Why are there (at most) eight planets in the Solar System?
We all feel bad for Pluto, but it had its demotion coming.
“I have announced this star as a comet, but since it is not accompanied by any nebulosity and, further, since its movement is so slow and rather uniform, it has occurred to me several times that it might be something better than a comet. But I have been careful not to advance this supposition to the public.” –Giuseppe Piazzi
So it begins again: the neverending debate about who gets to be a planet and who doesn’t. Everyone can bring their own interpretation of the science to the table — and everyone has their own preferred naming scheme — but when I think about the Solar System, I try to think about it in the context of all star systems. If science has taught us anything about our planetary system in context with others, the only thing that’s special about this one is that it’s ours.
Believe it or not, as far as we’re able to tell, all stars and star systems have some very important things in common.
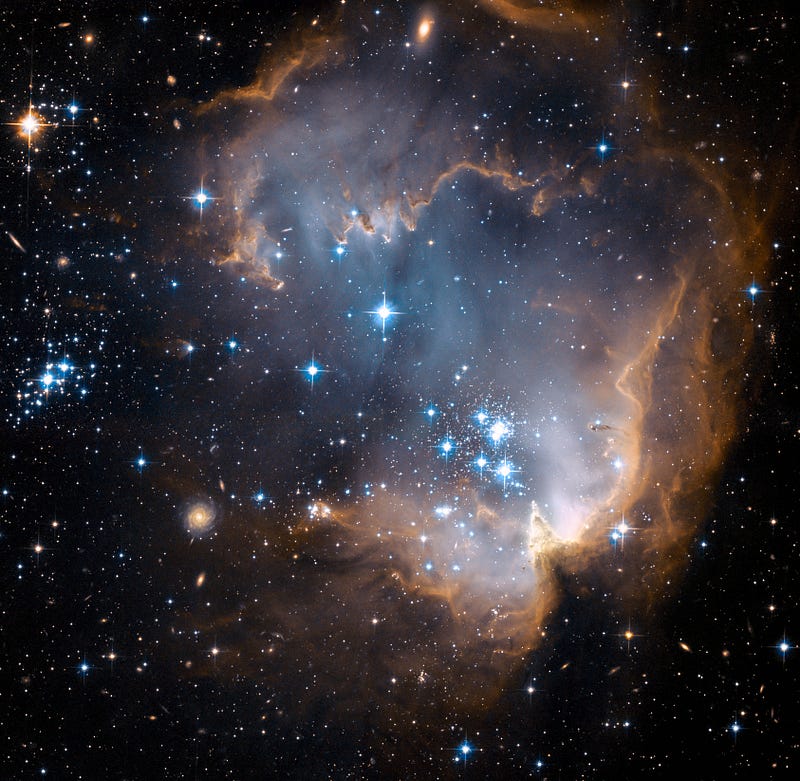
One is that — in the grand scheme of things — all star systems are intimately connected in the sense that no star, as far as we can tell, is ever born in true isolation. Large molecular cloud complexes can hang around for billions of years, but they eventually undergo gravitational collapse. When they do, they form large numbers of stars all at once, anywhere from hundreds to tens of millions of stars in a single cluster! Although the stars that form in these clusters will come in a wide variety of sizes and masses, they all have many similar properties, including the same rough proportion of heavy elements to one another.
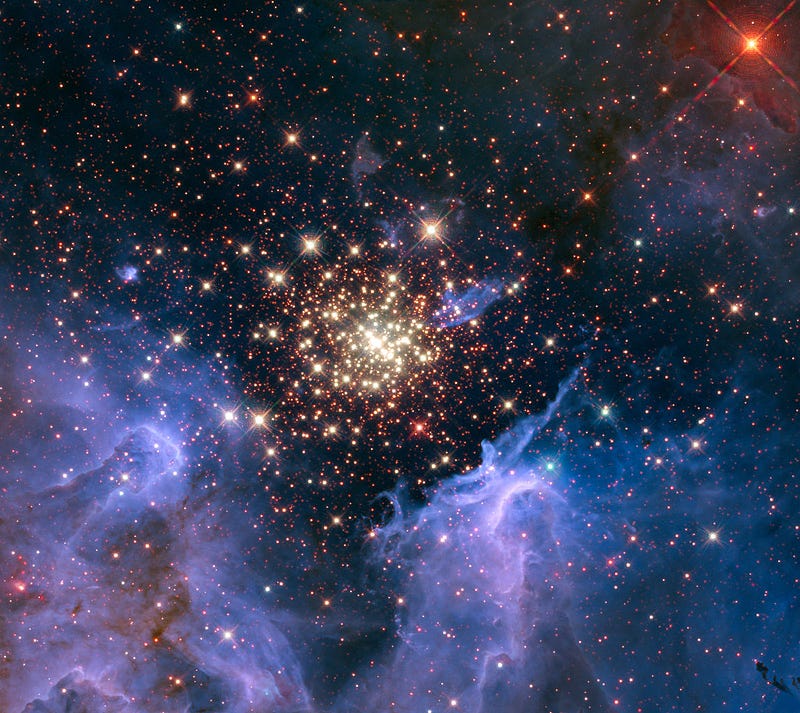
But with the exception of the highest mass, globular star clusters, these large groupings of stars don’t last very long.
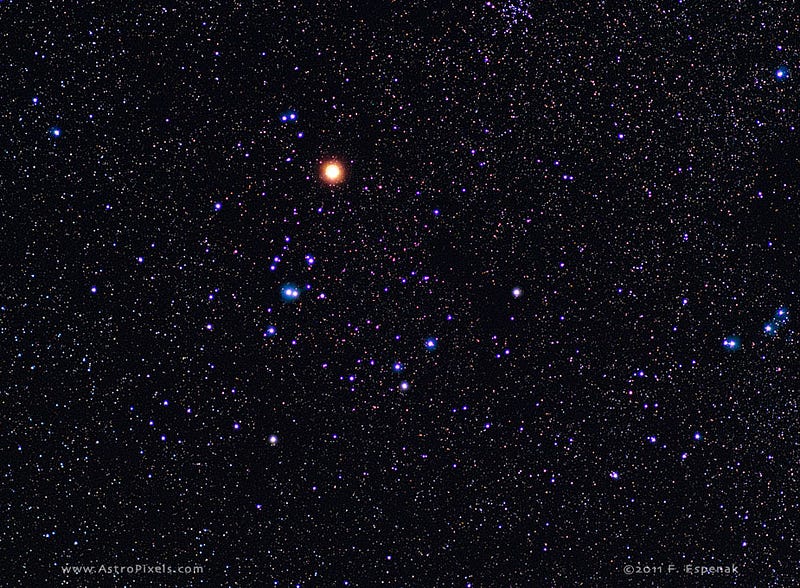
The closest star cluster to us, the Hyades (just 151 light-years away), is in the process of dissociating, where repeated gravitational encounters with (or within) the disk of our galaxy drive the individual stars making a star cluster apart. Our Sun, itself, was very likely once a part of a similar cluster of thousands of stars, born some 4.5 billion years ago in one of our galaxy’s ancient star-forming regions!
But when they do form, these stars aren’t the only things that come about.
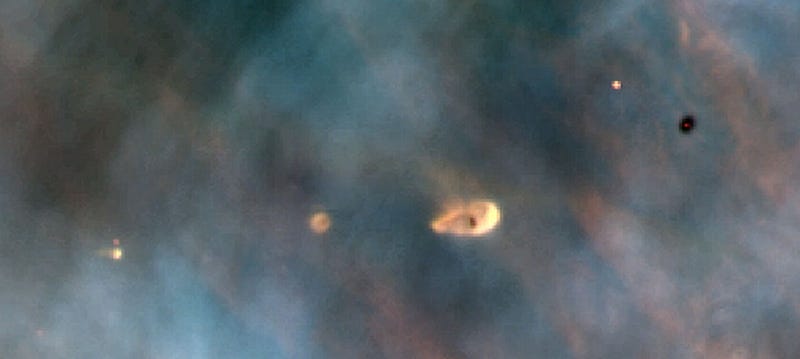
What will eventually become each star, to the best of our knowledge, starts out as a triaxial ellipsoid, undergoes gravitational collapse, forming a star (or stars) near the central region. But as gravity continues to work, all three directions contract, with the shortest axis contracting the fastest and “pancaking”, which means it winds up forming a protoplanetary disk around the central protostar.
The entire complex rotates with some angular momentum, and the protoplanetary disk itself typically lasts for a few million years.
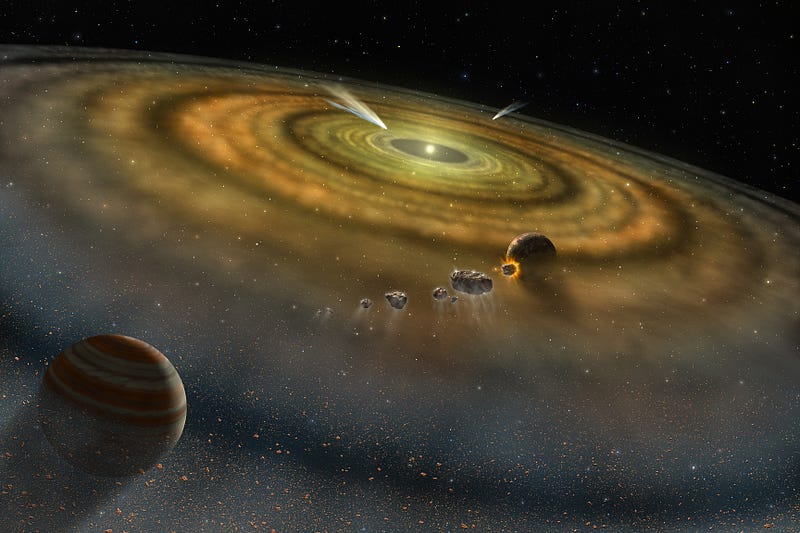
During this time, there are a few physically interesting things fighting for superiority.
- The young star (or stars) are shining brightly, emitting both intense radiation and charged particles, creating not just an inward gravitational force but also an outward flux of energetic particles of both matter and radiation.
- Small gravitational perturbations or instabilities in the disk are racing to grow as large as they can and accrue as much mass as they can before the disk boils away.
- Denser objects — as well as objects with larger mass-to-surface-area ratios — are relatively less affected by the outward flux of the star(s), but are simultaneously subject to resistance (and mass accrual) from the particles they run into.
The net result of all this is that denser and larger bodies tend to migrate inwards, and that the young star system begins to act as though there’s a net buoyant force, pulling the densest objects (and elements) inwards and forcing the less dense objects to the new system’s outskirts.
This might sound like an amazing, unique story, but — at the end of the day — it’s all just straightforward physics, and these are the inevitable consequences of our physical laws.
In addition to what gravitation does, there’s a tremendous temperature gradient around the star(s), where objects very close to the star are said to be inside the Soot Line. In this region, any complex molecules (like polycyclic aromatic hydrocarbons, or PAHs) photodissociate, which means the solar radiation blasts complex molecules apart into simpler ones. Additionally, objects beyond a certain point — the star’s Frost Line — can condense into solid ices, but not inside. It should come as no surprise that Earth is in this hospitable region between the two lines of our Solar System.
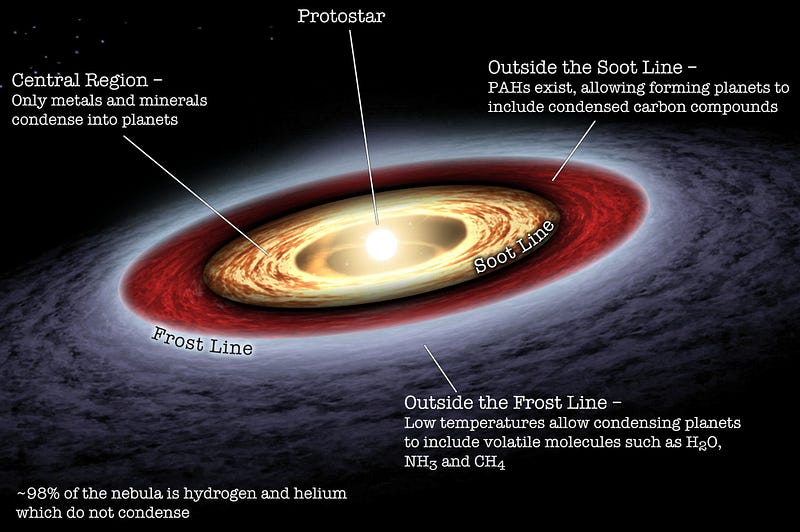
So, with all of that in mind, what is a typical star system — once it’s all grown up — going to look like?
Inside the Frost Line, there can be rocky planets, gas giants, and moons, where the density of these worlds will tend to decrease as we move away from the central star. Beyond that, there will typically be a belt of frozen particles accrued at the Frost Line, exemplified by the asteroid belt in our own Solar System. [And if you were wondering about the quote atop by Giuseppe Piazzi, that was about the very first asteroid ever discovered (by him), but it wasn’t a planet, after all!]
Out beyond the Frost Line, it’s typically only going to be puffy, gas giant worlds (although mini-Neptunes count) that can clear their orbits and exist as planets-as-we-know-them, and finally there will be both a scattered disk and a large, spheroidal cloud of frozen planetesimals, all of much lower density than the inner, rocky worlds.
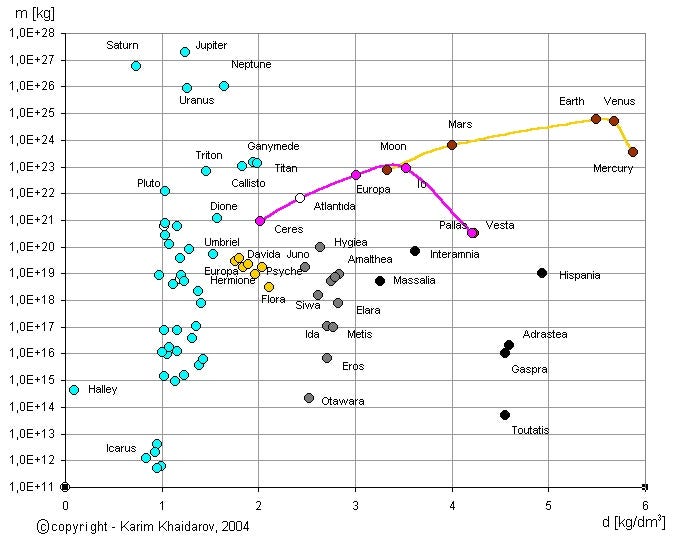
Measurements of the densities of worlds in our own Solar System confirm this picture, as do the first measurements of some exoplanetary systems.
So that’s what pretty much every star system is going to look like: worlds interior to a system’s frost line that can be a mix of rocky planets and gas giants, rock-and-ice asteroids at the frost line (and some denser asteroids that had most of their ices boiled away), gas giants as the only major worlds exterior to the frost line, and mostly-ice-worlds beyond that in a scattered disk and in a spheroidal distribution beyond that.
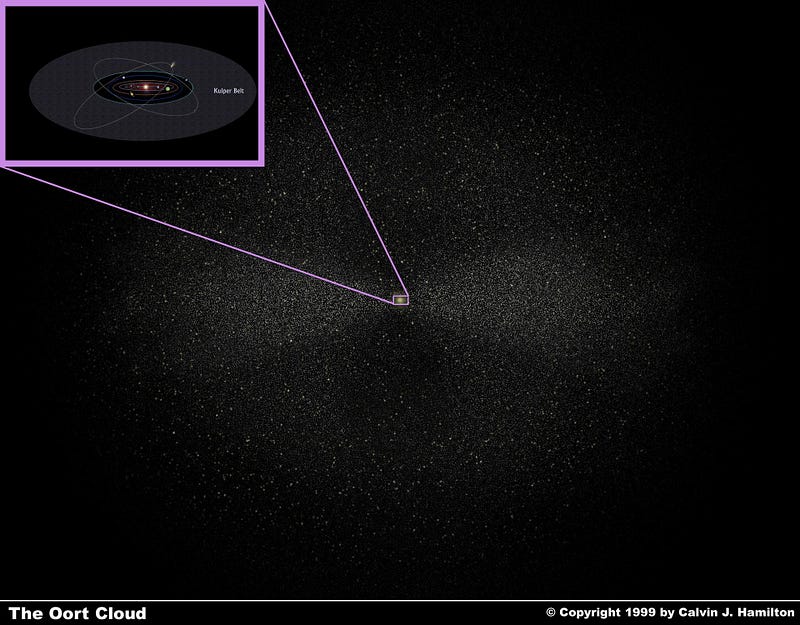
So what does that mean for considering an object a planet in our Solar System, or in our experience in general?
It means that there’s a fundamental difference between the round worlds in hydrostatic equilibrium that have cleared their orbits interior to the frost line and all others, and it means there’s a fundamental difference between the gas giant worlds beyond the frost line and all others. It also means that all the frozen worlds — both the ice-and-rock worlds at the frost line as well as the mostly ice worlds out beyond them — are ubiquitous and super common. Even ones that have enough mass to pull themselves into a sphere!
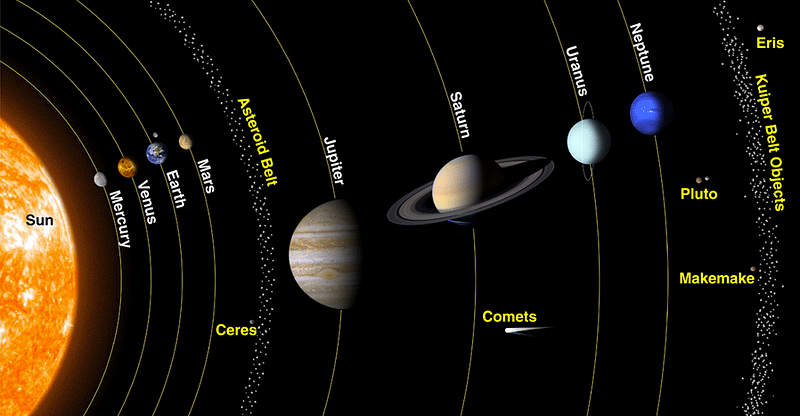
If we make only the worlds (rocky ones as well as gas giants) interior to the frost line planets, we have four planets. If we add in the gas giants beyond the frost line, we’d have four more, for a total of eight. If we decided to add in all the worlds in hydrostatic equilibrium — or with enough gravity to pull themselves into a sphere — we’d have something like an estimated 200 planets. And if we added all the rogue worlds in between our star and the next nearest one, we’d maybe even get into the hundreds of thousands!
Mercury, Venus, Earth and Mars aren’t special only because they’re spheres; they’re special because of where they are and what their formation history is! They’re special because of their densities, temperatures, atmospheres (or lack thereof, right Mercury?) and locations.
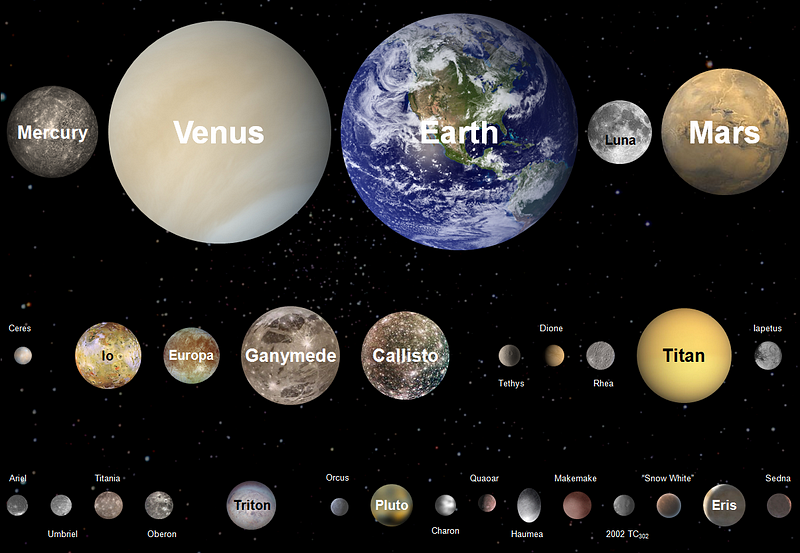
The largest of the asteroids and Kuiper Belt Objects, as well as the giant moons of Jupiter, Saturn and Neptune are interesting, but not in the same ways that true planets are.
If I had my way, that’s what I’d teach everyone about the Solar System, and that’s why I think that eight planets is just the right number for ours. You may (and many of you, I’m sure, will) disagree, but this knowledge and understanding is part of the driving force behind Pluto’s 2006 “demotion” from planetary status, not some vendetta against the cold, icy worlds of the Kuiper Belt, Oort Cloud and other locations beyond our Solar System’s frost line.
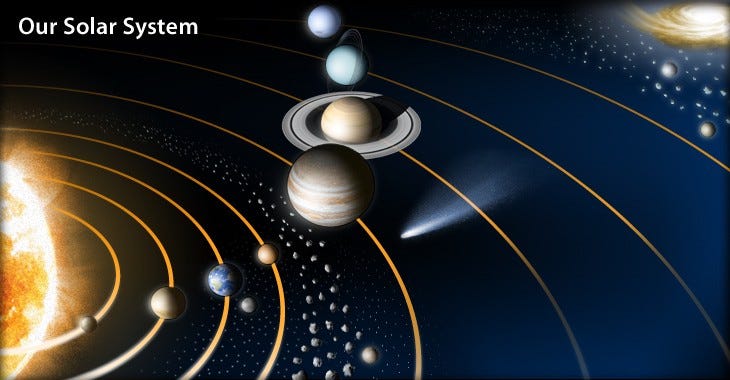
Our eight planets are all special, and all the gas giants and rocky-worlds-interior-to-a-frost-line are special in exactly the same orbit-clearing way. The asteroids, Kuiper Belt objects and Oort Cloud objects may be special in their own way as well, but it’s a decidedly different way than these worlds that we presently call planets are.
So remember that the next time you argue about what is-or-isn’t a planet; this is how the Universe really works, and everything else is just a name!
An earlier version of this post originally appeared at Scienceblogs. Head on over there to the Starts With A Bang forum and weigh in!