What Will It Be Like When We Reach The End Of The Universe?
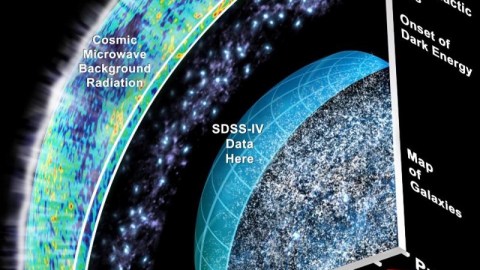
There’s a million things we haven’t done. But just you wait.
The Universe as we know it began some 13.8 billion years ago with the onset of the hot Big Bang. Ever since that early stage, our cosmos has been expanding, cooling, and gravitating in accordance with the laws of physics. As the Universe unfolded, we passed a series of important milestones that led to the Universe we observe and inhabit today. After 13.8 billion years, on one world in an outer arm of a non-descript galaxy on the outskirts of our local supercluster, human beings emerged.
It’s been spectacular how we’ve managed to put together our entire cosmic history, from what set up and caused the Big Bang until the present day. But that leads to one spectacular question that humanity has long wondered about: what is our ultimate fate? What will it be like when we reach the end of the Universe? After countless generations of searching, we’re closer than ever to the answer.
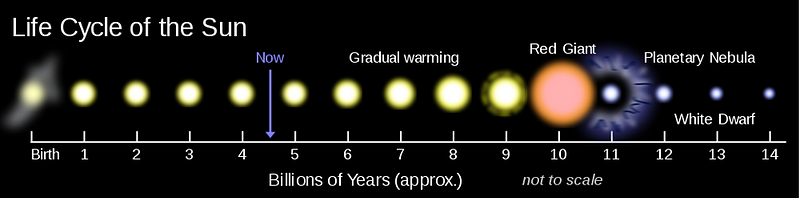
On a local scale, we have our planet orbiting the Sun as one component of our Solar System. But on long timescales, things get exciting relatively quickly. The Sun, as it burns through the nuclear fuel in its core, slowly heats up and becomes more luminous: over the 4.5 billion years that our Solar System has been around, the Sun has increased its energy output by about 20–25%.
In another one or two billion years, the Sun’s temperature will increase by a great enough amount that Earth will heat up so severely that our planet’s oceans will boil. This will effectively end all life on Earth (at least, as we know it) at that time, bringing an end to whatever lives our surviving descendants and our evolutionary cousins continue to enjoy. But the demise of our planet will likely go unnoticed by the cosmos.
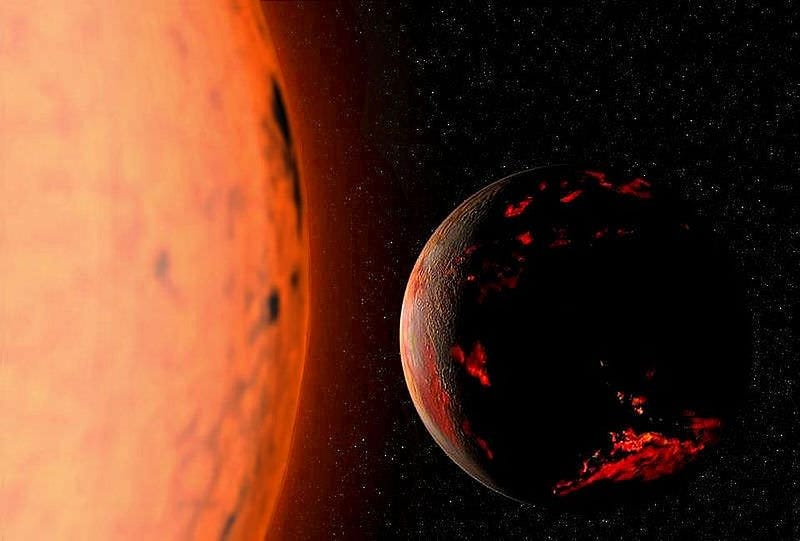
Sure, there are grander things to think about. As the Universe ages, the rate of star formation continues to plummet. The number of new stars we’re forming right now is just a few percent (perhaps 3–5%) of what it was at its peak, some 11 billion years ago. Star formation reached a maximum some ~3 billion years after the Big Bang, and has been falling ever since. To the best of our understanding, most of the stars that will ever exist in the Universe have already been created.
And while galaxies will continue to grow by both funneling in new matter from the intergalactic medium and by joining and merging together, most of the structures we’re ever going to form have already been formed. Our Local Group of galaxies might all eventually merge together into one giant elliptical galaxy — Milkdromeda, which will primarily form in 4-to-7 billion years when the Milky Way and Andromeda collide — the larger-scale structures aren’t really getting any bigger.
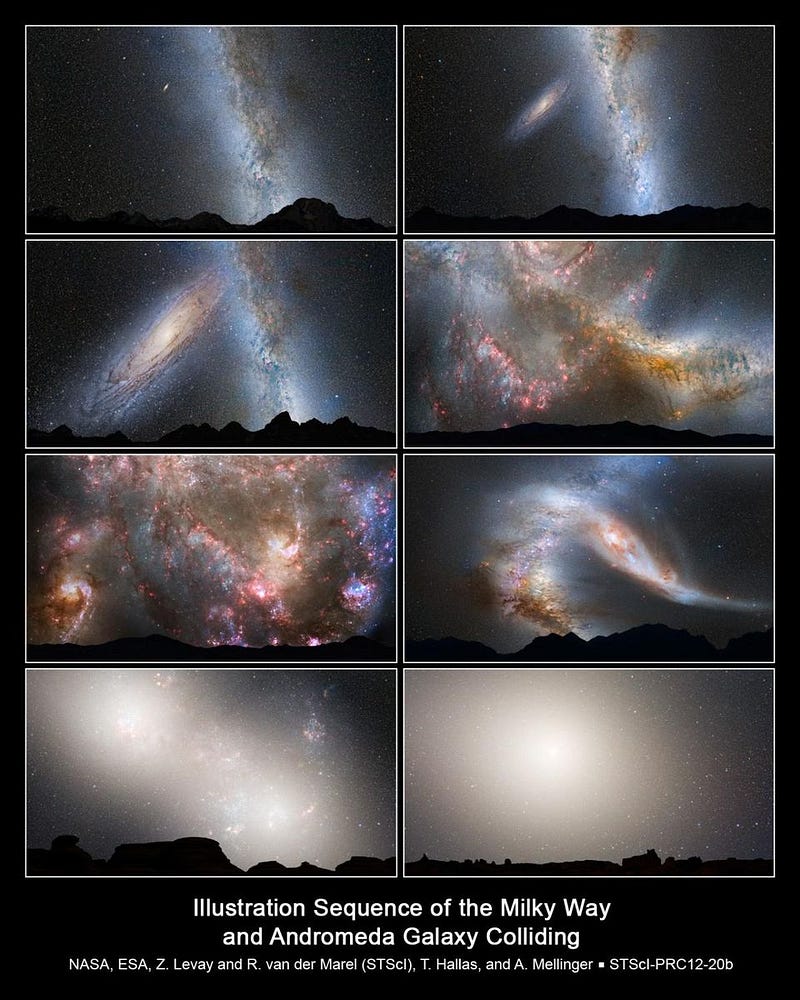
Yes, the Local Group is relatively small potatoes on a cosmic scale. With two or three (if you include Triangulum) large galaxies alongside perhaps 60 small ones, the Local Group is notable only because it’s our home. In reality, groups and clusters of galaxies with dozens, hundreds, or even thousands of times the mass of our Local Group are common across the Universe. The Virgo Cluster, just 50–60 million light-years away, is approximately 1,000 times as massive as our Local Group is.
For a long time, we didn’t know whether we were gravitationally bound to an even larger structure that included the Virgo Cluster; it was sometimes assumed that we were and it was called the Local Supercluster. Ironically, even though we now have a name for this larger structure — Laniakea — it turns out that there is no such thing as this “supercluster”-scale structure. The reason has to do with the fate of the entire Universe.
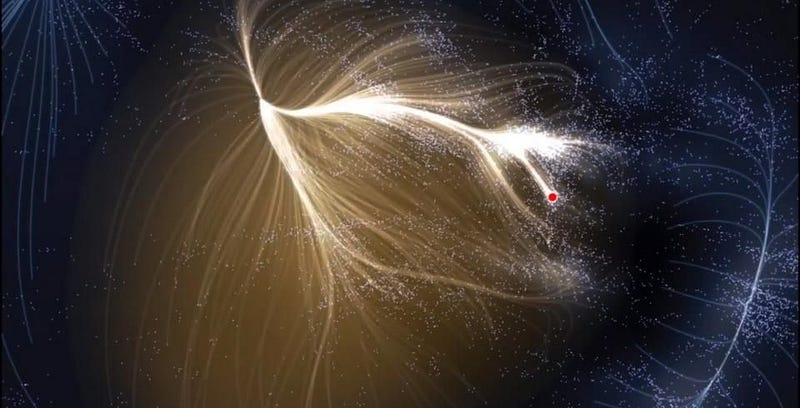
If you had gone to an astrophysicist in the 1960s, shortly after the Big Bang had been revealed as the source of our cosmic origins, you could have asked them a simple question, “what will the fate of our Universe be?” In the context of the Big Bang and Einstein’s General Relativity, there’s a simple and straightforward relationship between three things: the expansion rate of the Universe, the total amount and type of stuff inside it, and our fate.
You can imagine this as a cosmic race between two players: the initial expansion and the total gravitational effects of everything in the Universe. The Big Bang is the starting gun, and as soon as that gun goes off — as the astrophysicists would have told you — there are three possible outcomes.
- Recollapse. The expansion starts off fast, but there’s enough matter and energy for gravitation to successfully overcome it. The expansion slows, the Universe reaches a maximum size, and recollapses, ending in a Big Crunch.
- Expansion forever. The expansion starts off fast, and there isn’t enough matter and energy to overcome that initial expansion. The expansion rate drops but never reaches zero; the Universe expands forever and ends in a Big Freeze.
- The “Goldilocks” case. Right on the border between expansion forever and recollapse, this is the critical case. One more proton in the Universe would lead to recollapse, but it isn’t there. The expansion asymptotes to zero, but never reverses.
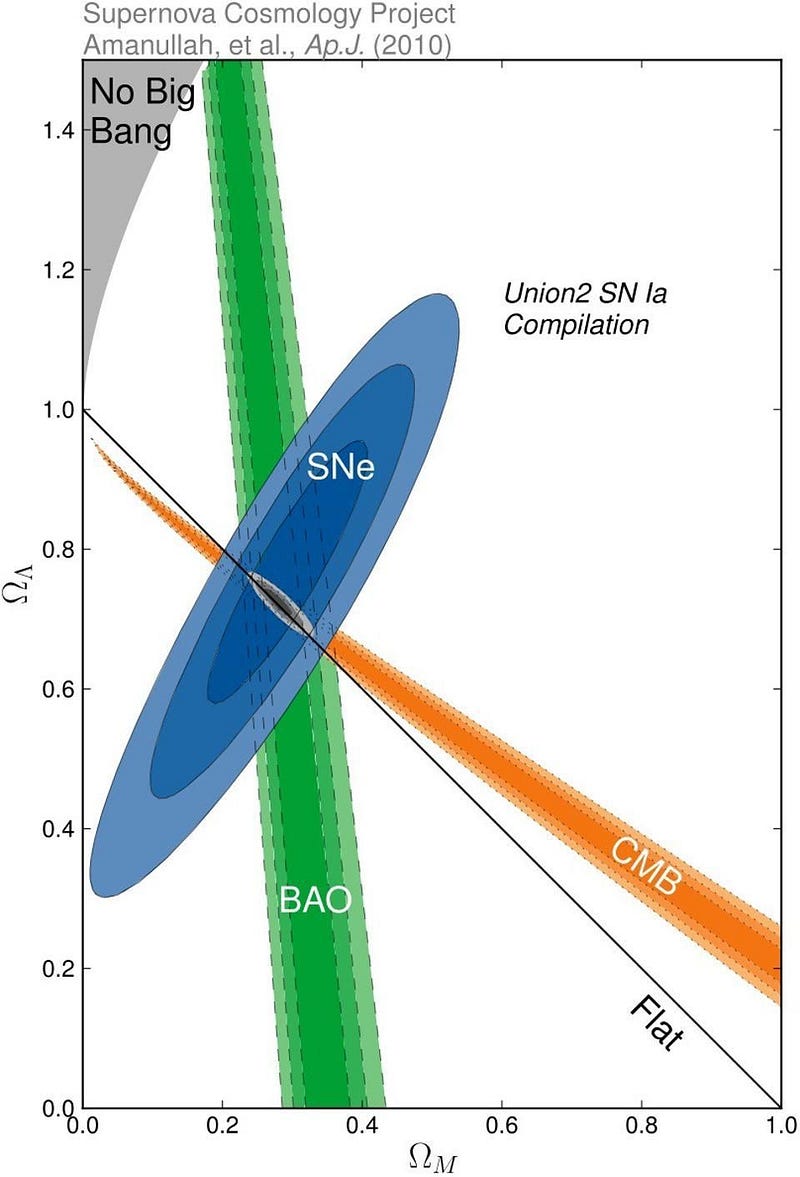
For decades, the big quest of the scientific field of cosmology — itself a sub-discipline of astrophysics — was to measure these quantities: how fast the Universe is expanding today and how the expansion rate has been changing over the Universe’s history. It’s often said, about General Relativity, that “matter tells space how to curve; that curved space tells matter how to move.”
Well, for the expanding Universe, the expansion tells light how to redshift, and the redshifted light reveals the expansion history of the Universe. Because of the link between spacetime and matter/energy, measuring how the Universe has expanded over its history has the capacity to reveal exactly what the Universe is made of: what the different types of energy in it are and how they compel the Universe to expand.
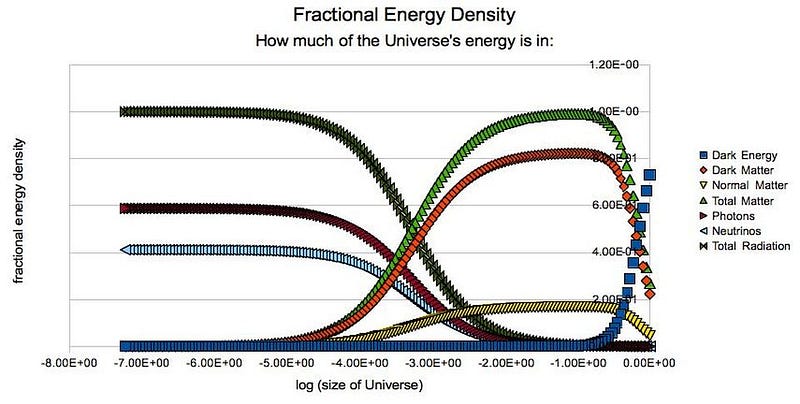
What’s remarkable about the past three decades or so is that we’ve been able to gather enough observations to a high-enough precision that what was once a question for philosophers and theologians — imagining what will happen when we reach the end of the Universe — has now been answered scientifically. Of the three fates we once imagined we now know something remarkable: they’re all incorrect. Instead, the Universe surprised us when the answer came in to the questions of what it’s made of and what its fate will be.
We’re not dominated by matter, radiation, or by spatial curvature. Instead, the greatest component of our Universe is dark energy, which will not only cause our Universe to keep expanding, but for the speed of these receding galaxies to increase without limit. Our Universe isn’t just expanding, but accelerating: these galaxies will recede faster and faster until they’re pushed so far away that we’ll never be able to reach them.
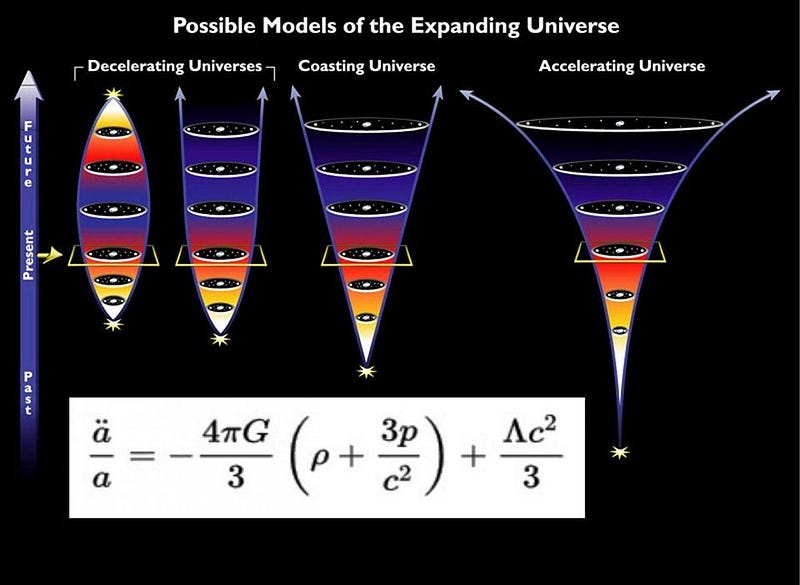
What does this mean for the fate of our Universe? On the one hand, there are a lot of things we already know. We know that the expansion has been accelerating for some 6 billion years, and that dark energy has dominated the Universe for the entire history of planet Earth. We know that the largest structures that are bound together today — galaxies, galaxy groups, and galaxy clusters — are the largest structures that will ever form; would-be structures on larger scales are being driven apart by this accelerated expansion.
And even though everything we see is consistent with dark energy being a cosmological constant, with the same energy density everywhere in space and throughout time, we cannot be certain. Dark energy could still evolve, leading to a Universe that might either recollapse in a Big Crunch, expand forever, or speed up in its acceleration and eventually tear even the fabric of space apart in a catastrophic Big Rip.
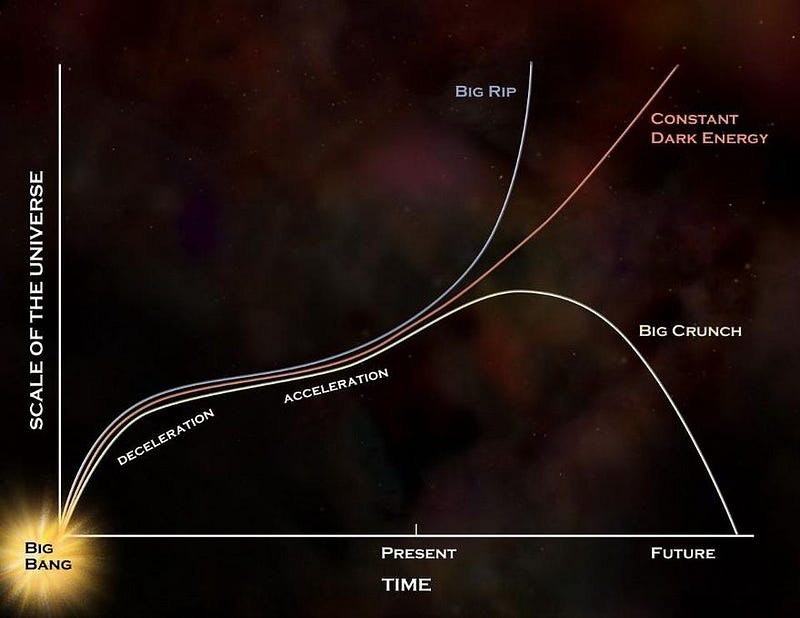
Right now is a critical time for cosmology, as the coming new generation of space-based and ground-based observatories should help us reveal the answers to these burning questions. Will our Universe continue to expand and accelerate forever? Is dark energy truly a constant in both space and in time? Or does dark energy evolve in some fashion? Is it smooth or inhomogenous? And what, if anything, does that mean for the fate of the Universe?
Astrophysicist Dr. Katie Mack, who’s making a career out of the attempt to answer this ultimate question (and has a new book coming out on exactly this topic), will be delivering a public lecture in a very special interview-like format this Wednesday, May 6, at 7 PM ET / 4 PM PT, courtesy of the Perimeter Institute. You can watch it, either live or anytime after the lecture is complete, simply by clicking on the embedded video below.
If dark energy truly is a constant, then we already know how our Universe will end. It will expand forever; the galaxies within groups and clusters will merge together to form a giant super-galaxy; the individual super-galaxies will accelerate away from one another; the stars will all die or get sucked into supermassive black holes; and then the stellar corpses will get ejected while the black holes decay away. It might take googols of years, but eventually, the Universe will be cold, dead, and empty.
But this is not the only possibility, as Dr. Katie Mack will help us explore. Join us when the talk occurs in real-time for a live-blog extravaganza (below), or come back any time after it’s over to watch the talk in its entirety with the full live-blog presented below. It’s your Universe, too. Don’t you want to know how the story ends?
Live blog begins at 6:50 PM ET/3:50 PM PT; all timestamps below are on Pacific time.
3:50 PM: Welcome, everyone, as we get ready for the live show to begin! When you think about the far future of the Universe, you probably think about the Earth and the Sun and our solar system ending its life. You probably think about stellar death, the formation of a planetary nebula and a white dwarf, and Mercury, Venus, and maybe even the Earth getting engulfed.
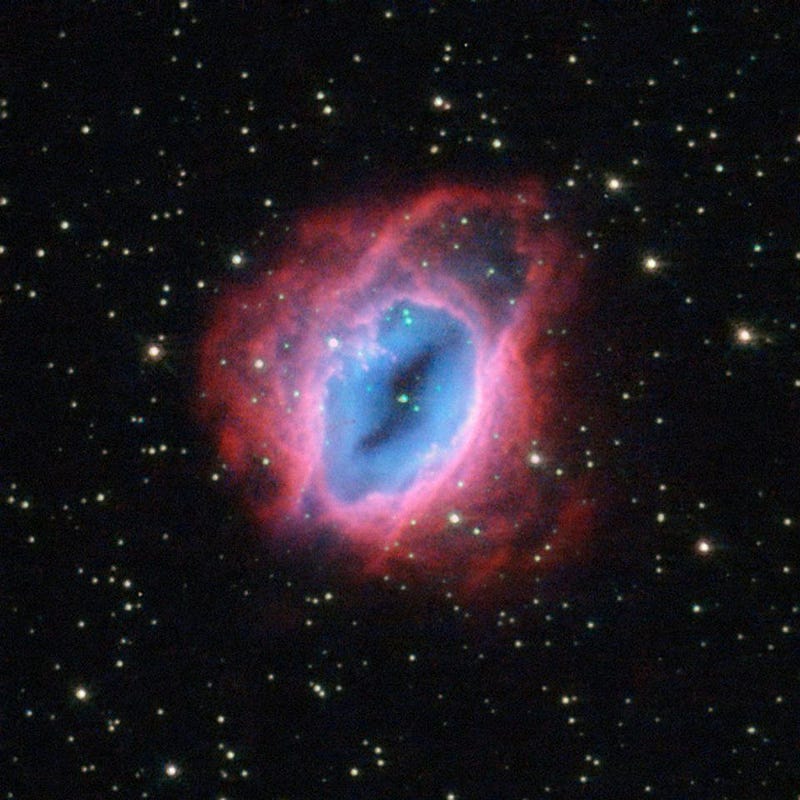
It’s a fascinating thing to consider on what’s generally viewed as a “small” cosmic scale. But what about the larger ones?
3:53 PM: As we look to larger scales, we’ll find that galaxies merge together and give off bursts of star formation. We’ll find that individual galaxies will lose and eventually run out of gas, and that star formation will drop lower and lower, eventually forming just a few rare stars every few millennia in each galaxy.
It’s a slow death for even the largest bound structures in the Universe: massive galaxies and massive galaxy clusters.
But on larger scales than that, these enormous structures are all escaping from one another’s reach.
3:56 PM: This is because the expansion of the Universe is not just relentless, but there’s a special type of energy that appears to be inherent to space itself: dark energy. We initially thought there would be no reason for this “cosmological constant” to be non-zero, and that if it was non-zero, there was no reason for it to be positive. And yet, when the observations came in, that’s what they pointed to.
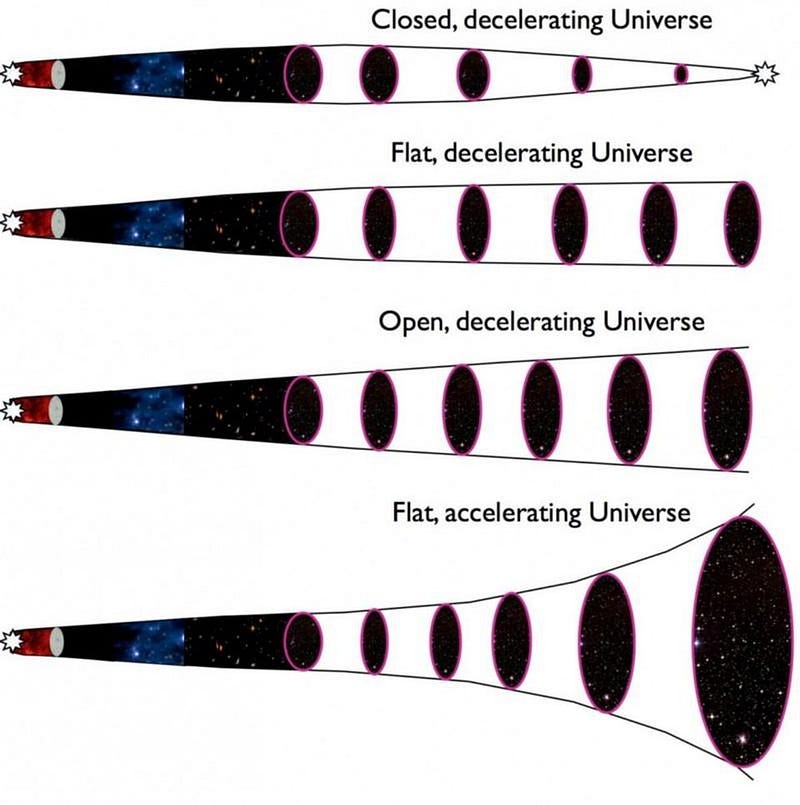
Then that leads to a new question: is dark energy truly a constant? Is it really going to remain constant forever and ever?
Or will it increase in strength? Will it weaken and decay to zero? Will it reverse sign?
Is it the same everywhere in space, and every “when” in time? Or does it vary?
And what does that mean for our ultimate fate?
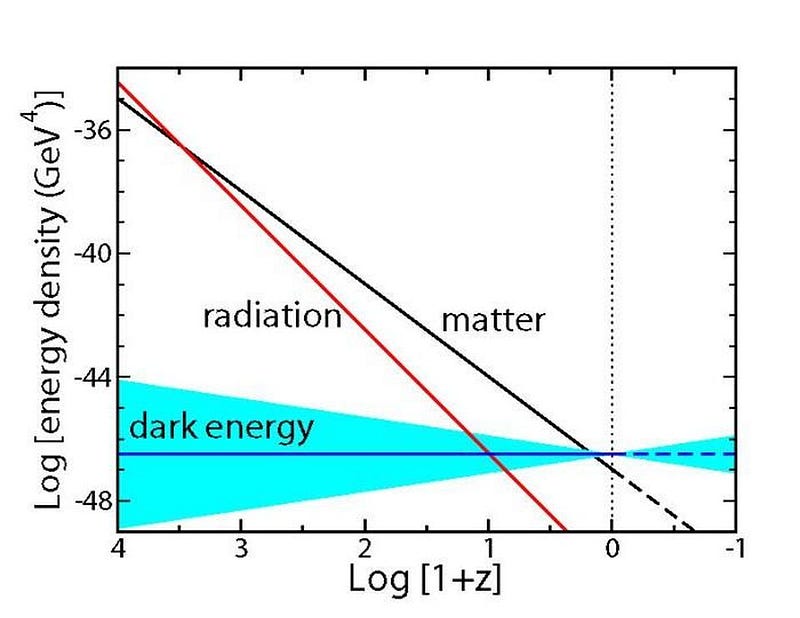
3:59 PM: Before the lecture starts, I’ll note that nobody knows this, but also that despite all the possibilities that are talked about in the literature, there is no good, compelling theoretical reason for dark energy to be anything other than a constant in both space and time.
Moreover, there is no compelling observational evidence, not from any of the strange ways of looking at the Universe we’ve developed, that the Universe is expanding in any way other than with dark energy as a cosmological constant. When I was a grad student, dark energy was known to about a 30% uncertainty to be a constant; that’s down to about 7% now, and with telescopes like Euclid, WFIRST and the LSST, that should get down to about 1–2%. This decade is really the last chance for non-standard dark energy to show up!
4:00 PM: And now, at last, right on time, we get to see what the first post-COVID-19 Perimeter Institute public lecture looks like!
4:02 PM: And the audience is looking good: there are nearly 500 people online watching right at the very moment. Good job, Perimeter Institute!
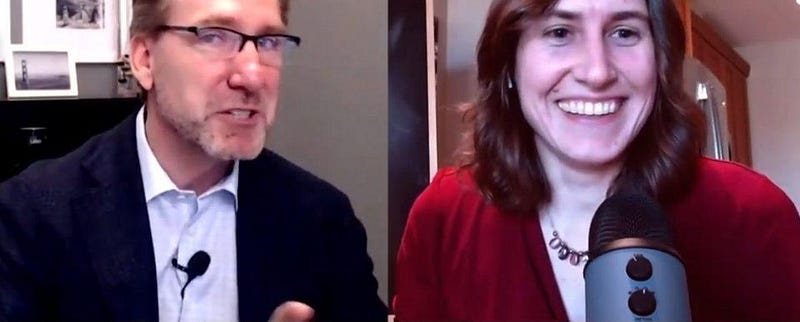
4:05 PM: For those of you expecting an organized, tight lecture, I can assure you that Katie Mack is very good at those, but to switch to a new format is extremely challenging. The end of the Universe is the topic of Katie’s new book, and you can pre-order it now, and it comes out in just 3 short months: on August 4th.
4:08 PM: There are a lot of things to consider when it comes to the very end, because extremely long timescales (much longer than the current age of the Universe) aren’t things in our experience. This leads to questions you might never thing to ask, because they’re not relevant to our Universe.
For example:
- Will atoms remain stable, or will they all decay?
- Does everything decay, or will we still have structures forever?
- Will there be a new transition coming at some point?
- Will there be a rejuvenation or a cyclic occurrence?
- Or will everything continue as this “vanilla” scenario, with a constant dark energy and a “heat death” that we asymptotically approach?
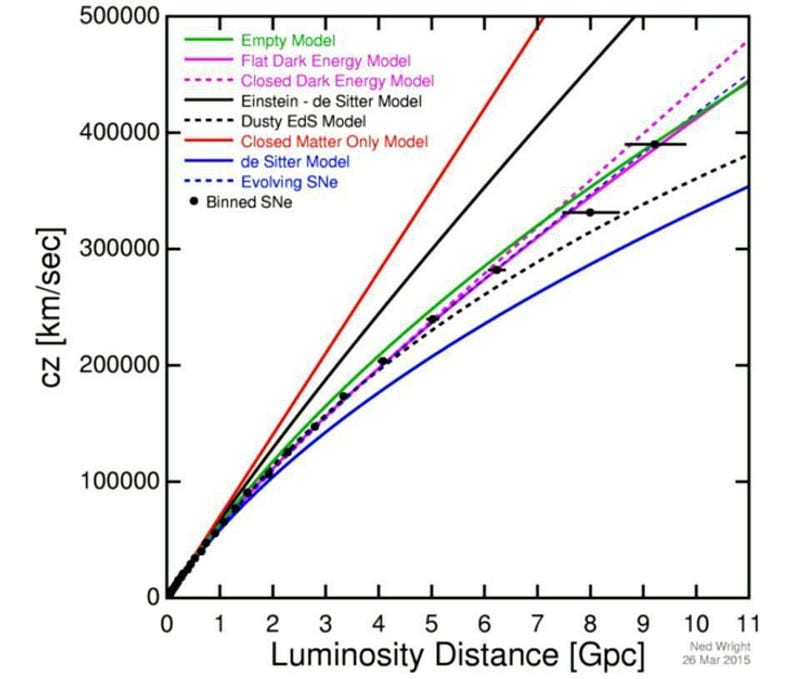
4:11 PM: You have to appreciate what a surprise the above discovery (that Katie refers to) actually was. The Universe, if it were just a “matter-and-radiation” on one hand and “expansion” on the other hand, fighting each other, the actual curve that we see would never be a possibility.
There must be some sort of new ingredient, and that’s where dark energy comes in.
4:14 PM: A lot of people are dissatisfied with the idea of the heat death of the Universe, but this is kind of interesting. About 2 generations ago, there was this prejudice that the Universe should end in a Big Crunch: in a recollapse scenario. There was no physical reason for it; it just “seemed natural” to most people. Penrose’s Conformal Cyclic Cosmology is one modern version of such a scenario, but it doesn’t have the evidence you’d want to support it.
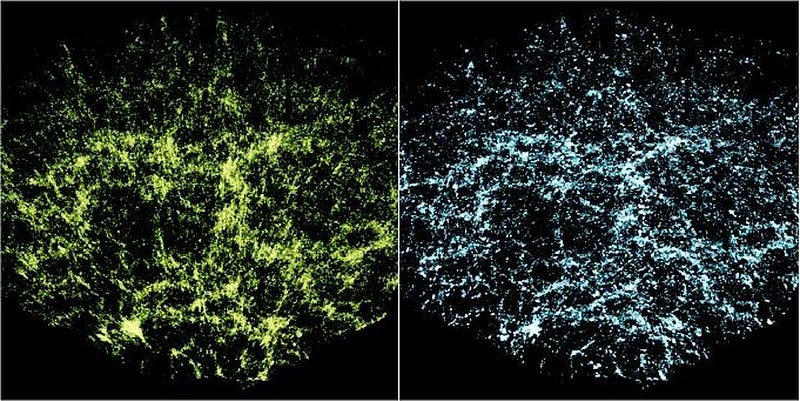
In fact, that’s an enormous problem for alternatives to the heat death: they have big problems trying to reproduce what we’ve already observed. Penrose’s idea, in particular, fails because it cannot reproduce the large-scale structure of the Universe that we observe the Universe to have.
4:16 PM: Could the Universe end today? Or right now? That’s the vacuum decay transition, and it is, in fact, eminently possible. If it happened, we’d transition to a lower-energy state than we’re currently in right now. It would be like quantum tunneling from the state we’re in to an even lower, closer-to-zero energy state. The fact that dark energy exists tells us that this may be possible.
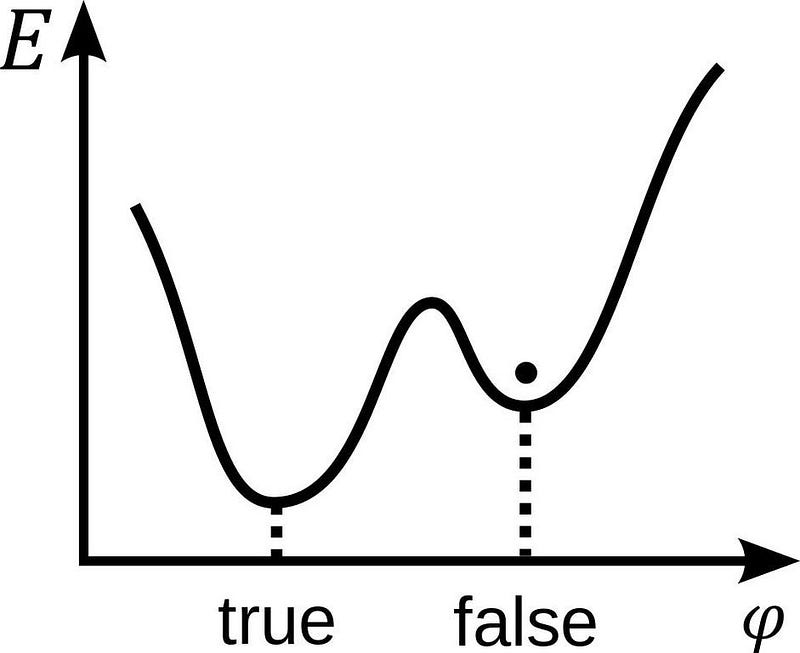
So here we go, and this changes all sorts of things. Fundamental constants, masses, the properties of atoms, etc. If we made this transition, even in one region of space, it would propagate outward at the speed of light and cause this destructive transition everywhere that was affected.
Once it got to us, that would be our end. Exciting, but absolutely terrifying.
4:20 PM: Why would we worry about the vacuum decaying? Well, one is that we might be in a meta-stable state, but the other is that the Higgs itself could take on a lower-energy configuration. Remember that the Higgs boson has a particular mass, and its coupling to all the other particles determines what their rest masses are.
But now, we go into a lower energy state, and the Higgs boson can take on a different mass and the couplings change. And, as Katie puts it, “everything is over.” But quantum tunneling, even if we can’t transition directly from the false vacuum we currently occupy to the true vacuum, we might get there even if we couldn’t classically. And that, in fact, would end the Universe as we know it.
4:22 PM: For those of you looking for an illustration of quantum tunneling, you might really enjoy this animation.
Or, perhaps, you want an example that involves real, actual photons, some of which are reflected and some of which actually tunnel through the barrier.
4:25 PM: What’s messed up is that with dark energy, this “expanding bubble” of true vacuum that’s trying to get us in the false vacuum is only going to get about 3% of the observable Universe, even if it were to happen right now! It’s dramatic and unlikely, but even if it occurs, even then, it’s not likely to “get” us.
4:28 PM: The way it could be possible to get a Big Crunch, even today, would be if dark energy somehow evolved in a way to reverse its sign. It would mean that the expansion would reach some maximum, and that distant galaxies would stop receding and would turn around to start contracting.
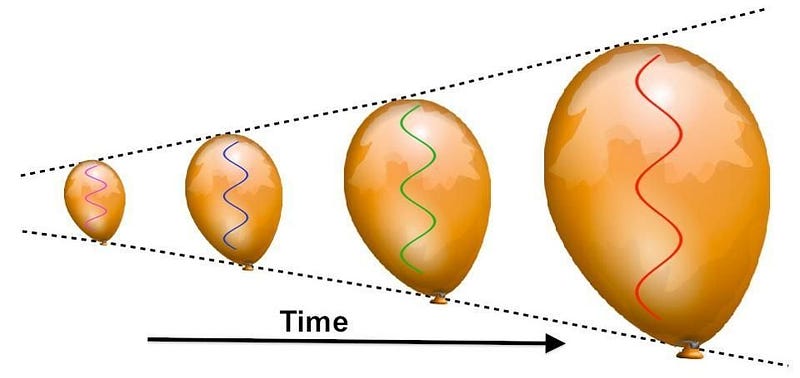
This is terrifying, as recontracting would make the Universe heat up again, as the opposite of “redshift” is blueshift. Eventually, we’d get cooked, as our atoms would get ionized and it would be impossible for electrons to remain bound to their atomic nuclei.
It’s a terrifying scenario, as Katie says, but the nice thing is that it would take at least the current age of the Universe on top of how old things are already for this to happen in our future.
4:32 PM: One of the things that Katie’s talking about is her athletic history, and I think this is important for everyone, even if you’re not athletically inclined: it’s vital to be a well-rounded person. You have an entire life ahead of you, however you choose to spend it, and spending 100% of your time on work — even if you love your work — is not going to give you satisfaction in all areas of your life.
Make friends. Do activities that interest you. Use your body. Use your mind in ways you aren’t accustomed to. Learn. Go outside your area(s) of expertise. And get experience at things you aren’t good at; come to terms with “failure” as a milestone on the road to success. What each of us does with our lives will not look exactly like what anyone else looks like. But do it any way. Make it a part of your journey. The reward is not only a well-lived life, but a way to relate to others who don’t love your work as much or in the same way as you do. (Which is everyone except you, BTW.)
4:36 PM: I like what Katie is talking about concerning how she interacts with people on Twitter, or in the public arena. How she doesn’t punch down. How she tries to be nice and helpful. How she tries to be a good source of accurate information. How to be a positive presence and a good role model. I like how she doesn’t try to abdicate that responsibility, even when there’s no benefit to her other than just doing good in the world.
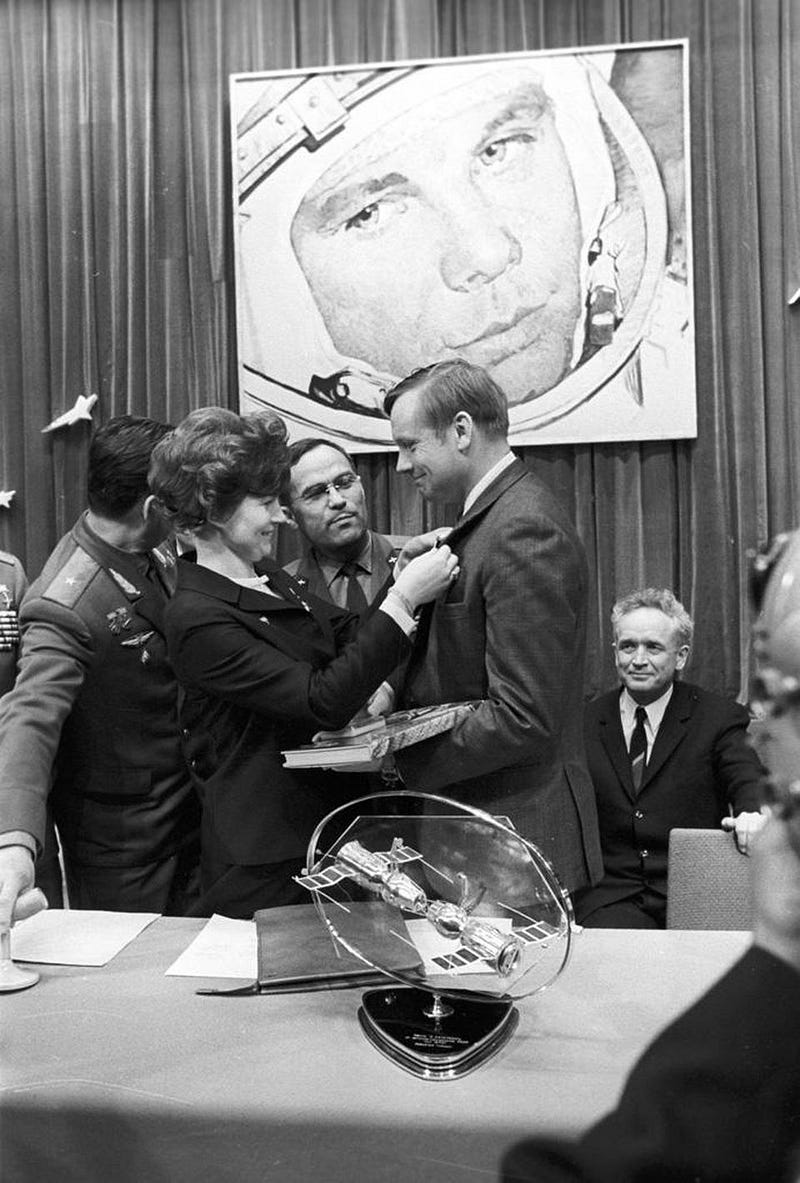
4:39 PM: Scientists don’t normally get the fame or accolades that people engaged in arguably less heroic pursuits are awarded, but that doesn’t mean that scientists can’t be the ambassadors for the better world we want to create and live in. I like this idea.
4:42 PM: So cosmic inflation, which I’m very excited about (and is the subject of my next book), actually came about in a “wrong” incarnation. It is now called “old” inflation, because what it did right was:
- explain the puzzles we wanted to solve that we had identified as gaps with the hot Big Bang,
- could make new predictions for certain effects that differed from the infinite-temperature and infinite-density hot Big Bang,
which is great. But the one thing it needed to do is “reproduce all the successes of the hot Big Bang,” and it failed on a big one: giving us a Universe that had the same temperature and energy density everywhere. It couldn’t do that, unfortunately, but that didn’t mean it was a dead end.
Instead, it was promising enough that, over the next year or two, a couple of independent teams found a way to keep the successes of inflation and solve the problem that it couldn’t. That first successful model was called “new inflation,” and it is still viably valid today.
4:45 PM: For an even greater level of detail, you can view inflating space as a pot of water that’s at the boiling point, and the regions where inflation ends as the bubbles within that water. In old inflation, because of the way inflation ends, the energy winds up in the bubble walls, with the original idea being that the bubble walls would splash together and create our uniform Universe.
But it turns out that the bubbles don’t collide in old inflation, so there’s no way to get a homogeneous Universe. But in new inflation, the way they solved that problem was to come up with a different way to end inflation, and that puts the energy (evenly, everywhere) in the insides of the bubbles. That’s the difference, in technical terms, between a first-order and a second-order phase transition, and that was the revelation of new inflation.
4:48 PM: What happens to matter when it falls inside a black hole? We only can ever observe it from the outside, so the only three things that change (according to Einstein) are its mass, its electric charge, and its spin (or angular momentum).
But is there information encoded on its surface? Do things get crushed down to a singularity? Do things create a new Universe at the inner horizon?
These are fun theoretical questions to explore, but there’s no known way to uncover evidence to test any of these ideas. Once you cross that event horizon, all you have left is what you can observe from the outside.
4:50 PM: Above, by the way, is my favorite visualization of how a mass moving through space “curves” the space that it moves through. It’s pretty good stuff; if you normally envision space as a series of grid lines in 3D, a gravitational source (or a mass) basically pulls all those lines in towards it, which causes space to bend. If an object moves through that space, it “flows” towards the mass, and in the case of a black hole, it’s just having enormously large amounts of mass in a very tiny volume of space.
4:53 PM: Are space and time not fundamental? I think there’s a very important thing to state here (that Katie is too kind to say): there is a difference between what’s fashionable (which is this idea) and what’s well-motivated by data, experiment, or even the logical consistency of a theory.
Right now, there are many things that are fashionable that are fashionable because people are choosing to work on them, but I might argue that the field would be just as healthy, or perhaps even healthier, if large numbers of people weren’t working on them. Everyone is free to choose what to work on based on where their intellectual curiosity drives them, but in the absence of concrete progress that has a connection to a physical measurable or observable, all of these pursuits should be looked at with at least a small grain of salt.
4:55 PM: “I hope that if anyone is really interested in this topic, I really hope that they’ll consider picking up this book, because it’s a labor of love but also […] because it’s really written for everyone. It’s not written for specialists, but even if you do have a lot of knowledge in physics, you might learn something reading it because I learned something writing it.” -Katie Mack’s final thoughts.
Thanks for participating in this live-blog and thanks for listening to some excellent thoughts concerning the end of the Universe, and everything from now until then, however it might turn out.
Ethan Siegel is the author of Beyond the Galaxy and Treknology. You can pre-order his third book, currently in development: the Encyclopaedia Cosmologica.