Why Isn’t Our Universe Perfectly Smooth?

If it were, we wouldn’t be here. But there’s a scientific answer that’s been spectacularly confirmed.
When we examine our Universe, looking out at the planets, stars, galaxies, and vast cosmic voids separating them, “smooth” isn’t exactly the first word that comes to mind. The enormous cosmic web is one of the clumpiest things imaginable in the Universe, with a planet like Earth some 1030 times denser than average. Yet the Universe wasn’t always this clumpy, or it wouldn’t have evolved to appear the way we see it today. It had to have been born almost perfectly smooth, where the imperfections were just a few parts in 100,000, or it wouldn’t have taken hundreds of millions of years to form the first galaxies. Yet those tiny imperfections were vital, or we wouldn’t have formed the structure we see today at all! After centuries of not understanding how this happened, one of cosmology’s most controversial theories, inflation, provided the answer. And now that our measurements have achieved unprecedented precision, its predictions check out spectacularly.
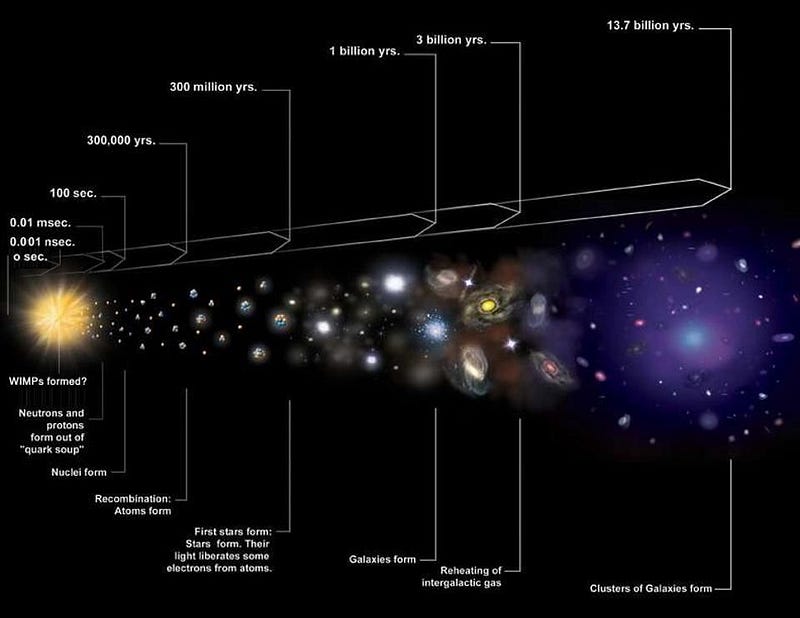
According to cosmic inflation, the hot Big Bang wasn’t the very beginning of space and time, but was merely a hot, dense, rapidly expanding early state. It was cosmic inflation, a phase where the Universe was dominated not by matter and radiation, but by the energy inherent to space itself, that set up the Big Bang. This inflationary phase was characterized by an exponential expansion of space, where the Universe doubled, then quadrupled, then octupled (etc.) in size as time went on. After as little as 10–33 seconds, a region the size of a theoretical string from string theory would have been stretched to a scale larger than the observable Universe is today. In other words, cosmic inflation takes whatever existed beforehand and stretched it really, truly, and perfectly flat-and-smooth.
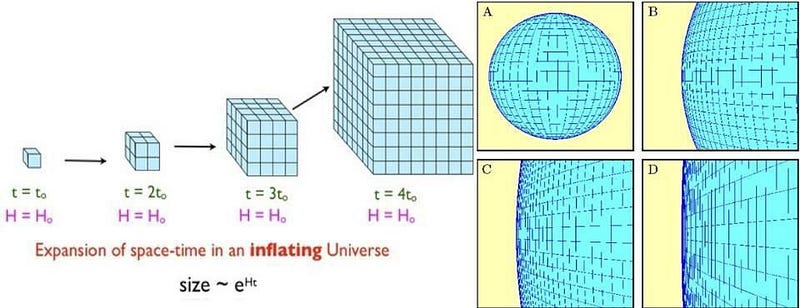
This seems, at first glance, to pose a tremendous problem. If inflation stretches space to be flat, uniform, and smooth, indistinguishably so from perfection, then how did we arrive at a clumpy Universe today? Both Newton’s and Einstein’s theories of gravity are unstable against imperfections, meaning that if you start with an almost-but-not-quite perfectly smooth Universe, over time, the imperfections will grow and you’ll wind up with structure. But if you start with perfect smoothness, with literally no imperfections, you’re going to remain smooth forever. Yet this doesn’t jibe with the Universe we observe at all; it had to have been born with imperfections in its matter density.
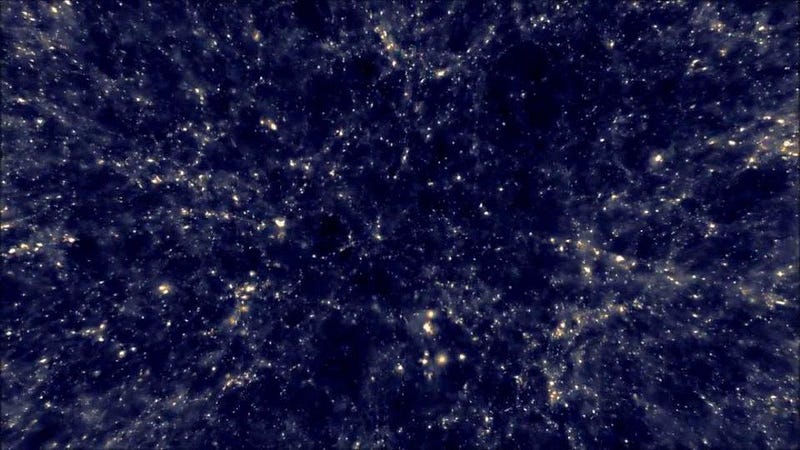
This naive picture of inflation must therefore be incomplete. There has to be some way of generating these imperfections, otherwise the Universe wouldn’t exist the way we see it. But an important property of the Universe, and of inflation, comes to the rescue in the most spectacular of ways. You see, empty space itself isn’t perfectly flat and smooth on its own, but rather, at the smallest scales, exhibits quantum fluctuations.
This can be viewed in many ways: an inherent uncertainty to the energy of space itself; as vacuum fluctuations; or as sets of particle-antiparticle pairs popping in-and-out of existence. But regardless of how you view it, one thing remains clear: if you were to graph the energy density of the Universe, and look at it on extremely small and granular scales, you’d see that it wasn’t uniform and constant in space or time, even if you removed all the matter and radiation from it. There are quantum fluctuations inherent to the fabric of space itself.
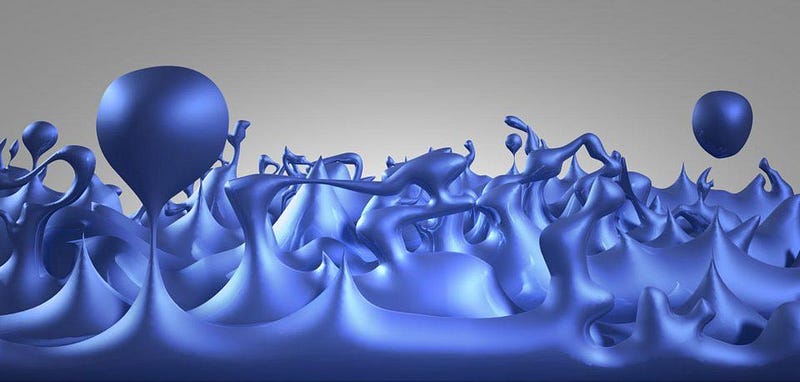
Normally, these fluctuations cancel each other out, on average, and so you just wind up with a tiny zero-point energy that’s positive inherent to space itself. But during inflation, these quantum fluctuations don’t have the opportunity to average out, because space itself is expanding at this exponential rate!
Instead, what happens is that these fluctuations get stretched across the Universe, and so the idea of a quantum fluctuation no longer is restricted to a very small scale. In timescales that are only a tiny fraction-of-a-second long, these quantum effects can get stretched to be fluctuations in energy on stellar, galactic, or even Universe-encompassing scales!
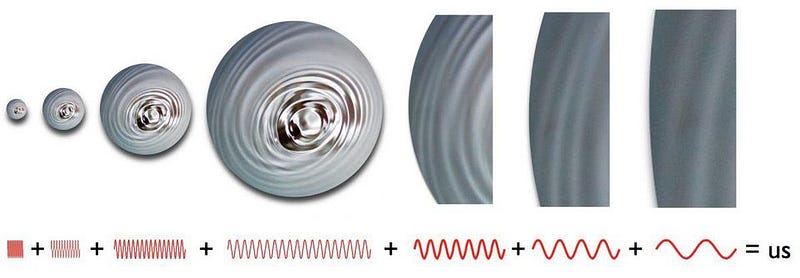
As inflation continues, new quantum-scale fluctuations get created, resulting in additional, smaller-scale fluctuations superimposed atop the larger-scale ones. This goes on and on, creating a pattern of fluctuations, and random regions of all sizes that have overdense and underdense energy densities, for as long as inflation goes on.
Then, after an indeterminate amount of time, inflation comes to an end. And when this occurs, all of that energy inherent to space itself gets converted into matter, antimatter, and radiation. As inflation ends, the hot Big Bang begins, and the Universe becomes filled with stuff.
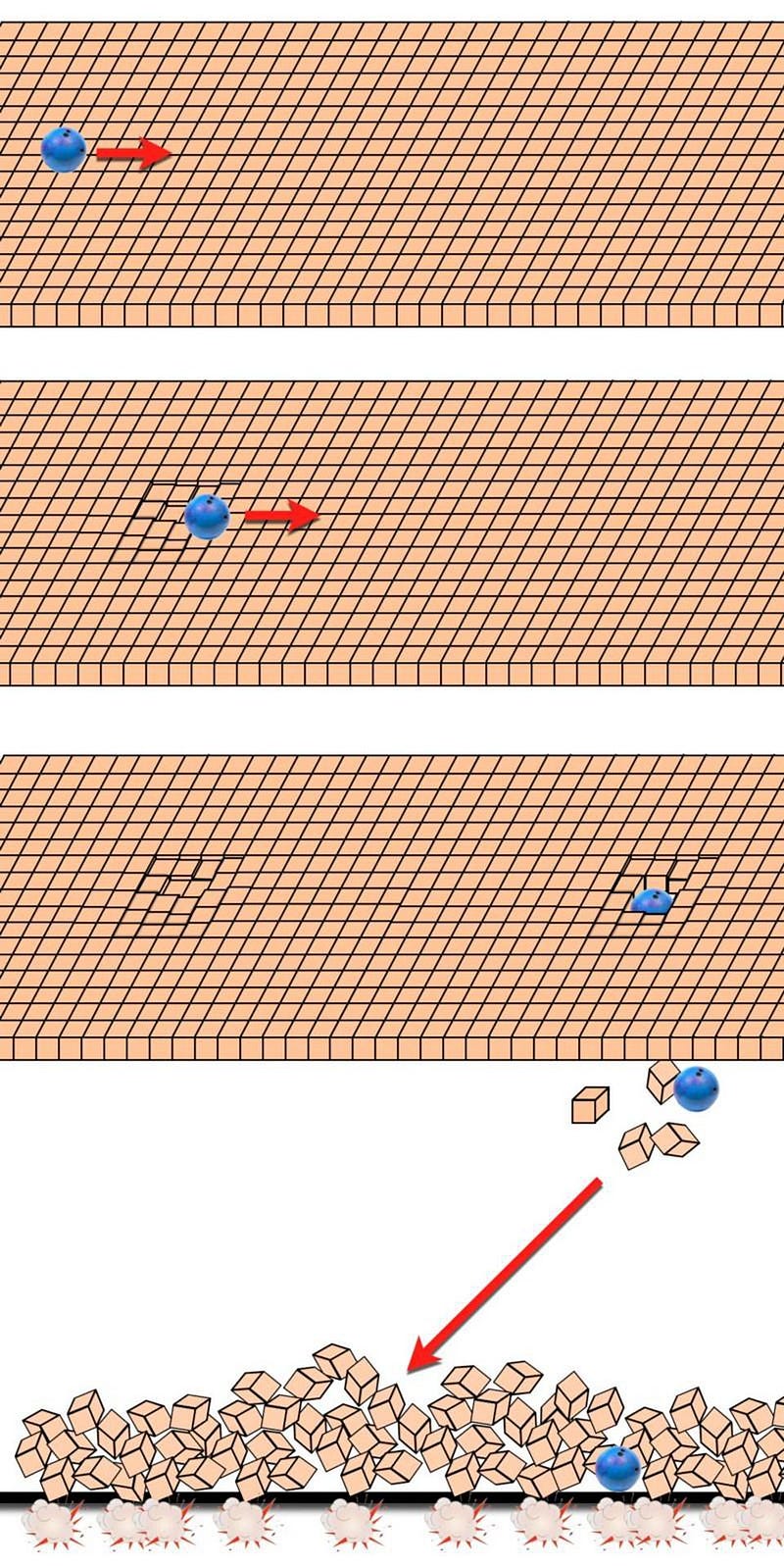
But in the regions that were initially overdense to begin with in terms of energy, due to those quantum fluctuations during inflation, a tiny bit more matter, antimatter, and radiation than average will come to exist in those places. In regions that were underdense, a bit less-than-average matter, antimatter, and radiation will come to exist there. And this spectrum over overdensities and underdensities should result in ever-so-slightly cooler and hotter regions, in terms of temperature, in the Universe as a result.
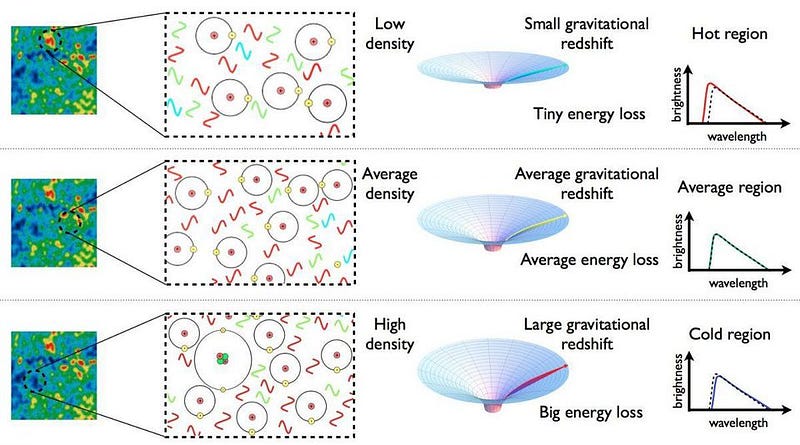
After the Universe has been around for a little while, expanding and cooling, gravitation gets to work. This grows the fluctuations that existed in whatever direction they departed from the average. The slightly hotter regions, being underdense, will more easily give up their matter to denser regions. The colder regions, being overdense, will preferentially attract matter more efficiently than underdense or average-density regions will.
There’s an intricate balance between gravitation, which works to attract everything according to the logic above, and radiation, which presses back against regions that become too dense too quickly. It’s this interplay of forces, between gravitation, radiation, and the initial fluctuations from inflation, that give rise to the bumps, wiggles, and imperfections that we see in the cosmic microwave background.
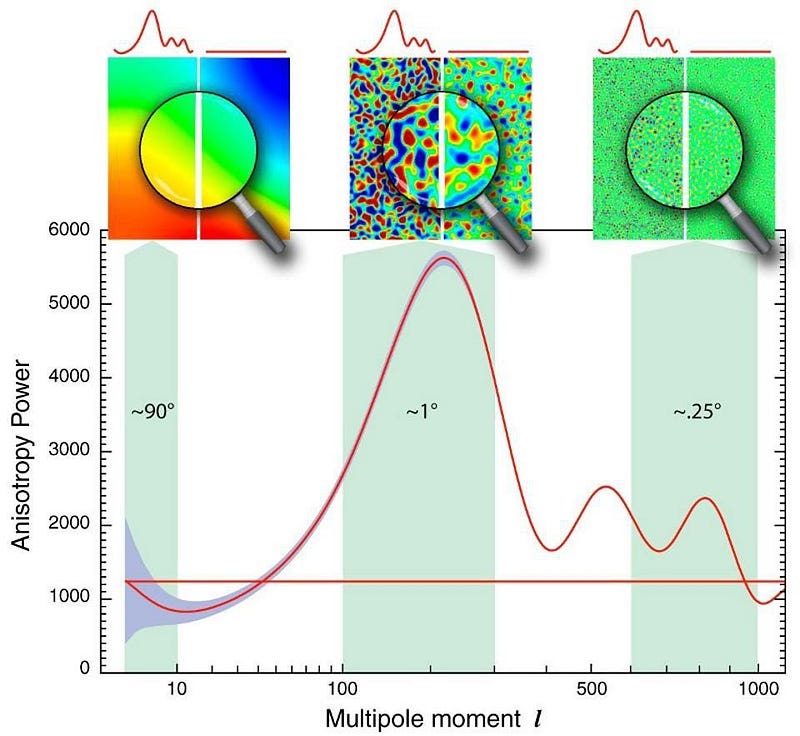
The initial fluctuations, on average, must have had a mean value of 1-part-in-30,000 or so, which is how we arrive at the fluctuations we observe in the Big Bang’s leftover glow. These fluctuations then grow, after the Universe becomes neutral and the radiation stops scattering off of electrons, to produce the large-scale structure we see in the Universe today. Over time, this leads to gravitational growth into stars, galaxies, clusters, and the great cosmic voids separating them.
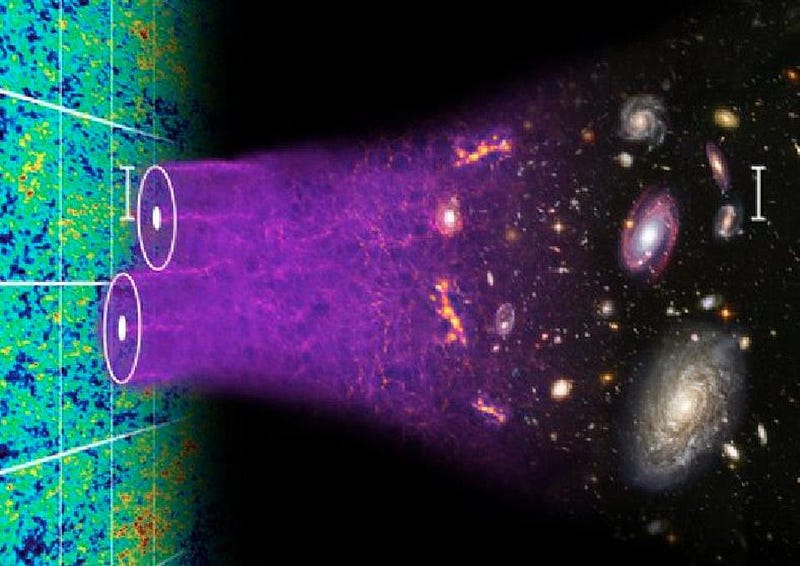
If the Universe were born perfectly smooth, there would be no way to obtain the detailed structure, on both large scales and small ones, that we have today. Our observations require that, somehow, fluctuations of the same magnitude exist on all scales, and that the Universe needed to be born this way. When inflation was first theorized in the late 1970s and early 1980s, there was no way of knowing how these fluctuations would turn out; this was a prediction that inflation made that wouldn’t be verified for decades! Yet the confirmation here is spectacular, as no other theory has a way of generating these fluctuations, and the observations matched what inflation predicted in perfect, incontrovertible fashion as satellites like COBE, WMAP, and most recently, Planck, returned their data.
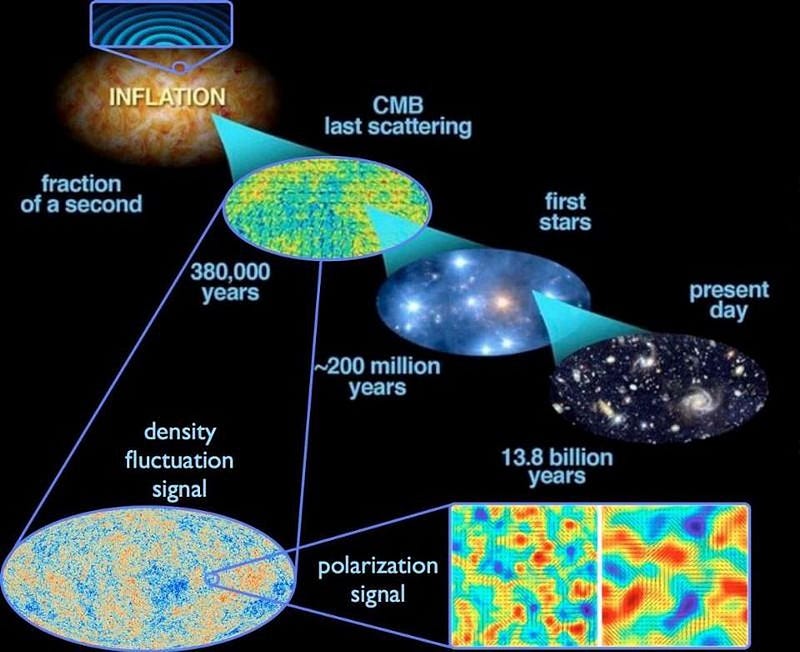
The result is a story so compelling and in agreement with the data that there’s practically no alternative. Inflation isn’t just the thing that happened to set up the Big Bang or solve a slew of problems that we knew beforehand; it made quantitative predictions about what we could expect to exist in the Universe, from early times to modern ones, and observations have confirmed it. Inflation, and its quantum nature, is the reason why the Universe isn’t perfectly smooth today, and that’s a very good thing. Without it, it never would have been possible for us to exist.
Ethan Siegel is the author of Beyond the Galaxy and Treknology. You can pre-order his third book, currently in development: the Encyclopaedia Cosmologica.