What’s the third most common element?
The Universe was 99.999999% Hydrogen and Helium after the Big Bang. Billions of years later, there’s a new contender in town.
“When it comes to atoms, language can be used only as in poetry. The poet, too, is not nearly so concerned with describing facts as with creating images.” –Niels Bohr
One of the most remarkable facts of existence is that everything material we’ve ever touched, seen, or interacted with is made up of the same two things: atomic nuclei, which are positively charged, and electrons, which are negatively charged. The way these atoms interact with each other — the ways they push-and-pull against each other, bond together and create new, stable energy states — is literally responsible for the world around us.
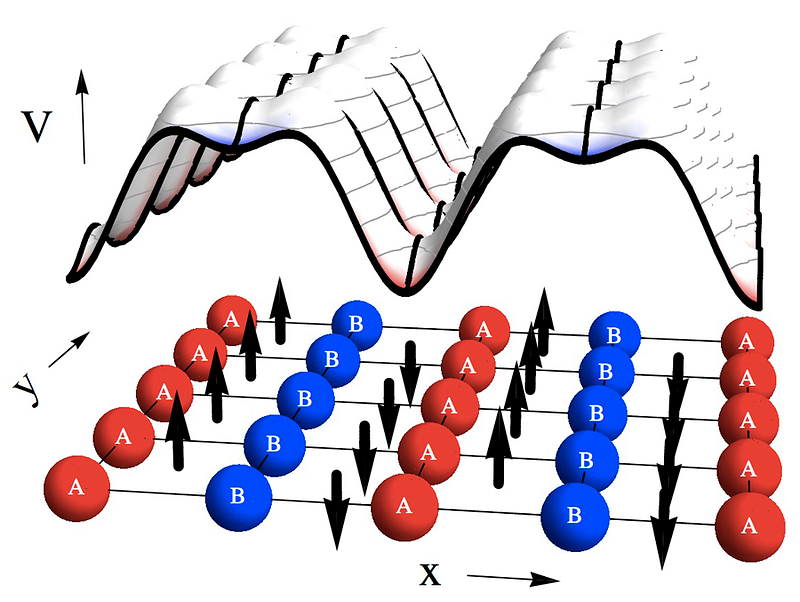
While it’s the quantum and electromagnetic properties of these atoms that enable our Universe to exist exactly as it is, it’s important to realize that the Universe didn’t start out with all the ingredients necessary to create what we know today. In order to achieve these various bond structures, in order to build complex molecules which make up the building blocks of all we perceive, we needed a huge variety of atoms. Not just a large number, mind you, but atoms that show a great diversity in type, or in the number of protons present in their atomic nucleus.
Our very bodies themselves require elements like carbon, nitrogen, oxygen, phosphorous, calcium and iron, none of which existed when the Universe was first created. Our Earth itself requires silicon and a myriad of other heavy elements, going all the way up the periodic table to the heaviest naturally occurring ones we find: Uranium and even trace amounts of Plutonium.
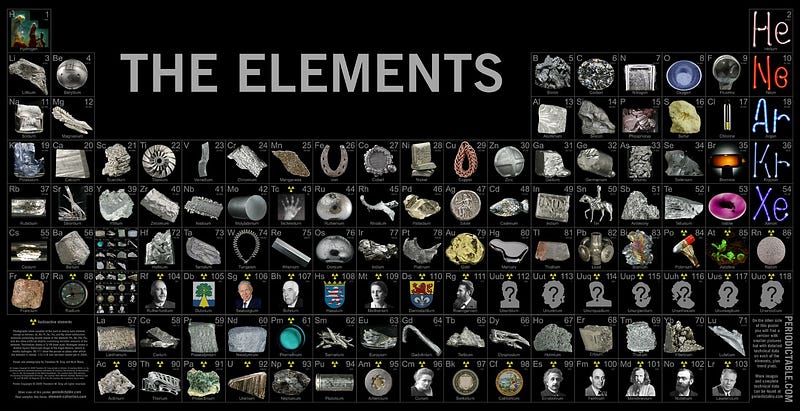
In fact, all the worlds in our Solar System show signs of these heavy elements in the periodic table, with some ~90 or so found before humans started creating ones that don’t occur without our intervention. Yet back in the very early stages of the Universe — before humans, before there was life, before there was our Solar System, before there were rocky planets or even the very first stars — all we had was a hot, ionized sea of protons, neutrons and electrons.
This young, ultra-energetic Universe was expanding and cooling, and eventually reached the point where you could fuse protons and neutrons without them immediately being blasted apart.
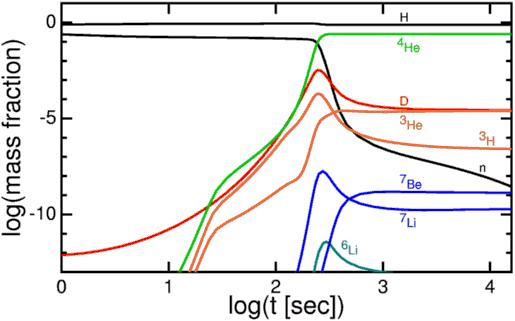
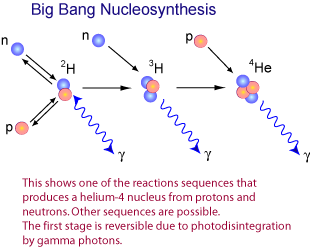
After a chain reaction, we wound up with a Universe that was — by number of nuclei — about 92% hydrogen, 8% helium, about 0.00000001% lithium, and maybe 10^-19 parts beryllium.
That’s it.
In order to cool enough to form deuterium, the first (but precarious) step in the chain reaction to build heavier elements, the Universe has to cool a lot. By time it gets to those (relatively) low temperatures and densities, you can’t build anything heavier than helium except in tiny, trace amounts. For a brief time, then, lithium, the third element in the periodic table, is the third most common element in the Universe.
Pathetic! But once you start forming stars, all of that changes.
The moment the first star is born, some 50-to-100 million years after the Big Bang, copious amounts of hydrogen start fusing into helium. But even more importantly, the most massive stars (the ones more than about 8 times as massive as our Sun) burn through that fuel very quickly, in just a few million years themselves. Once they run out of hydrogen in their cores, that helium core contracts down and starts fusing three helium nuclei into carbon! It only takes approximately a trillion of these heavy stars existing in the entire Universe for lithium to be defeated.
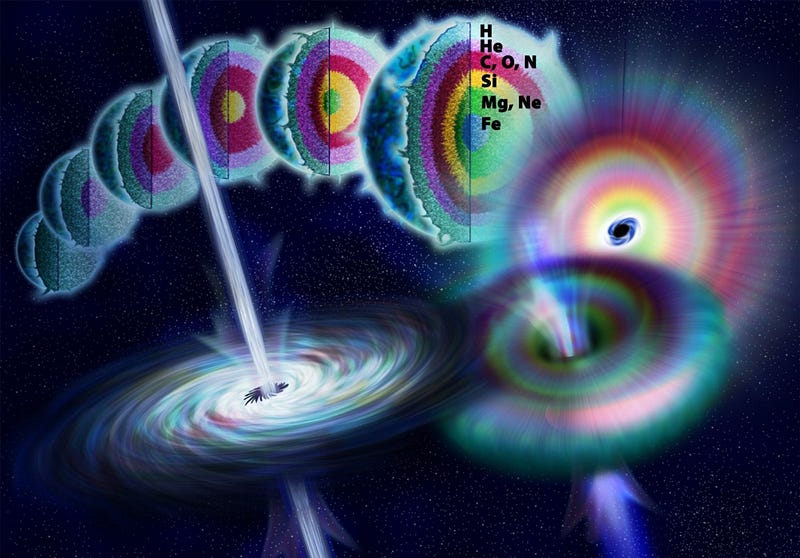
But will it be carbon that breaks the record? You might think so, since stars fuse elements in onion-like layers. Helium fuses into carbon, then at higher temperatures (and later times), carbon fuses into oxygen, oxygen fuses into silicon and sulphur, and silicon finally fuses into iron. At the very end of the chain, iron can fuse into nothing else, so the core implodes and the star goes supernova.

This enriches the Universe with all the outer layers of the star, including the return of hydrogen, helium, carbon, oxygen, silicon, and all the elements formed through the other processes:
- slow neutron capture (the s-process), building elements up sequentially,
- the fusion of helium nuclei with heavier elements (creating neon, magnesium, argon, calcium, and so on), and
- fast neutron capture (the r-process), creating elements all the way up to uranium and even beyond.
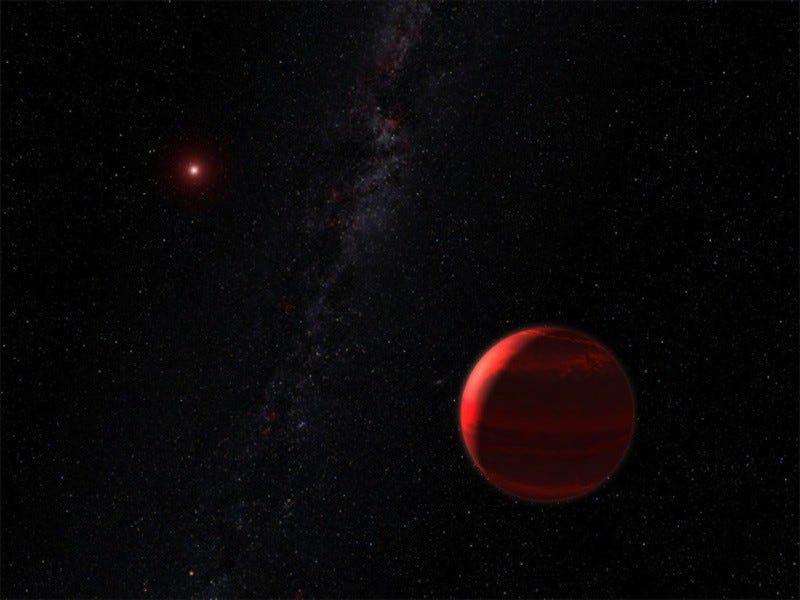
Over many generations of stars, this process repeats itself, except this time it starts with the enriched ingredients. Instead of simply fusing hydrogen into helium, massive stars fuse hydrogen in what’s known as the C-N-O cycle, leveling out the amounts of carbon and oxygen (with somewhat less nitrogen) over time.
When stars undergo helium fusion to create carbon, it’s very easy to get an extra helium atom in there to form oxygen (and to even add another helium to the oxygen to form neon), something even our paltry Sun will do during the red giant phase.
And when a star is massive enough to begin burning carbon into oxygen, that process goes almost to full completion, creating significantly more oxygen than there was carbon.
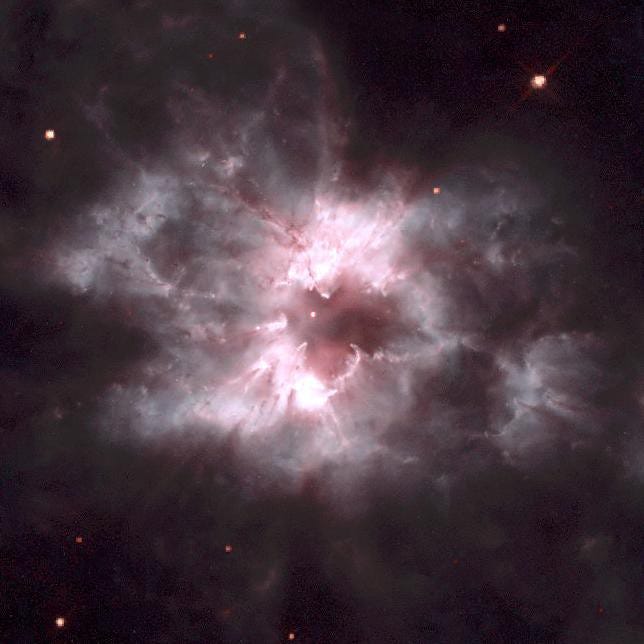
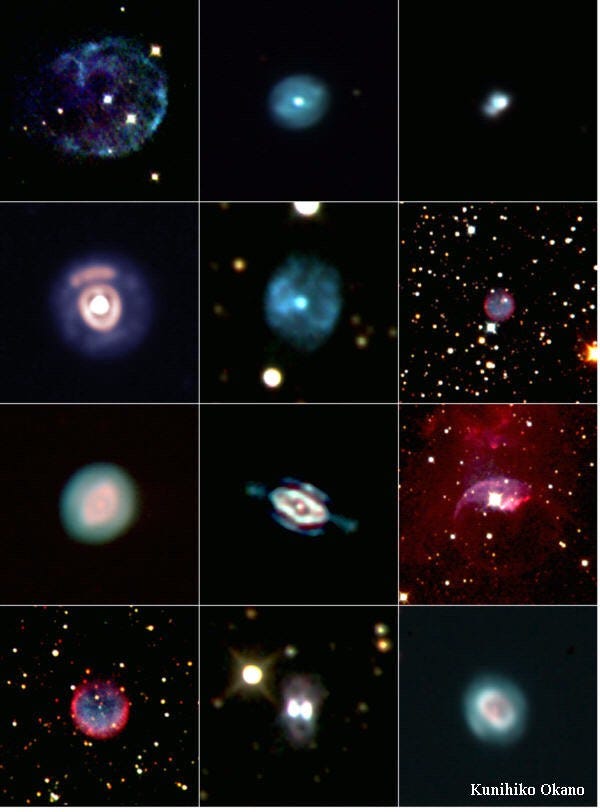
When we look at supernova remnants and planetary nebulae — the remnants of very massive stars and sun-like stars, respectively — we find that oxygen outmasses and outnumbers carbon in all cases. We also find that none of the other, heavier elements come close!
These three processes, combined with the lifetime of the Universe and the duration that stars have been living teaches us that oxygen is the third most abundant element in the Universe. But it’s still far behind both helium and hydrogen. (Don’t be fooled by optical illusions, either; iron is no higher than silicon in the graph below!)
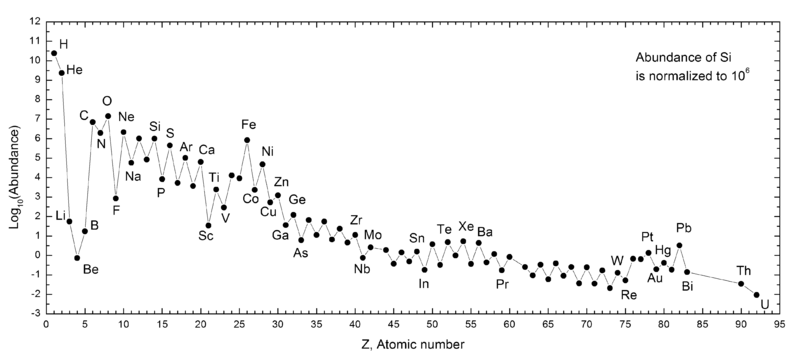
Over long enough time periods, periods that are at least thousands (and probably more like millions) of times the present age of the Universe, helium might finally overtake hydrogen as the most abundant element, as fusion may eventually run to some sort of completion. As we go to extraordinary long timescales, the matter that doesn’t get ejected from our galaxy may wind up fusing together, over and over, so that carbon and oxygen might wind up someday surpassing even helium; one never knows, although simulations indicate this is possible.
At the present, here’s where each of the individual elements primarily come from.
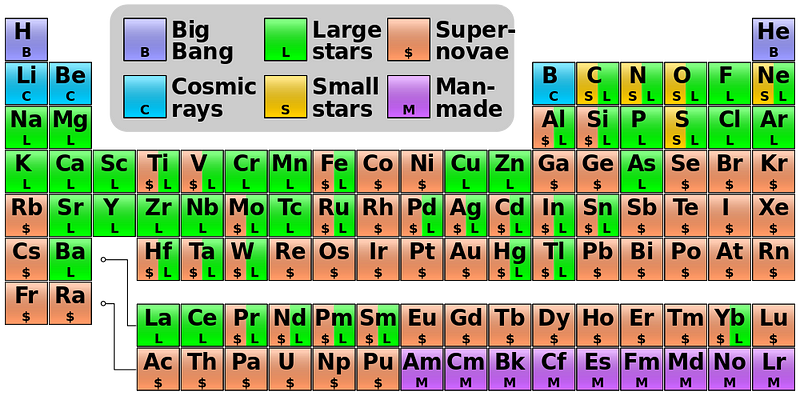
So stick around, because the Universe is still changing! Oxygen is the third most abundant element in the Universe today, and in the very, very far future, may even have the opportunity to rise further as hydrogen (and then possibly helium) falls from its perch. Every time you breathe in and feel satisfied, thank all the stars that lived before us: they’re the only reason we have oxygen at all!
Leave your comments at our forum, and support Starts With A Bang on Patreon!