy that goes well beyond anything we’ve got so far, including antimatter.
The reason is simple: to achieve warp drive, you need to bend the space in front of you so that it contracts, and that can only occur at the expense of expanding the space behind you. This takes an enormous amount of energy all localized in one spot, and you need to do it while still keeping the space where your spaceship will be not too severely bent, or you’ll wind up destroying it with terrific gravitational tidal forces.
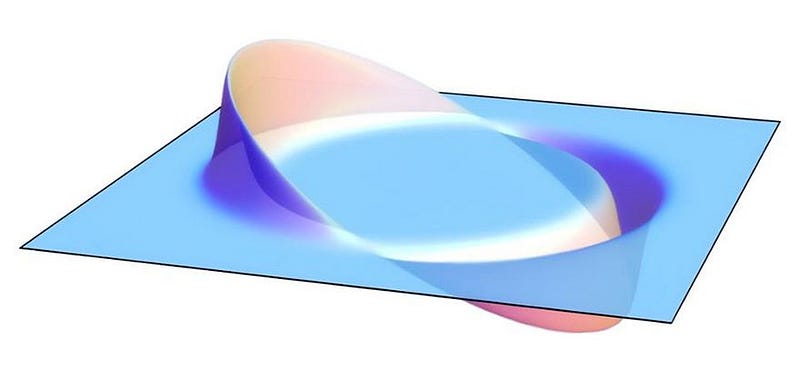
3:54 PM: But if you can do it, and it is something allowed in General Relativity, this requires not only the matter-and-energy we know, but also some form of negative energy: either matter with negative mass or a form of anti-energy itself. If we could harness this, it would mean we could travel through the contracted space (slower than light), but we could do something like contract a 40 light-year journey down to 6 light-months.
Even if we only traveled through that now-contracted space at half the speed of light, we’d get there in 1 year, rather than 40. That’s pretty impressive!
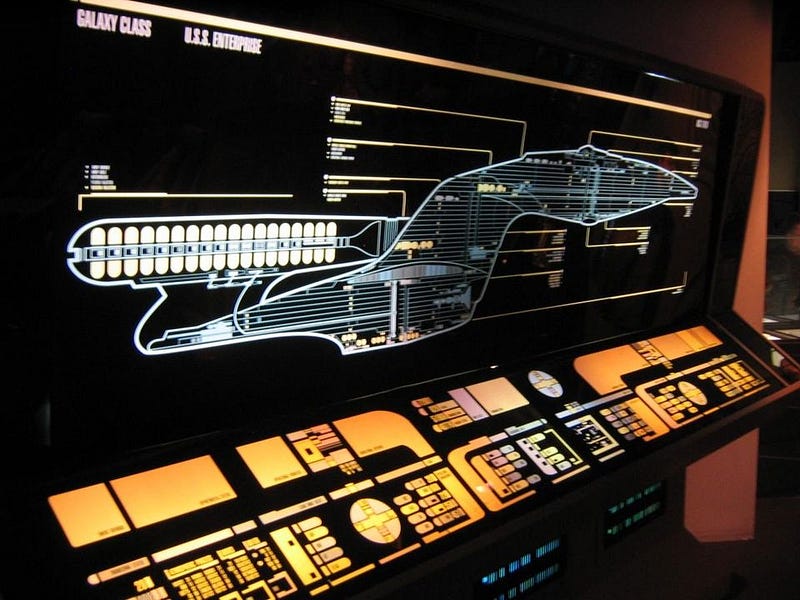
3:57 PM: That doesn’t mean, however, that the plot devices or treknobabble cooked up by Star Trek’s writers, which includes things like:
- dilithium crystals,
- warp nacelles,
- Bussard ramjets
- warp cores,
or anything else we might immediately refer to has any relevance. Science fiction provides us with possible outcomes, but only very rarely gets the path to that technological solution correct. We know enough about physics, today, to be certain that Star Trek’s “solution” to this problem is not feasible. But, then again, that’s part of what makes science so wonderful: it can take a fictional idea and make it a reality. Or, if we’re really lucky, surpass our sci-fi dreams!
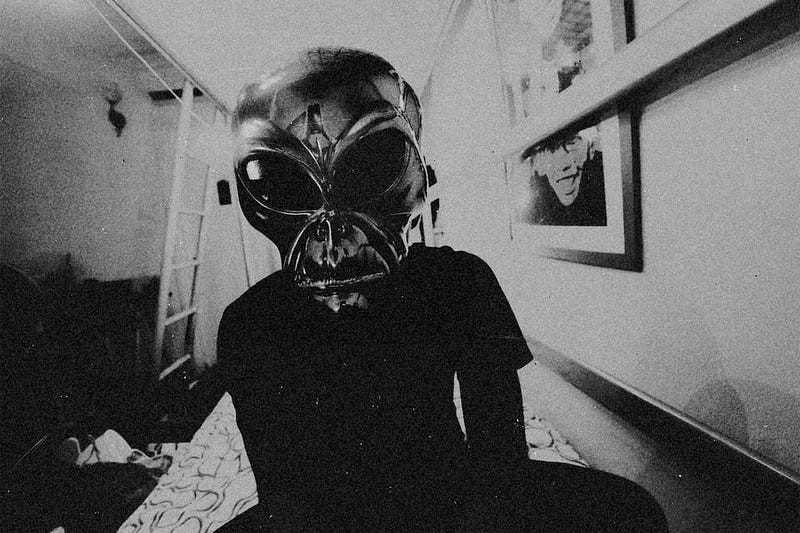
4:00 PM: Aliens, on the other hand, are likely ubiquitous, based on what we know about the ingredients for life in the Universe, the workings of chemistry, and our measurements of exoplanets with the right conditions for life around other stars. We have literally billions and billions of potentially habitable planets in our galaxy alone, with similar conditions to early Earth. In many models, early Venus and Mars were similar to early Earth.
Are we supposed to believe that Earth, where life arose within the first ~3% of our planet’s history, is somehow unique in that regard? Although winding up with something like human beings is a difficult proposition, winding up with no life at all, across billions and billions of other instances with similar initial conditions, seems far more unlikely, at least from a scientific perspective.
4:01 PM: Hooray for another on-time start, as Greg Dick, the executive director of Perimeter Institute, gets us started right on time with his introduction!
4:02 PM: Oh, before I forget, Bryan is Australian, so get ready for an accent, although his won’t be the strongest Australian accent you hear by a long shot!
4:03 PM: And that’s a pretty quick introduction! Here we go; curious what the scientific perspective holds, according to an astronomer/astrophysicist who isn’t me!
4:05 PM: Spoilers: we don’t have warp drive yet, and we haven’t found the aliens yet. Love to hear this up-front, but I also love the optimism that he has that science can make pretty much all of our non-laws-of-physics-violating dreams come true. I think, at its best, this is the dream we all have for science.
4:07 PM: Bryan absolutely talks about an important aspect of being exposed to not just answers of what we do know, but what the frontiers of science are, what’s unknown, at a young age. As a five year old, to discover that adults, parents, teachers, and even experts (libraries and encyclopedias) didn’t know the answer to everything.
And that there are people who figure out the answers to those questions, and they’re just ordinary people, and that he could be one of them.
Please note that this applies to everyone! You can do it, too, and you don’t have to figure that out at age 5 to do it.
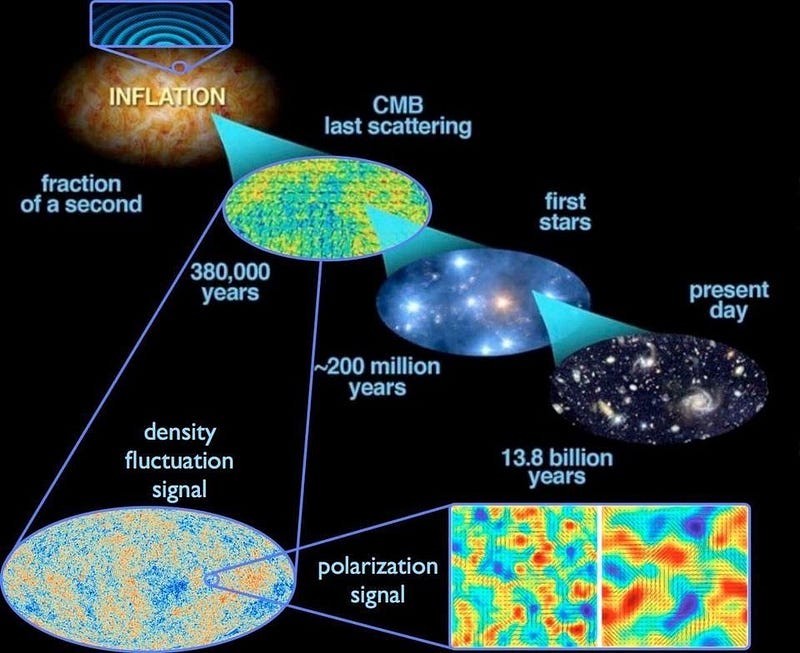
4:10 PM: And this is a lot of fun, too: the fact that questions we didn’t even know we needed to ask can be revealed by finding the answers to previous scientific questions. In the 1920s, we didn’t know the Universe was expanding, but its discovery led to the idea of the Big Bang. In the 1960s, we didn’t know that the Big Bang was true, but its confirmation led to questions about what came before it and what our Universe’s ultimate fate would be.
And now, as you can see, we’re talking about the mysteries of cosmic inflation and dark energy, which are where those frontiers now lie. And in any field, this is how it works: discovering an answer only reveals a deeper frontier that we haven’t yet explored.
4:11 PM: I like Bryan’s delineation between the difference between science and science fiction. Science is all about discovering and following the rules; science-fiction is about breaking those rules. I haven’t explicitly thought about it in those terms, and I agree that this is pretty much how it usually works. I don’t know that this is why I, personally, like or don’t like various forms of science-fiction, but it’s a new perspective to think about for me.
4:13 PM: We constantly have advancing technology, and science-fiction asks the question of how advancing technology will change our lives. He brings up the example of Westworld, which I like, but I really think he missed a golden opportunity to reference Black Mirror, which really highlights and elevates the dystopian aspects of our society in a new way in each episode.
4:15 PM: Alright, some science! Here we are, moving on to interstellar interloper ‘Oumuamua, one of the things we’ve seen that wasn’t particularly anticipated, even by science fiction. And yet, Bryan is correct to point out that Star Trek IV: The Voyage Home, had a cigar-shaped alien asteroid in our own solar system.
It’s not, of course, telling us to save the whales, and it’s not a space probe, but it’s remarkable that science fiction had this idea before astronomers or any scientists knew it was coming.
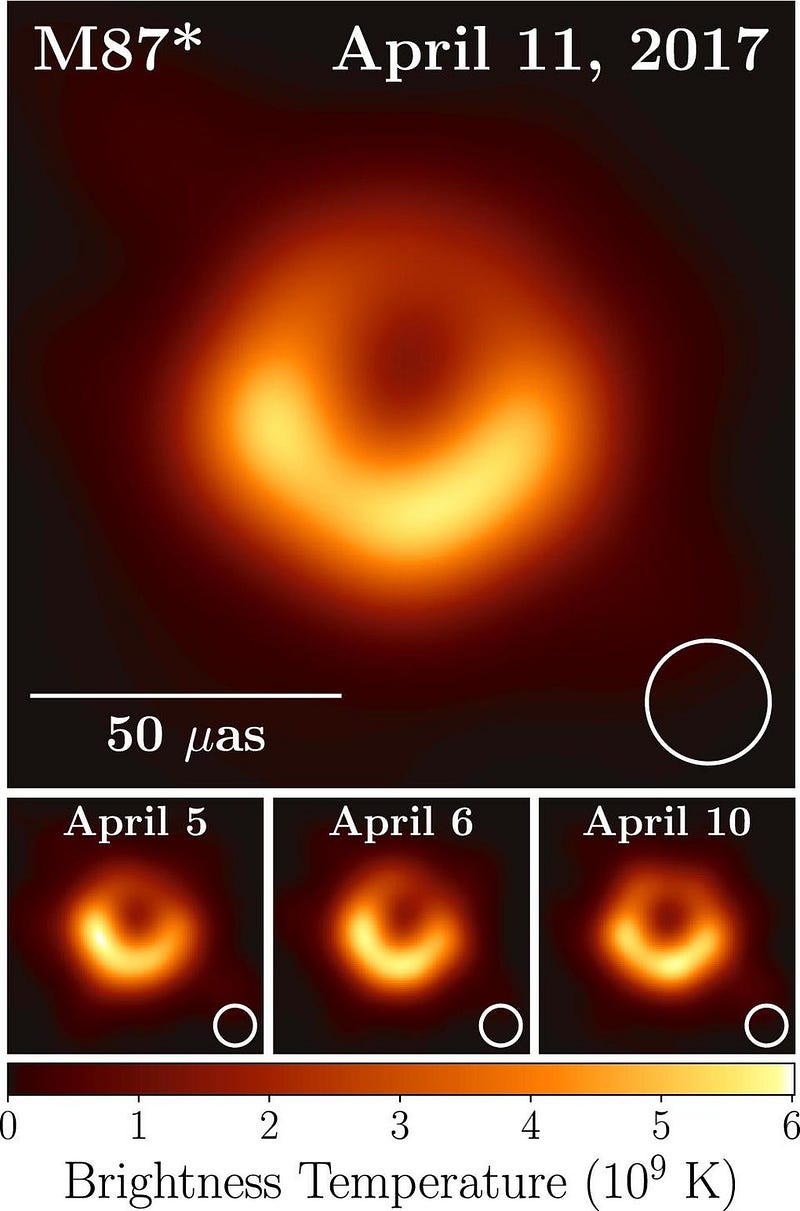
4:18 PM: This one is a little bit less fair. When you’re talking about older movies that talk about black holes, it’s really unfair to talk about how “we knew what black holes would look like” in science-fiction, because black holes have been astrophysically theorized for decades, going back to the 60s, 50s, or even 1916 in the context of General Relativity, and even earlier (the late 18th century) in Newtonian gravity.
Sure, it’s fascinating, but visualizations, based on a mix of science and artistic license, have been around for as long as we’ve even known enough about science to imagine what could realistically be. Also, side note, the “interstellar” black hole is probably not very likely to be what we see when we examine our realistic black holes in supreme accuracy; there’s a lot of artistic license and some likely unphysical assumptions that were made for Insterstellar.
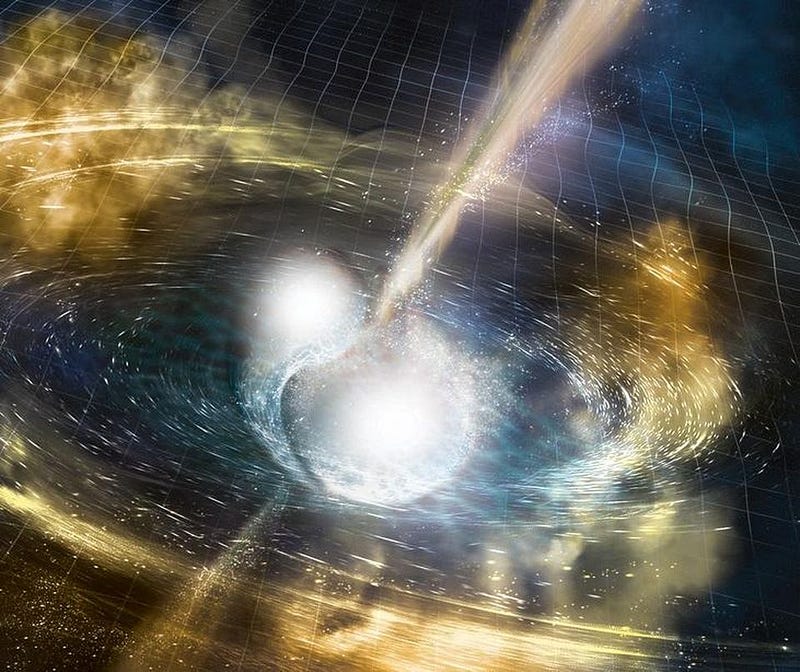
4:22 PM: I also don’t quite think it’s fair to say “well, we simulated and visualized this astrophysical event,” and then “we observed it,” and “that’s an example of science outstripping science fiction.
Yes, it’s true that the entire Universe shook… but not every scientific event, including one that involves planet Earth “shaking” by less than an atom’s width, makes for particularly good science fiction. He said earlier, remember, that science fiction was about investigating the human condition. It’s hard to see how a tiny, subtle effect like that would make for a good sci-fi story.
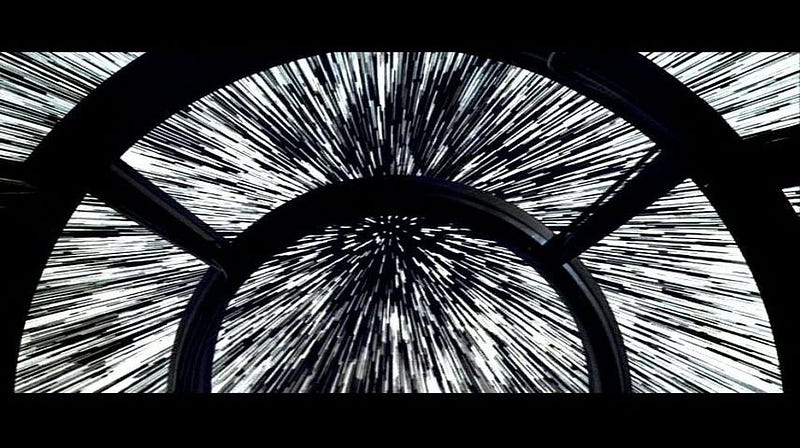
4:25 PM: Okay, this is a pet peeve of mine. Do you know why things like rockets and space shuttles have the shapes they do? That elongated, narrow-cone shape you’re familiar with? It’s because of atmospheric drag.
If you’re going to build your ship in space, and fly it only in space, you don’t need to factor in aerodynamic considerations at all! You’d be much, much smarter to build a structure with a good volume-to-surface-area ratio: a sphere. The Death Star, not the Millennium Falcon or an X-Wing, is going to be much more practical for structures we build in space!
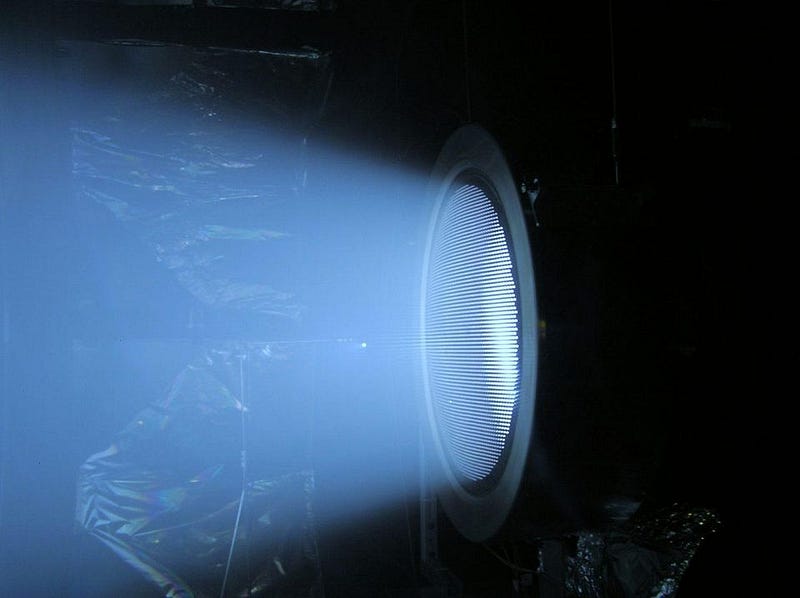
4:28 PM: Ion drives are real, and they’re very cool. But if you want to power a journey across large distances in a reasonable amount of time, ion drives won’t get you far at all. They can take you ~6 billion kilometers over 11 years, as Bryan said, and can do so pretty efficiently. But if you factor that distance over that time as a “mean acceleration,” you get something truly atrocious: 100 nanometers/second².
You’re… not going to go very far very fast. ~100,000 years to the nearest star, same as conventional fuel. I’ll pass, thank you.
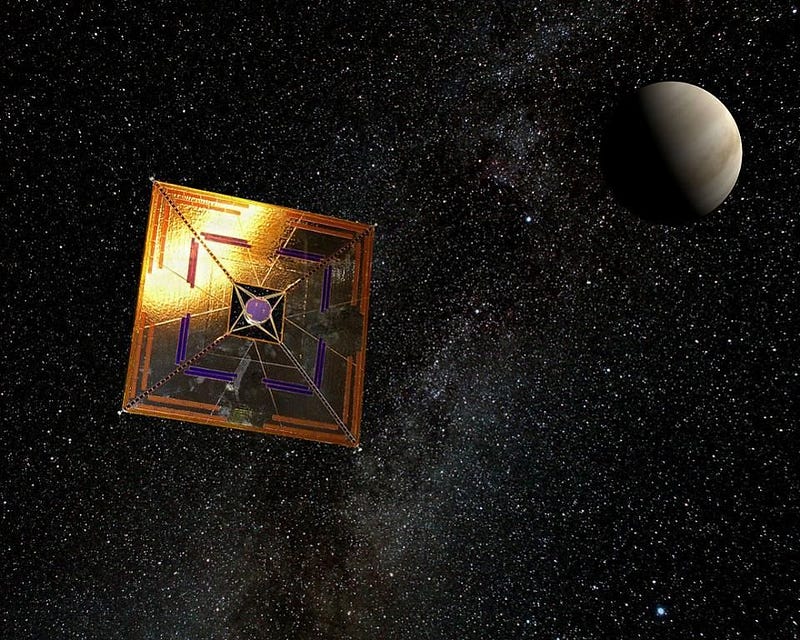
4:30 PM: Hey, solar sails! Yes, if you accelerate with a solar sail, you can decelerate with a solar sail! The “fuel” is simply radiation provided by a star, so as long as you visit a star comparable to the Sun, you could decelerate the same way you accelerated.
Unfortunately, this technology is inferior to ion drives not only in terms of distance reached, but in terms of acceleration and control over your spacecraft. It’s a nice idea, but it’s an idea that’s in its infancy, at best, despite being proposed more than 400 years ago by Johannes Kepler!
4:32 PM: 75 years?! That’s… that’s going to assume a very light payload and a very, very large and efficient over a distance of 1.8 kilometers. Can we do that for ~4 light years, or 20 trillion kilometers. That’s… well, good luck is all I’ll say.
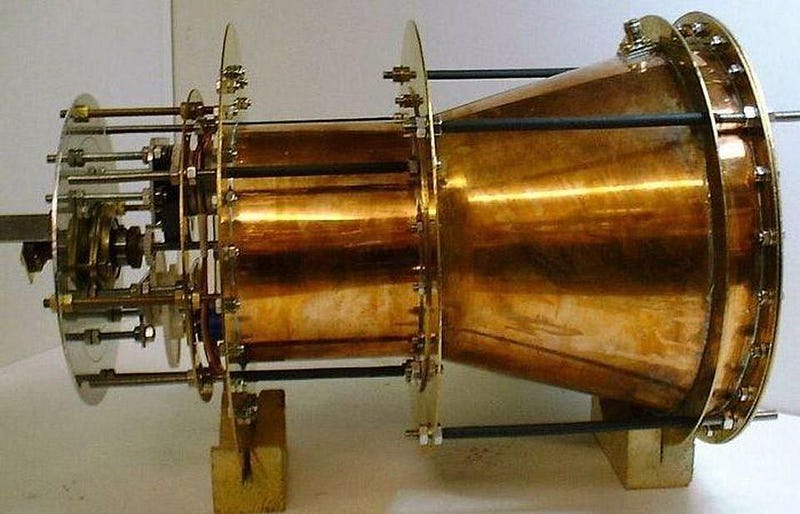
4:33 PM: Hey, don’t be out of date, Bryan! The Em Drive was totally debunked a few years ago. Nice idea, but it’s done.
4:36 PM: Remember, what “quantum teleportation” is doesn’t involve teleporting a particle, it involves teleporting the quantum state of a particle. And Bryan gets that right, but this doesn’t solve the problem of teleporting an inanimate object, much less a person.
4:38 PM: Yes, you need a lot of information to encode a human being. Remember that there are around ~10²⁸ atoms in the human body, and that means something like 10²⁹ or 10³⁰ quantum bits of information. As Bryan says, “I don’t think we’ll be teleporting anytime soon.”
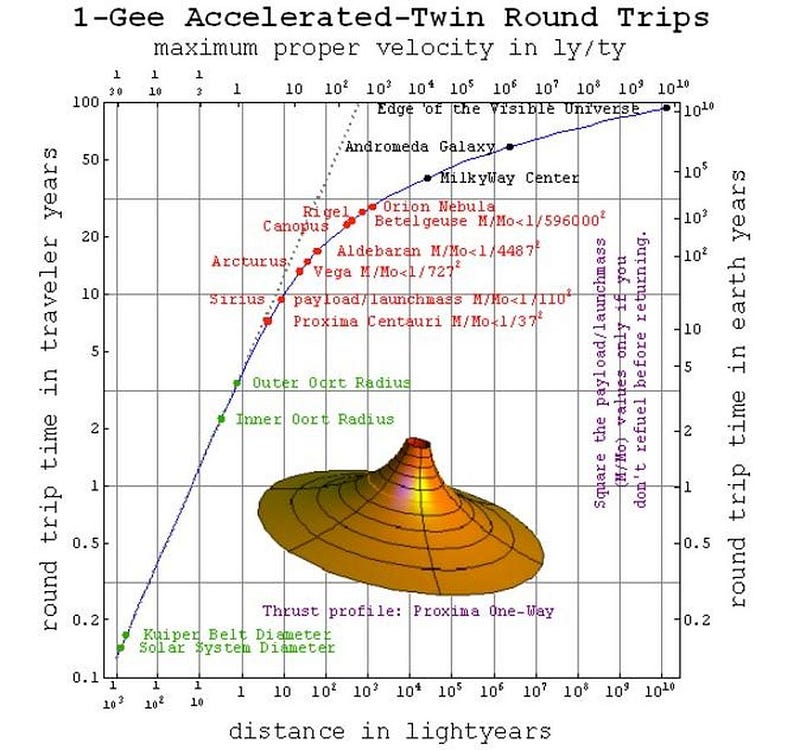
4:40 PM: Hey, don’t be mad at time dilation! Time dilation is what could get us to the stars in a human lifetime. If you wanted to go more than ~100 light-years, it would always take you more than ~100 years (a human lifetime, at the far end) to get there from the frame-of-reference of a person remaining on Earth.
But if you continue to accelerate at 1 g, or 9.8 m/s², you’ll get to wherever you want to go in a much shorter timescale from your frame of reference, as you travel close to the speed of light. Time dilation rules!
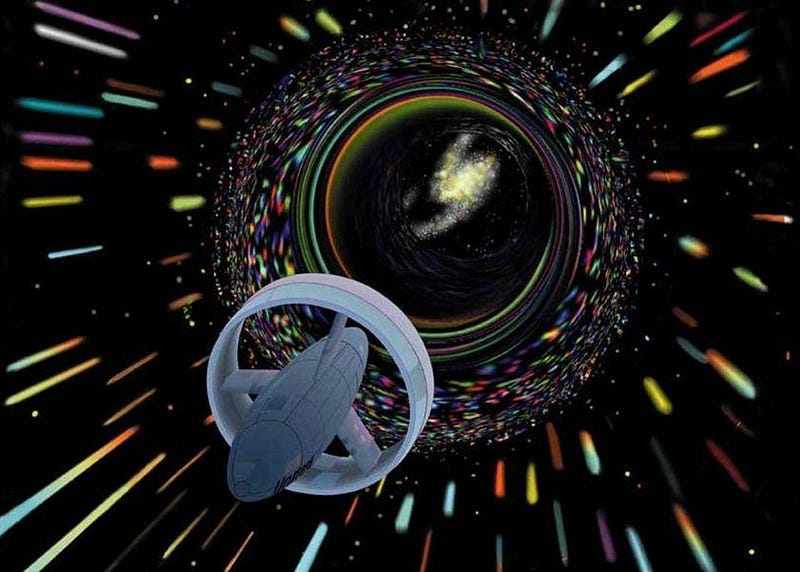
4:42 PM: Okay, really? From long, long-term technologies like ion drives and solar sails straight to warp drive, with nothing in between? In terms of not using fuel, Bryan is correct. But in terms of not using energy… well, good luck transforming your spacetime, where (reminder) spacetime’s curvature is based on matter-and-energy, without expending energy!
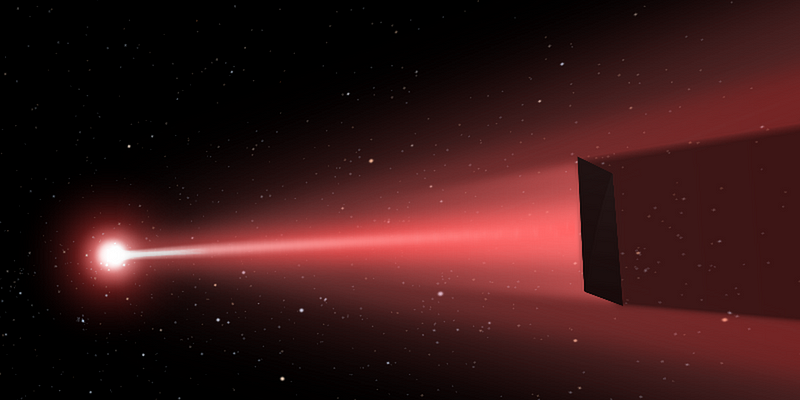
4:43 PM: Wait, he’s going to finish this part of his talk now, talking about Breakthrough Starshot (and the laser sail technology and a “starchip” spaceship) which we mentioned earlier, and cover “aliens” in… what, 10–15 minutes? We’ll see!
4:45 PM: Nope; we’re not onto the “aliens” part yet; we’re talking about femtosatellites, which are still quite large and weigh a few grams, which is still too much for Breakthrough Starshot.
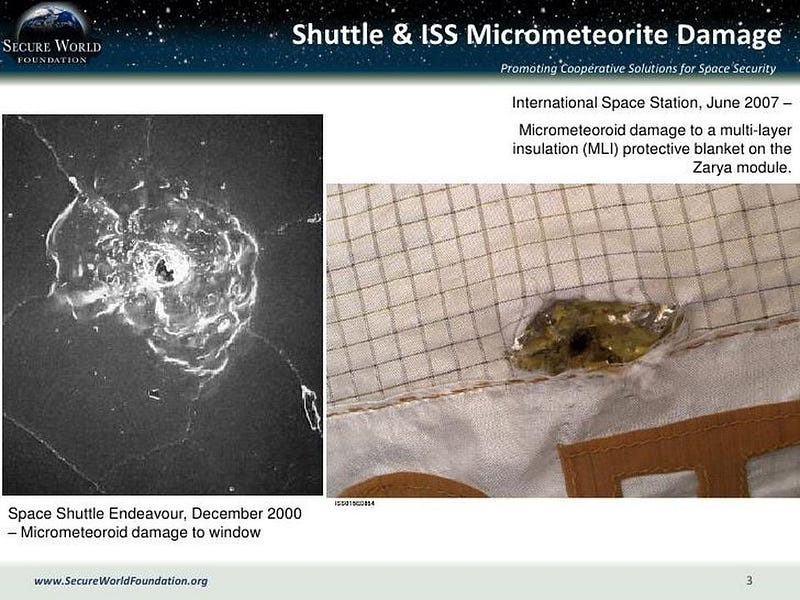
4:48 PM: Yesss! This is something I’m excited to hear, because it’s something that I’ve brought up that few people talk about: when you travel through space at relativistic speeds, you are going to smash into stuff in the interstellar medium! And that stuff is going to erode your spacecraft really fast, and there’s nothing that’s going to protect your “starship” (even if it’s a microchip) from smashing into that dust.
Remember that a little piece of nerf-like foam was all it took, at high speeds, to cause the Space Shuttle Columbia disaster. Remember that all of our spacecraft get hit by micrometeoroids. And remember that 20% the speed of light is about 100 times faster than our fastest spacecraft go, which means they have 10,000 times the kinetic energy from dust particle collisions. This is a harder problem to overcome than anyone has figured out a viable way to reckon with.
4:50 PM: Okay, it’s onto the aliens part, and I have to disagree with what Bryan says. We don’t want to go to planets around other stars to look for life; we want to find planets where life exists (or is likely) and then go there.
There are ~400 billion stars in our galaxy. Do you want to go on a wild goose chase, or do you want to know where you’re going before you go on a decades-long journey across the great void of space?
(Pick the latter.)
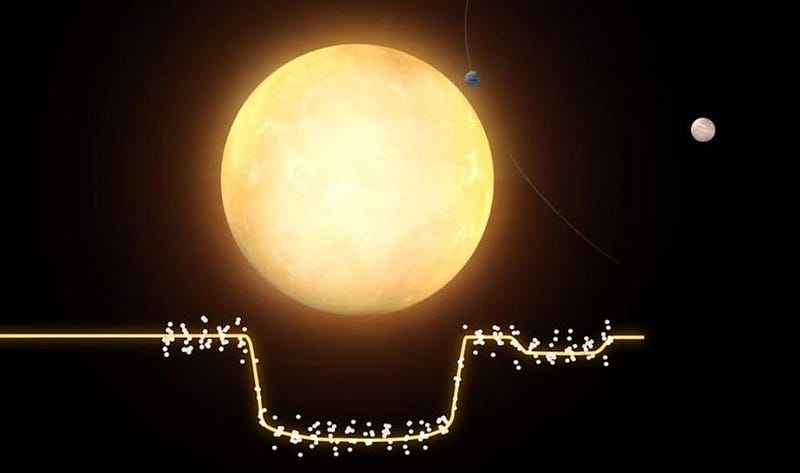
4:53 PM: Using the transit method, we can find out properties of the planets that orbit around the stars, and they come in enormous varieties, just like we’d expect if we didn’t assume the rest of the Universe was just like our little corner. We’ve found the planets that are easiest to find, and that means the largest planets relative to their star in close-in orbits. This, unsurprisingly, has skewed the population of planets that we’ve found.
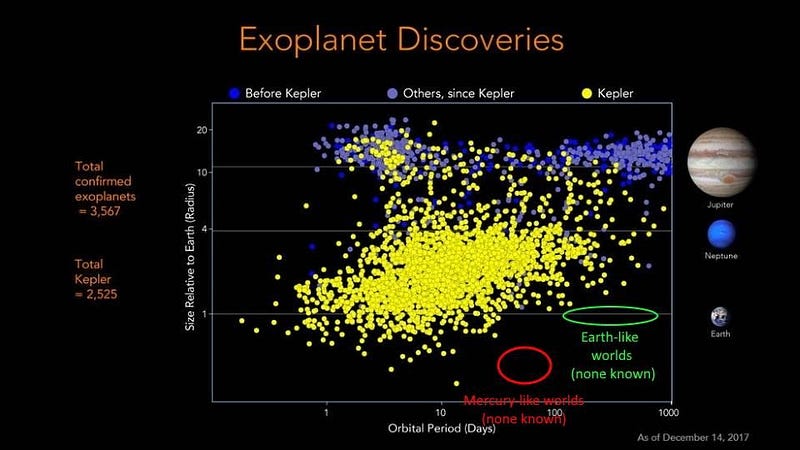
4:55 PM: We have found waterworlds and lava worlds, but these are… well, likely not the best candidates for an “interesting” form of alien life. Nor are hot Jupiters (or any type of Jupiter), or any gas planet with a large hydrogen/helium envelope.
Just like in our own Solar System, most of the planets out there are not expected to have life on them.
4:56 PM: This is a totally unimportant point, but for an astronomer, it’s a pet peeve for many.
The smallest stars in the Universe are red dwarfs. Always dwarfs, never dwarves. The plural of dwarf (for stars) is dwarfs; the plural of dwarf (for the fantasy race of short, stout, beard, axe-wielding characters) is dwarves.
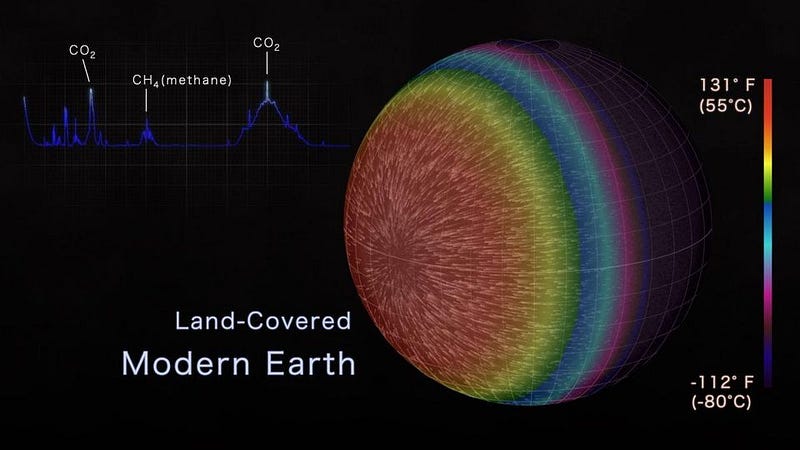
4:59 PM: This is also an important point: what’s happening on a world around a red dwarf star isn’t so much about the irradiance from the star and day/night temperatures and the border between them, but how the atmosphere circulates and what it’s composed of.
We also have to be very careful in distinguishing between “biosignatures,” which is going to be a slam-dunk signal that tells us, “wow, that’s a living planet right there,” and a “bio-hint,” which is what Bryan’s referring to, which is pretty much guaranteed to get you false positives, over and over, before you actually get it right.
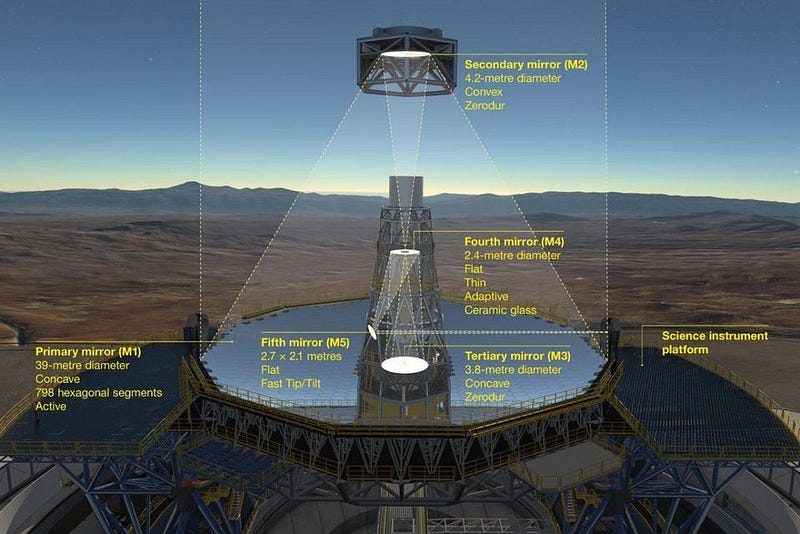
5:01 PM: This is really true: the ELT will be humanity’s best chance, in the 2020s, for directly imaging an Earth-like (or potentially inhabited) planet of any type. This could lead us to a revolution, where bio-hints and bio-signatures could be abundant. Right now, planet-finders like TESS are giving us the best candidate planets for direct imaging, and while we’ll have to get lucky, this is the high-reward science most of us dream about!
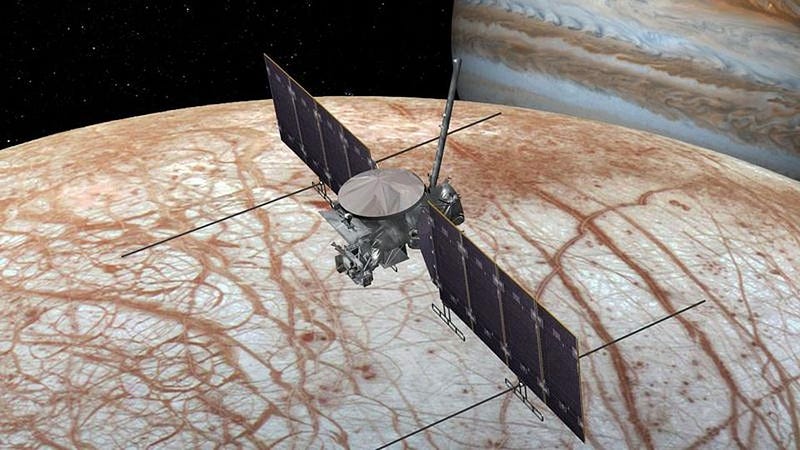
5:04 PM: Of course, this is the third possibility I haven’t discussed for finding life: it could be right here in our Solar System! Do we have life in a subsurface ocean on Europa or Enceladus? Do we have subterranean, potentially seasonally active/inactive life on Mars? Do the outer worlds, like Triton or Pluto, have anything on them of interest?
We have missions going to look, and hopefully in the 2020s, we’ll start to get answers that teach us whether our fantastical interpretations of signals like seasonal methane or organic molecules really hold up. They could be biotic in nature, and we won’t know until we do the appropriate tests!
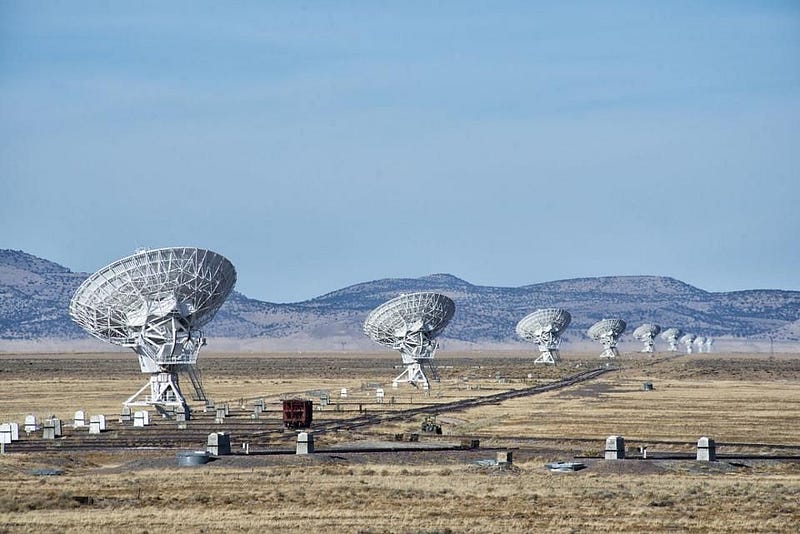
5:06 PM: This is a fun fact: you must not use a walkie talkie around radio telescopes; the interference is atrocious! Remember that people didn’t know what “fast radio bursts” were for much longer than we realized, because the microwave oven in the break room of a giant radio telescope was causing interference? That’s a true story; don’t use walkie talkies near radio telescopes!
5:07 PM: So I think this 1 hour talk has taught me how you talk about two topics when you spend the first 50 minutes on the first topic: just keep talking over your talk’s time!
5:10 PM: The present and the near future are incredibly exciting, and you don’t need warp drive or actual aliens to make it so. But, that said, it would be pretty cool to achieve interstellar travel or to find any true signatures (not just hints + wishful thinking) of alien life.
This is why we do science and why we develop technology; these are our sci-fi dreams and we’re making them come true!
5:12 PM: Alright, talk’s over and we’re into the Q&A. Hey, and the first question is “how do we go from that light of an exoplanet transiting” to “how do we extract all that useful information?” And the two answers are:
- transit spectroscopy, and
- direct imaging.
Bryan is only giving the first answer, but both matter!
5:14 PM: No to aliens in Roswell, New Mexico. Good answer, Bryan. I like the snark of, “why come all this way just to dissect a cow?”
Alright, everyone, that’s all the time I’ve budgeted for today’s talk; hope you enjoyed the live blog and Bryan’s talk! We might not have found aliens yet and we might still be quite far from reaching another star, but our technology has brought us quite an impressive way already, and we’re headed towards something even more spectacular as the 2020s begin to unfold. Stay curious and please join me in looking forward to all the wondrous discoveries that this decade is sure to hold!
Ethan Siegel is the author of Beyond the Galaxy and Treknology. You can pre-order his third book, currently in development: the Encyclopaedia Cosmologica.