What Was It Like When The First Supermassive Black Holes Formed?
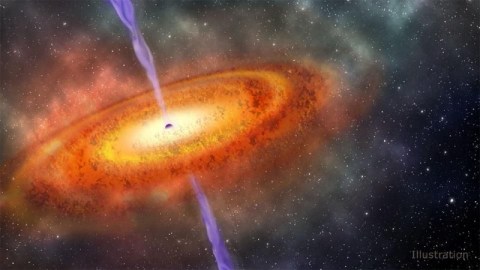
These cosmic behemoths were enormous from very early times. Here’s how they came to be.
One of the biggest challenges for modern astrophysics is to describe how the Universe went from a uniform place without planets, stars, or galaxies to the rich, structured, diverse cosmos we see today. As far back as we can see, to when the Universe was just a few hundred million years old, we find a slew of fascinating objects. Stars and star clusters exist in abundance; galaxies with perhaps a billion stars light up the Universe; even quasars with very large black holes formed before the Universe was even one billion years old.
But how did the Universe make such ultra-massive black holes in such short periods of time? After decades of conflicting stories, scientists finally think we know what happened.
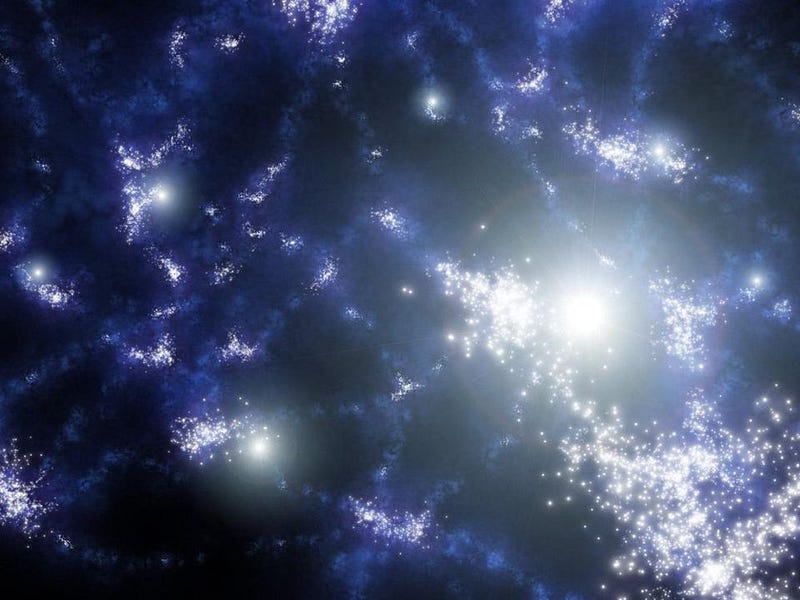
Just 50-to-100 million years after the Big Bang, the very first stars of all began to form. Massive gas clouds started to collapse, but because they were made up of hydrogen and helium alone, they struggle to radiate heat away and dissipate their energy. As a result, these clumps that gravitationally form and grow need to get much more massive than clumps that form stars today, and that has repercussions for what kinds of stars form.
While today, typically, we form stars that are about 40% the mass of the Sun, the very first stars were about 25 times more massive, on average. Because you need to cool in order to collapse, it’s only the largest, most massive clumps that form early on that will lead to stars. The average “first star” might be ten times as massive as our Sun, with many individual stars reaching hundreds or even a thousand solar masses.
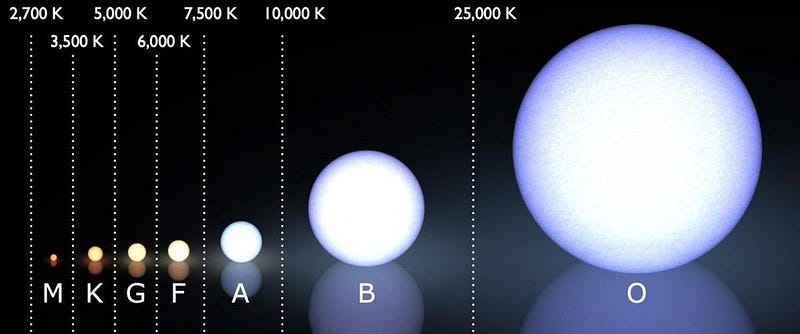
Most of these stars will end their life in a supernova, leading to either a neutron star or a small, low-mass black hole. But without any heavy elements at all, the most massive stars will reach such high temperatures in their cores that photons, the individual particles of light, can become so energetic that they will spontaneously begin to produce matter and antimatter pairs from pure energy alone.
You may have heard of Einstein’s E = mc², and this is perhaps its most powerful application: a pure form of energy, like photons, can create massive particles so long as the fundamental quantum rules governing nature are obeyed. The easiest way to make matter and antimatter is to have photons produce an electron/positron pair, which will happen all on its own if temperatures are high enough.
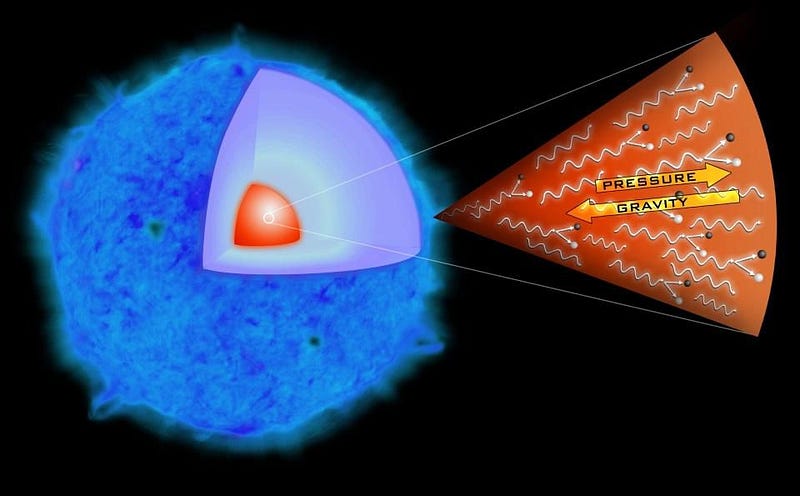
In these ultra-massive stars, as in all stars, gravitation is attempting to pull all of that matter in towards the center. But photons, and all of the radiation produced in the cores of these stars, pushes back, and holds the star up, preventing its collapse.
When you start producing electron-positron pairs from these photons, however, you’re losing some of that radiation pressure. You’re depleting your star’s ability to hold itself up against gravitational collapse. And while it’s true that there are a few, narrow mass ranges that lead to the star destroying itself entirely, a large fraction of cases will result in the entire star directly collapsing to form a black hole.
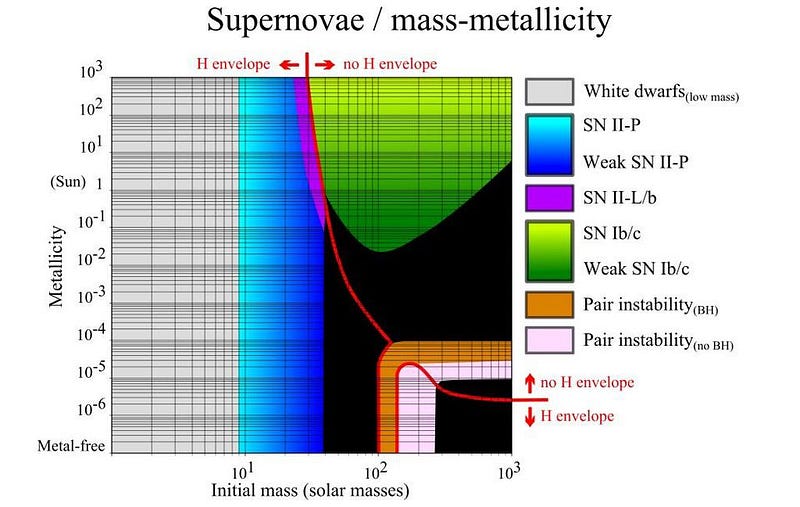
This is a remarkable step! It means that the most massive stars of all, with many hundreds or even a thousand solar masses, can form when the Universe is just 100 million years old or so: less than 1% its current age. These stars will burn through their nuclear fuel the fastest, in 1 or 2 million years, tops. And then, their cores will get so hot they’ll start turning photons into particles and antiparticles, which causes the star to collapse and heat up even faster.
Once you cross a certain threshold, all you can do is collapse. And this isn’t just theory, either; we’ve actually seen stars directly collapse without a supernova, leading directly to what could only be a black hole.
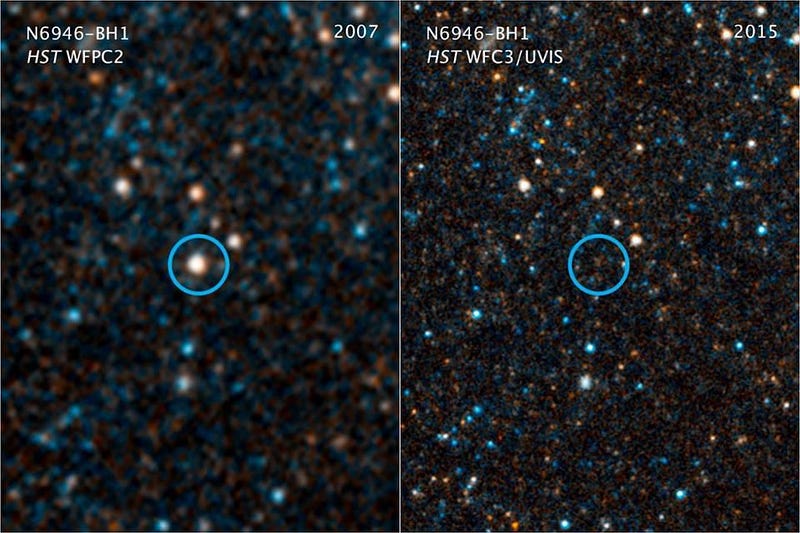
But that’s only the beginning. Whenever you have a large cluster of massive objects acting primarily under the force of gravity, different objects get kicked around from these interactions. The least massive objects are the ones that are the easiest to eject, while the most massive objects are the toughest to eject. As these stars, gas clouds, clumps, and black holes dance around, they undergo what’s known as mass segregation: the heaviest objects fall to the gravitational center, where they interact and can even merge.
All of a sudden, instead of a few hundred black holes of a few hundred or a few thousand solar masses, you can wind up with one single black hole of approximately 100,000 solar masses or even more.
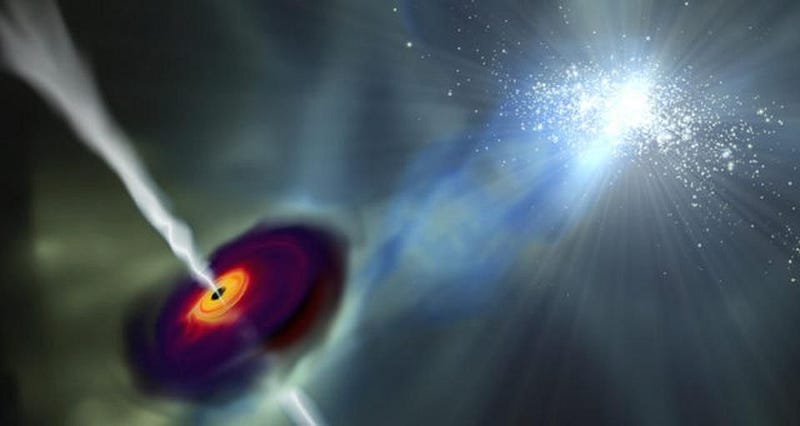
Although, gravitationally, it might take tens of millions of years for this to happen, this is just for a single star cluster! The Universe, from its earliest stages, is forming these star clusters all over the place, and these star clusters then begin to gravitationally attract one another. Over time, these disparate star clusters will influence one another, and gravity will bring them together.
By time the Universe is no more than 250 million years old, they will have begun to merge together en masse, leading to the first proto-galaxies. Gravity is a force that truly favors the overdog, and as time goes on, tens, hundreds, and even thousands of these initial, early clusters can come together to grow into larger and larger galaxies. The cosmic web causes structures to merge together into ever-larger ones.
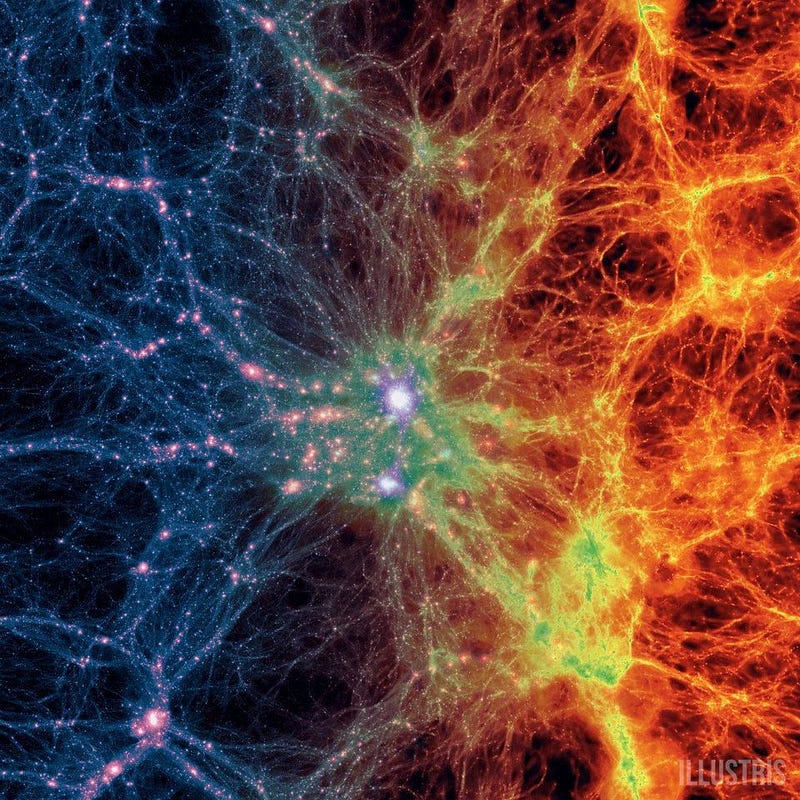
This can easily take us up to masses that are many tens of millions of solar masses by time we get to the first galaxies, but something else happens, too. It isn’t just black holes that merge together to build supermassive ones in the center; it’s any matter that falls into them! These early galaxies are compact objects, and are full of stars, gas, dust, star clusters, planets and more. Whenever anything gets too close to a black hole, it’s at risk of getting devoured.
Remember that gravity is a runaway force: the more mass you have, the more mass you attract. And if something gets too close to a black hole, its matter gets stretched and heated, where it will become part of the black hole’s accretion disk. Some of that matter will get heated and accelerated, where it can emit quasar jets. But some of it, too, will fall in, causing the black hole’s mass to grow even further.
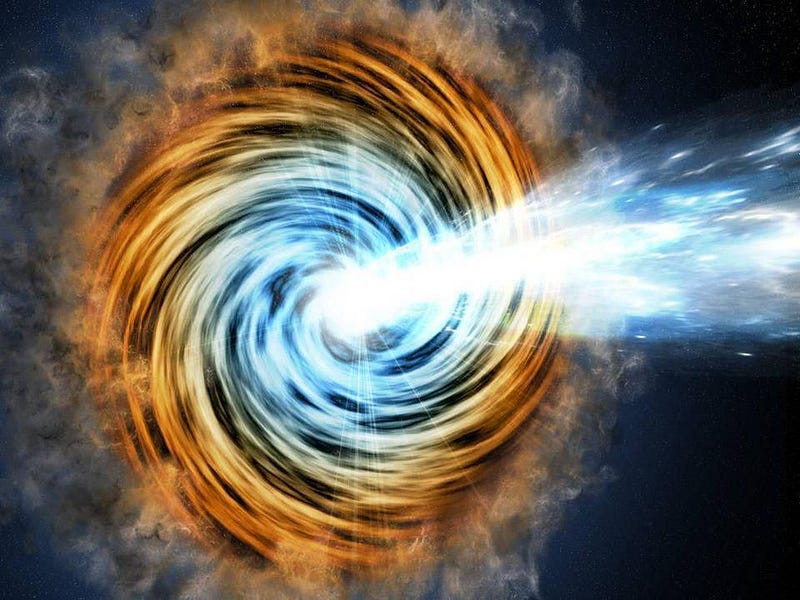
If there were one vocabulary word that astrophysicists who study the growth of object via gravity wish that the general public knew, it would be this oddball: nonlinear. When you have a region of space that’s denser than average, it preferentially attracts matter. If it’s just a few percent denser than average, the gravitational attraction is just a few percent more effective than average. Double the amount that you’re overdense, and you double the amount you’re more effective at attracting stuff.
But when you reach a certain threshold of being about double the average, you become much more than twice as effective at attracting other matter. When you start “winning” the gravitational war, you win harder and harder as time goes on. The most massive regions, therefore, not only grow the fastest, they eat everything around them. By time half a billion years go by, you can be enormous.
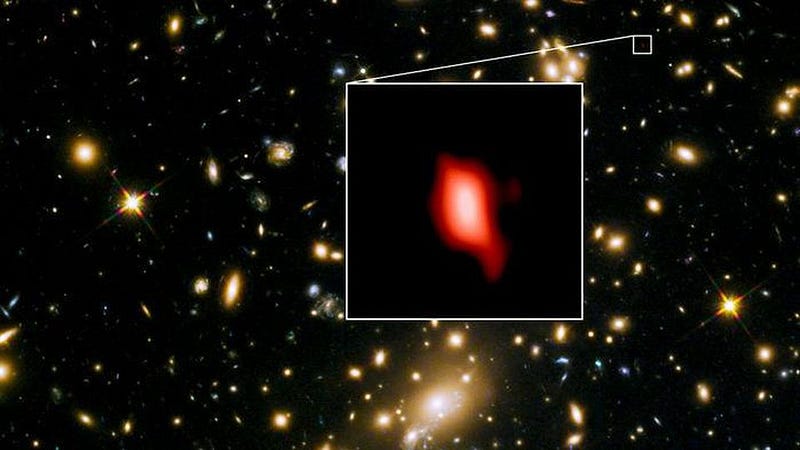
The earliest galaxies and quasars we’ve ever found are among the brightest, most massive ones we expect to exist. They are the great winners in the gravitational wars of the early Universe: the ultimate cosmic overdogs. By time our telescopes reveal them, 400-to-700 million years after the Big Bang (the earliest quasar comes from 690 million years), they already have billions of stars and supermassive black holes of many hundreds of millions of solar masses.
But this is not a cosmic catastrophe; this is a piece of evidence that showcases the runaway power of gravitation in our Universe. Seeded by the first generation of stars and the relatively large black holes they produce, these objects merge and grow within a cluster, and then grow even larger as clusters merge to form galaxies and galaxies merge to form larger galaxies. By today, we have black holes tens of billions as massive as the Sun. But even in the earliest stages we can observe, billion-solar-mass black holes are well within reach. As we peel back the cosmic veil, we hope to learn exactly how they grow up.
Further reading on what the Universe was like when:
- What was it like when the Universe was inflating?
- What was it like when the Big Bang first began?
- What was it like when the Universe was at its hottest?
- What was it like when the Universe first created more matter than antimatter?
- What was it like when the Higgs gave mass to the Universe?
- What was it like when we first made protons and neutrons?
- What was it like when we lost the last of our antimatter?
- What was it like when the Universe made its first elements?
- What was it like when the Universe first made atoms?
- What was it like when there were no stars in the Universe?
- What was it like when the first stars began illuminating the Universe?
- What was it like when the first stars died?
- What was it like when the Universe made its second generation of stars?
- What was it like when the Universe made the very first galaxies?
- What was it like when starlight first broke through the Universe’s neutral atoms?