What Is The Ultimate Fate Of The Loneliest Galaxy In The Universe?

In the middle of a great cosmic void, a single, isolated galaxy persists amidst the darkness. It’s about to get a lot lonelier.
Here in our own cosmic backyard, the Milky Way is just one galaxy among many. A slew of satellites galaxies accompany us on our journey through the Universe, and our nearby neighbor Andromeda outclasses us in terms of mass, stars, and even physical extent. All told, we’re just one of perhaps ~60 galaxies bound in our local group, which itself is a modestly small galaxy group on the outskirts of the enormous Virgo Cluster.
Not every galaxy is so fortunate, however. While galaxies are most commonly found bound together in large numbers, there are enormous cosmic voids separating the rich structures found throughout the Universe, with only tiny amounts of matter inside. One remarkable example is the galaxy MCG+01–02–015, which is the only one around for some 100 million light-years in all directions. It’s the loneliest galaxy in the known Universe, and we can scientifically predict its ultimate fate.
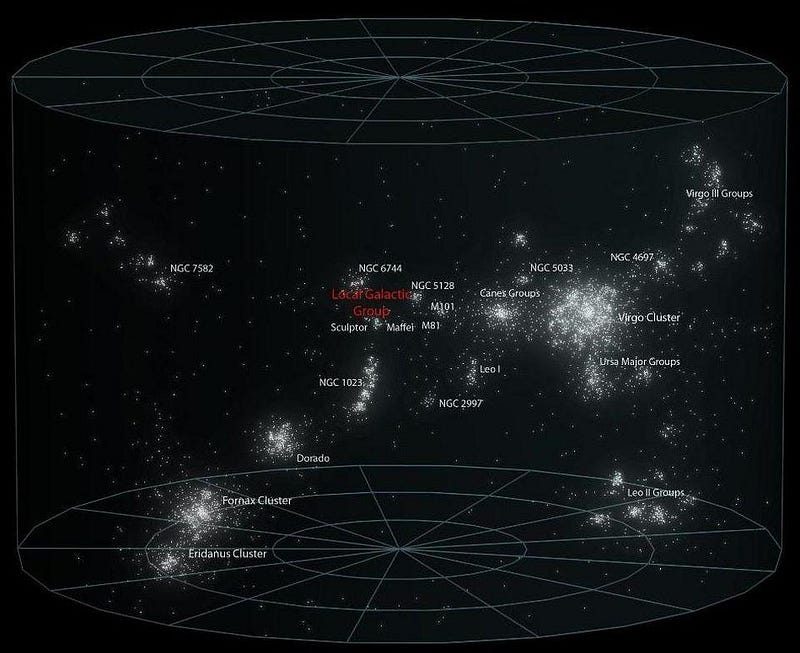
To understand what this galaxy is going to do, first we have to understand what it’s like from the inside out. When the Universe was much younger than it is today, it was almost perfectly uniform, with regions that are only slightly overdense or underdense compared to the large-scale average. The regions with more matter than average will self-gravitate, drawing in matter from the surrounding volumes of space and eventually leading to the formation of stars, galaxies, and groups and clusters of galaxies on even larger scales.
Regions that are underdense, however, tend to give up their matter to the surrounding overdense regions, leading to vast cosmic voids between the strands of the cosmic web. Contrary to popular belief, however, even the regions of below-average density still tend to hang on to some amount of matter — both normal and dark — and with enough time, that matter will collapse to form structures, too.
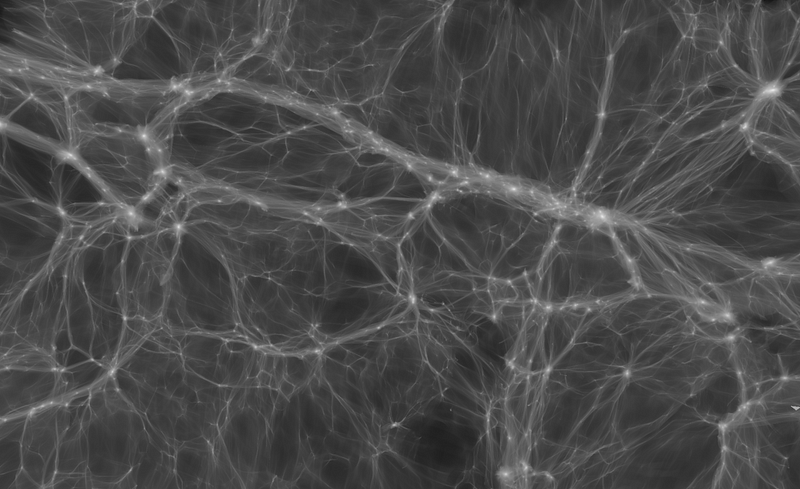
The overwhelming majority of galaxies, today, can be found along the filaments of our cosmic large-scale structure, with enormous concentrations of galaxies existing at the nexus points of multiple filaments. It’s dark matter that drives the formation of this cosmic web — outmassing normal matter by a relatively consistent 5-to-1 ratio — while it’s the normal matter that collides, heats up, sheds momentum, and forms stars.
The matter remaining in a cosmic void, rather than undergoing a complicated story of gravitational growth from a series of mergers, will instead tend to form a large, isolated single galaxy via monolithic collapse. From a distance, a galaxy that forms like this might appear very similar to any other spiral galaxy, such as Andromeda, but there are important additional properties that only a more detailed investigation will reveal.
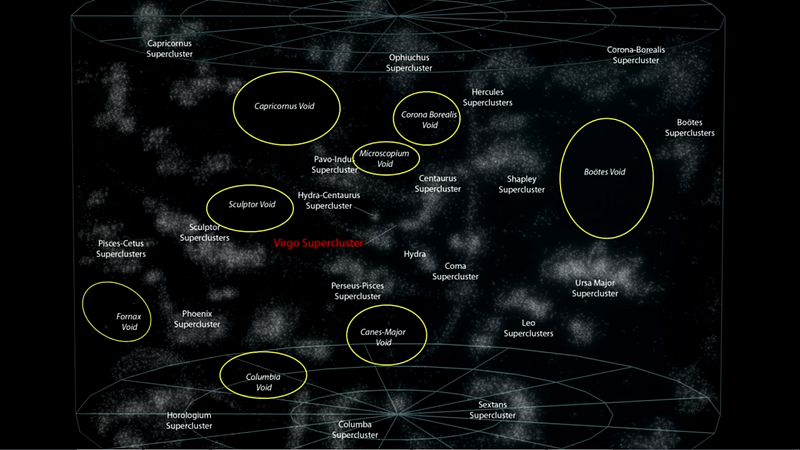
An extremely isolated galaxy, unlike their more common, more clustered counterparts, forms as follows.
- The regions that fail to give up all of their matter to the filamentary network that comprises our large-scale structure will gravitate towards their mutual center-of-mass, determined by the presence of both dark matter and normal matter.
- The dark matter forms a large, diffuse halo of mass, while the normal matter sinks to the center, colliding with other normal matter particles and collapsing in the shortest dimension first.
- The normal matter “pancakes,” which is the scientific term for “goes splat,” and forms a disk that starts rotating.
- Inside the disk, stars form, leading to the familiar spiral structure we recognize.
- Dark matter gets dynamically heated, changing its density profile somewhat, while low-mass neutrinos eventually fall into the halo, adding to the mass.
Subsequently, the normal matter goes through the normal stellar life cycle, leading to the isolated galaxies we see today.
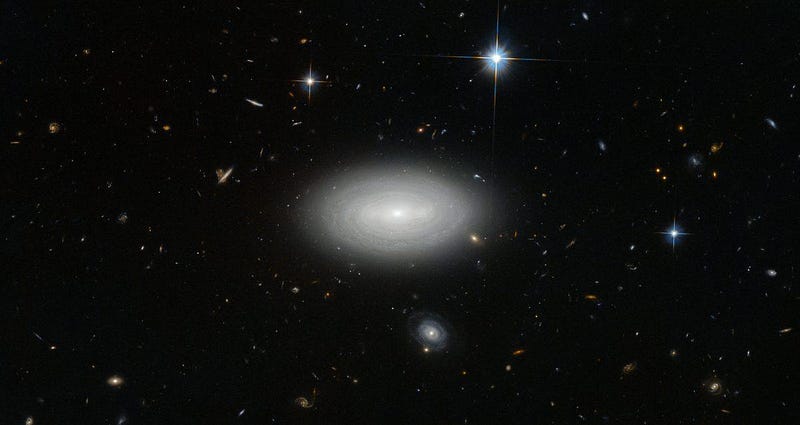
But the Universe is just getting started. Dominated by dark energy, distant galaxies will not only recede from one another, but their apparent recession speeds will increase faster and faster as time goes on. For galaxies like our own, we’ll remain bound to our local group, including Andromeda, Triangulum, and about 60 additional galaxies, until they all merge together many billions of years in the future. Galaxies beyond our gravitationally bound group, like those in the Virgo cluster, will remain bound to their own parent groups, but will accelerate in their recession from our own.
For an isolated, lonely galaxy, however, all of the galaxies and galactic groups will accelerate away. A galaxy like MCG+01–02–015 will remain isolated, forming stars in bursts lining its spiral arms for as long as new material to form new generations of stars remains.
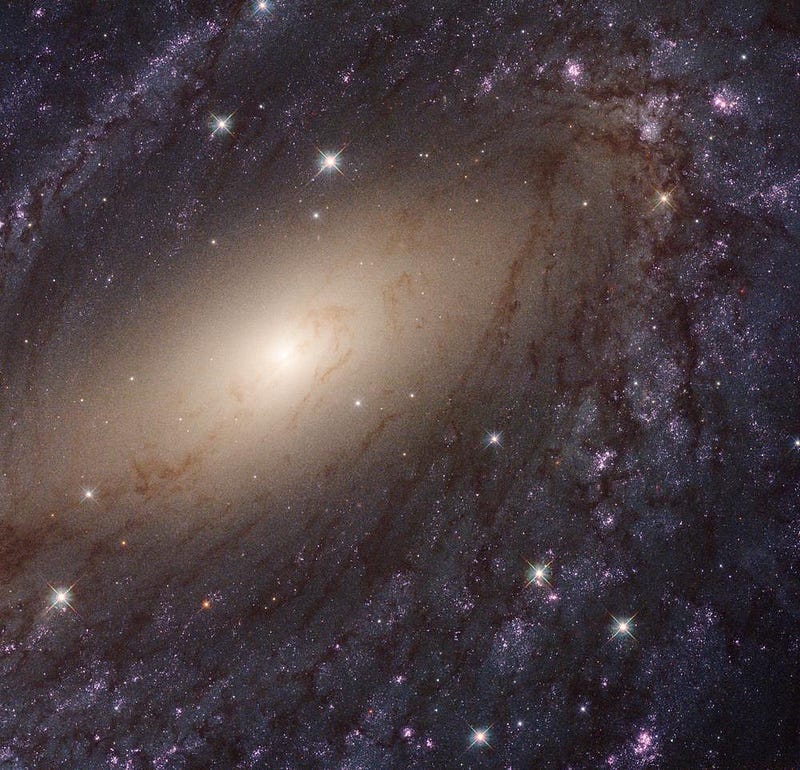
Over the next few tens of billions of years, all the galaxies that can be seen will accelerate away, leaving only some highly redshifted photons behind. Besides those, 100 billion years from now, there will be no indication that any other galaxies ever existed within our visible Universe.
Star formation rates will continue dropping inside each galaxy, with Sun-like stars burning out and only the least massive stars — the red dwarfs and their failed-star (brown dwarf) counterparts — continuing to shine. As billions of years turn into trillions or even hundreds of trillions of years, even these stars will burn through all of their fuel. White dwarfs, the dead remnants of most stars, will eventually fade away to become black dwarfs, as they cool down to become completely invisible.
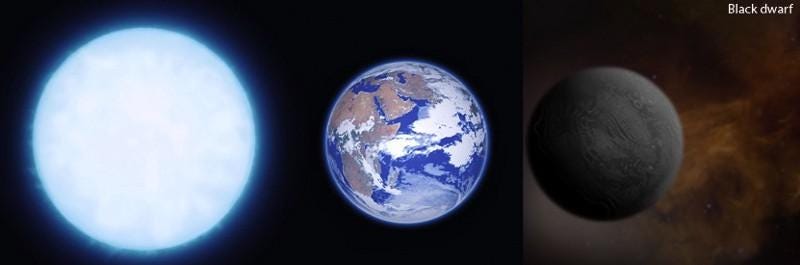
After around a quadrillion (10¹⁵) years have passed, the last stellar remnants will have burned out, darkening the Universe. Only the occasional merger of multiple objects, such as brown dwarfs, will cause a temporary reignition of nuclear fusion, creating starlight for tens of trillions of years at a time. Those events will not only be rare, but will have to fight against a competing process.
All of the collapsed objects, which is where the normal matter will overwhelmingly wind up, will gravitationally interact. The random close encounters between masses will, over time:
- lead to gravitational interactions and momentum exchange,
- ejecting the lightest ones, hurling them into intergalactic oblivion,
- and causing the heavier-mass objects to sink towards the center, losing momentum in a process known as violent relaxation.
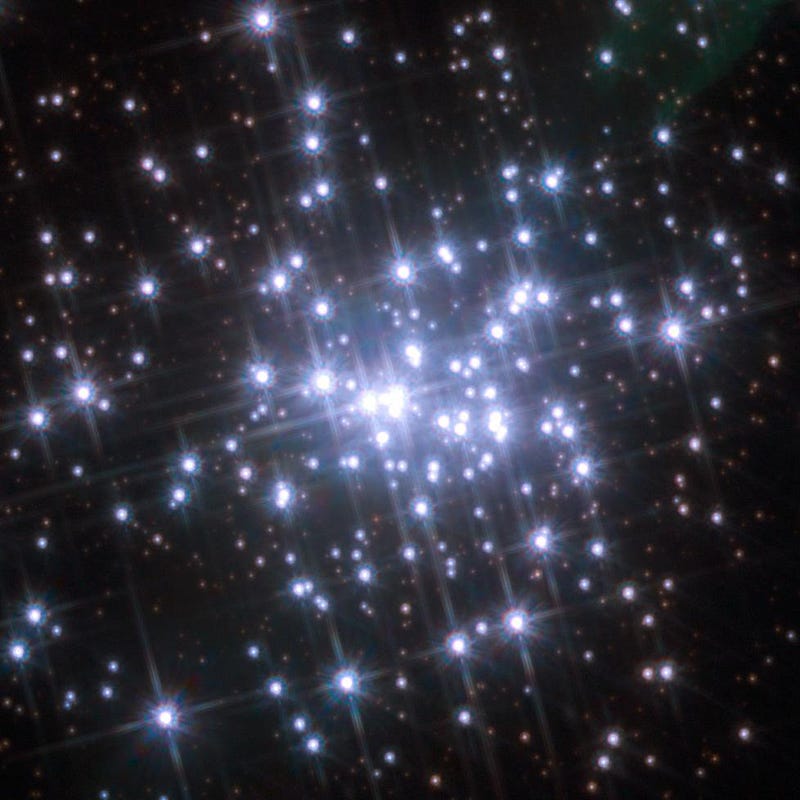
After enough time has passed, somewhere around 10¹⁹ or 10²⁰ years, only a small percentage of those masses composed of normal matter will remain, largely in the form of black holes or stellar remnants. Yet the large, diffuse halo of non-normal matter — dark matter and massive neutrinos — will remain largely unchanged; the evolution of normal matter should have only negligible effects here.
As we add more zeroes to the age of the Universe, the central black hole will grow by devouring matter, flaring when it does. The planets that remain in orbit around dead stellar remnants will see their orbits decay via gravitational radiation, spiraling into their remnants. Eventually, all the normal matter will be either ejected or concentrated into massive and supermassive black holes. Still, that halo of dark matter and neutrinos will remain.
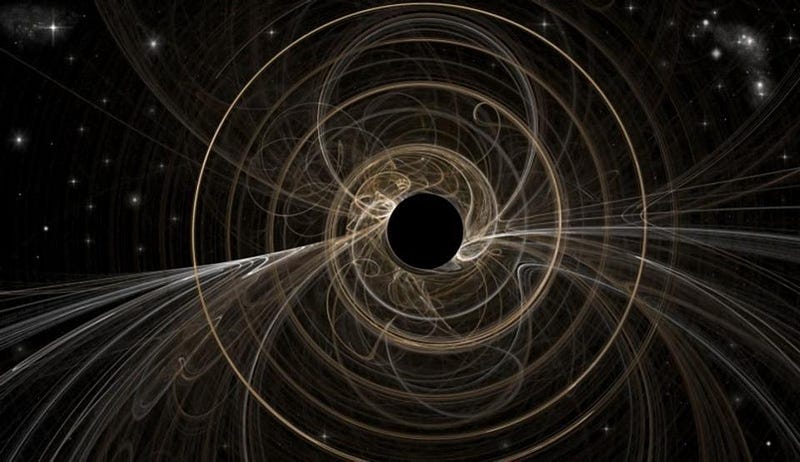
As the aeons tick past and the Universe ages even more severely, black holes themselves will decay through the quantum process of Hawking radiation. Stellar mass black holes will evaporate on timescales of around 10⁶⁷ years, while the most massive black holes in today’s Universe might persist for around 10¹⁰⁰ years. If we were to examine the most isolated galaxy of all, its black hole is likely to last 10⁸⁰ to 10⁹⁰ years, but no more.
Yet even when that much time has passed, and the last black hole in the most isolated galaxy we know of has decayed away, dark matter and neutrinos will still exist in the same enormous halo-like configuration they always did. Even without normal matter to absorb or emit radiation, the skeletal structure of the galaxy — the dark matter and neutrinos that don’t interact with photons — will still persist.
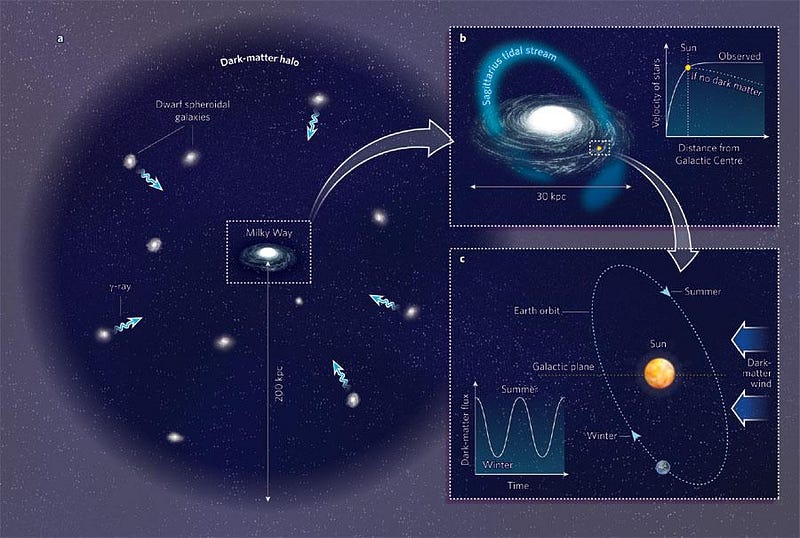
After an extraordinary amount of time has passed, googols of years or even more, the loneliest galaxy in the Universe will appear completely empty. No stars, stellar remnants, planetary corpses or even black holes ought to remain. And yet, it will still exist. Someone who could measure the spacetime curvature of the Universe or somehow detect dark matter or ultra-low energy neutrinos would encounter an enormous, diffuse halo of mass that will persist for far longer than any bound structure made of normal matter.
Eventually, dependent on the actual (and yet unknown) masses of individual dark matter particles and neutrinos, this remnant dark halo will decay, ejecting itself particle-by-particle until none remain. Until the masses and properties of those particles are known, however, we cannot calculate that timescale; we can only know it will persist longer than any normal matter will. The eventual fate of the last galaxies in the Universe will be a skeletal dark matter/neutrino halo, far outlasting anything else we’ve ever observed.
Ethan Siegel is the author of Beyond the Galaxy and Treknology. You can pre-order his third book, currently in development: the Encyclopaedia Cosmologica.