What is the future of gravitational wave astronomy?
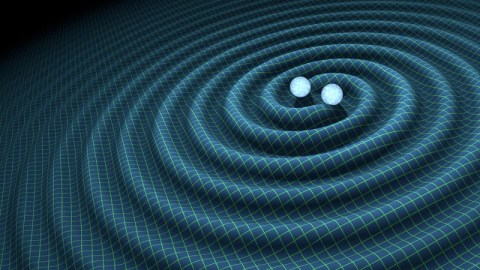
Now that LIGO’s found two pairs of merging black holes, what does the future hold?
“We shall not cease from exploration, and the end of all our exploring will be to arrive where we started and know the place for the first time.” -T.S. Eliot
After turning on in September of 2015, the twin Laser Interferometer Gravitational-wave Observatories — the LIGO detectors in Hanford, WA and Livingston, LA — simultaneously detected not just one but two definitive black hole-black hole mergers during its first run, despite having reached only 30% of the sensitivity it was designed for. These two events, one of a 36 and a 29 solar mass black hole merging on September 14, 2015, and one of a 14 and an 8 solar mass black hole merging on December 26, 2015, provided the first definitive, direct detections of the gravitational wave phenomena. It’s a remarkable fact, in and of itself, that it took a full century after their predictions for technology to catch up to the theory, and actually catch them.
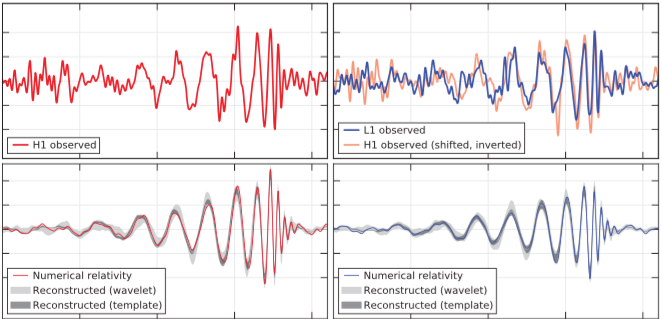
But detecting these waves is just the beginning, as a new era in astronomy is now dawning. 101 years ago, Einstein put forth a new theory of gravitation: General Relativity. Instead of distant masses instantaneously attracting one another across the Universe, the presence of matter and energy deformed the fabric of spacetime. This entirely new picture of gravity brought with it a slew of unexpected consequences, including gravitational lensing, an expanding Universe, gravitational time dilation and — perhaps most elusively — the existence of a new type of radiation: gravitational waves. As masses moved or accelerated relative to one another through space, the reaction of space itself causes the very fabric itself to ripple. These ripples travel through space at the speed of light, and when they pass through our detectors after a journey across the Universe, we can detect these disturbances as gravitational waves.
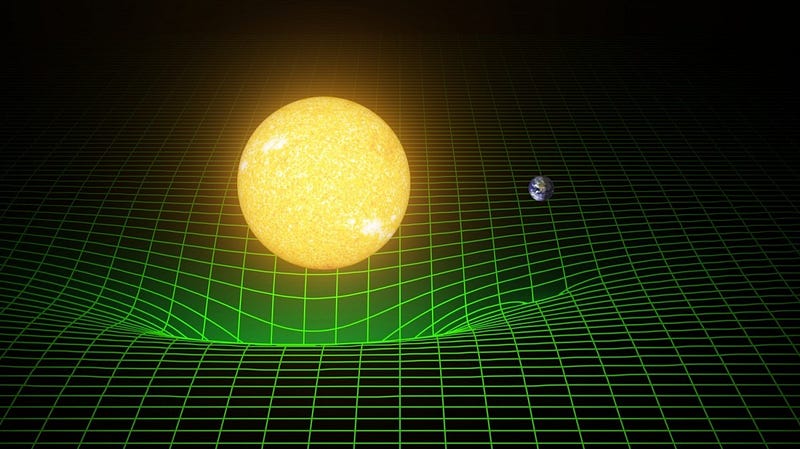
The easiest things to detect are the things that emit the largest signals, which are:
- large masses,
- with small distances between them,
- orbiting quickly,
- where the orbital changes are severe and significant.
This means collapsed objects, like black holes and neutron stars, are the prime candidates. We also need to consider the frequency at which we can detect these objects, which will be roughly equal to the path length of the detector (the arm length multiplied by the number of reflections) divided by the speed of light.
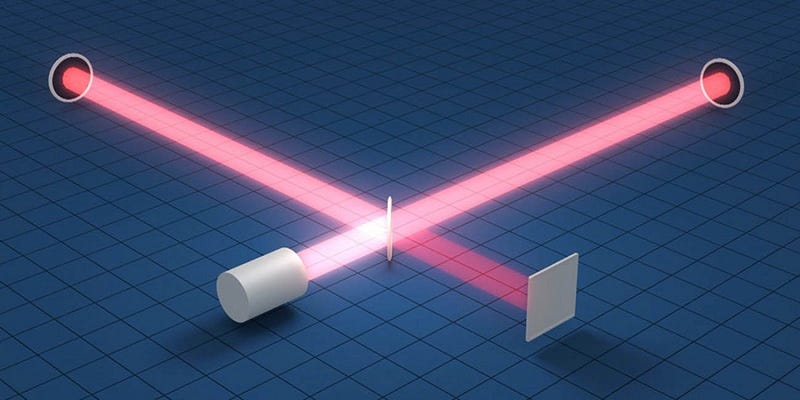
For LIGO, with its 4 km arms with a thousand reflections of the light before creating the interference pattern, it can see objects with frequencies in the millisecond range. This includes coalescing black holes and neutron stars in the final stages of a merger, along with exotic events like black holes or neutron stars that absorb a large chunk of matter and undergo a “quake” to become more spherical. A highly asymmetric supernova could create a gravitational wave as well; a core-collapse event is unlikely to make detectable gravitational waves but perhaps nearby merging white dwarf stars could do it!
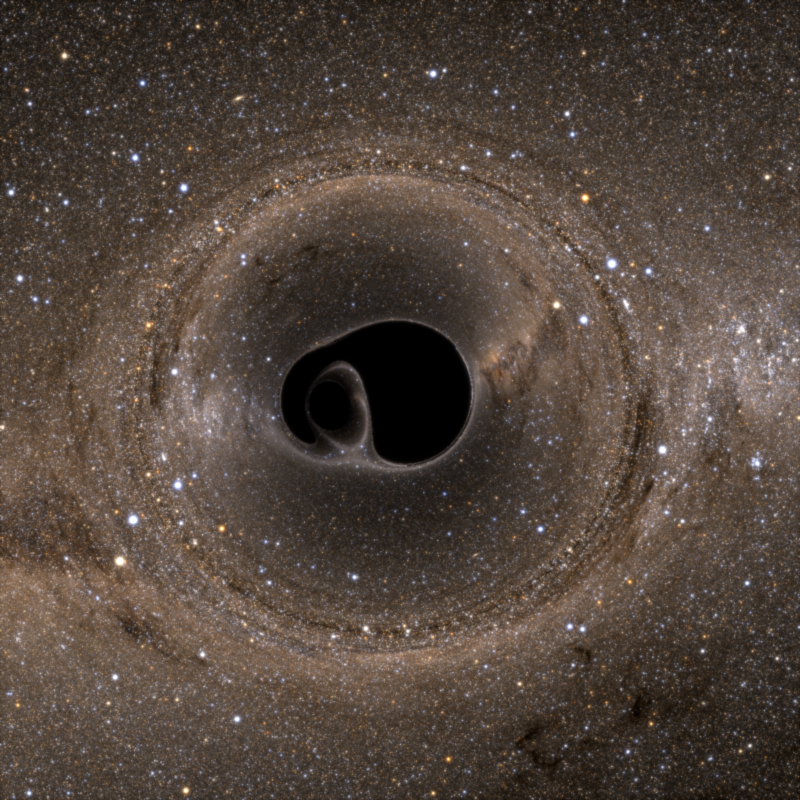
We’ve seen black hole-black hole mergers already, and as LIGO continues to improve, we can reasonably expect to make the first population estimates of stellar mass black holes (from a few to maybe 100 solar masses) over the next few years. LIGO is also highly anticipating finding neutron star-neutron star mergers; when it reaches the designed sensitivity, it may see up to three or four of these events each month if our estimates of their merger rates and LIGO’s sensitivity are correct. This could teach us the origin of short-period gamma ray bursts, which are suspected to be merging neutron stars, but this has never been confirmed.
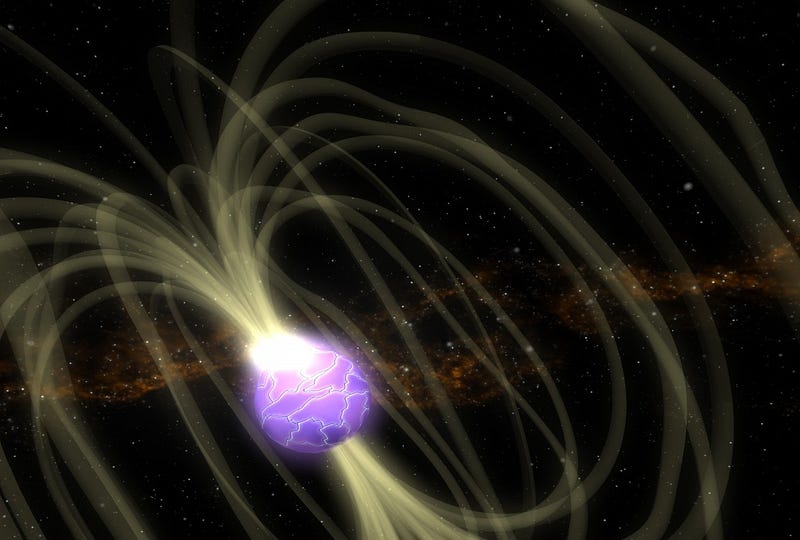
Asymmetric supernovae and exotic neutron star quakes are fun, if perhaps rare phenomena, but it’s exciting to have a shot at studying these in a new way. But the biggest new advances will come when more detectors are built. When the VIRGO detector in Italy comes online, it will finally be possible to do true position triangulation: to locate exactly where in space these gravitational wave events are originating, making follow-up optical measurements possible for the first time. With additional new gravitational wave interferometers scheduled to be built in Japan and India, our coverage of the gravitational wave sky is slated to improve rapidly in the next few years.
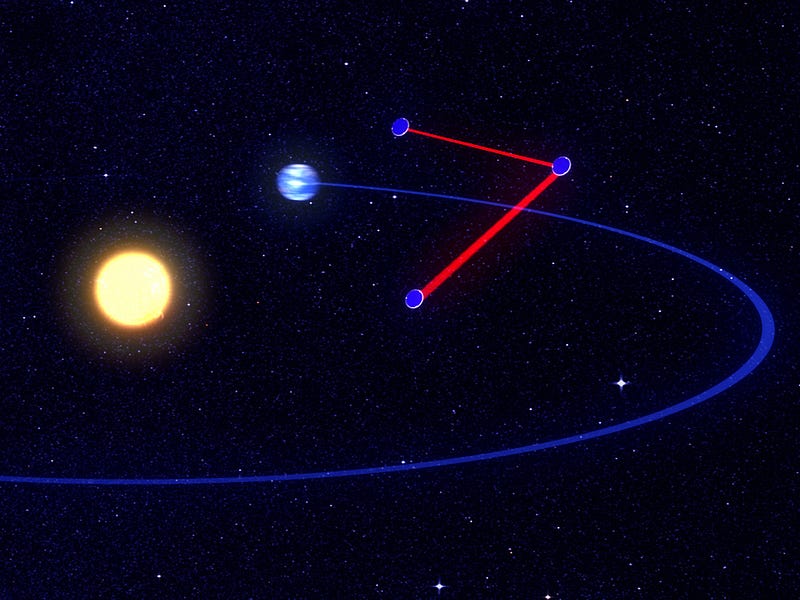
But the biggest advances will come from taking our gravitational wave ambitions into space. In space, you’re not limited by seismic noise, rumbling trucks or plate tectonics; you have the quiet vacuum of space as your backdrop. You’re not limited by the curvature of the Earth for how long you can build your gravitational wave observatory’s arms; you can put it in orbit behind the Earth, or even in orbit around the Sun! Instead of milliseconds, we can measure objects with periods of seconds, days, weeks or even longer. We’ll be able to detect the gravitational waves from supermassive black holes, including from some of the largest known objects in the entire Universe.
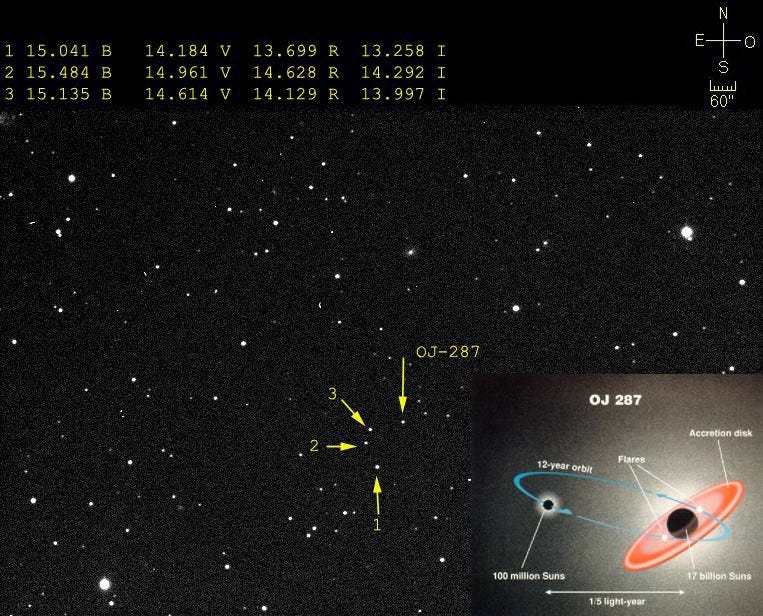
And finally, if we build a large enough, sensitive enough space observatory, we could see the leftover gravitational waves from before the Big Bang itself. We could directly detect the gravitational perturbations from cosmic inflation, and not only confirm our cosmic origin in a whole new way, but simultaneously prove that gravitation itself is a quantum force in nature. After all, these inflationary gravitational waves can’t be generated unless gravitation itself is a quantum field. The success of LISA Pathfinder more than proves this is possible; all it takes is the right investment.
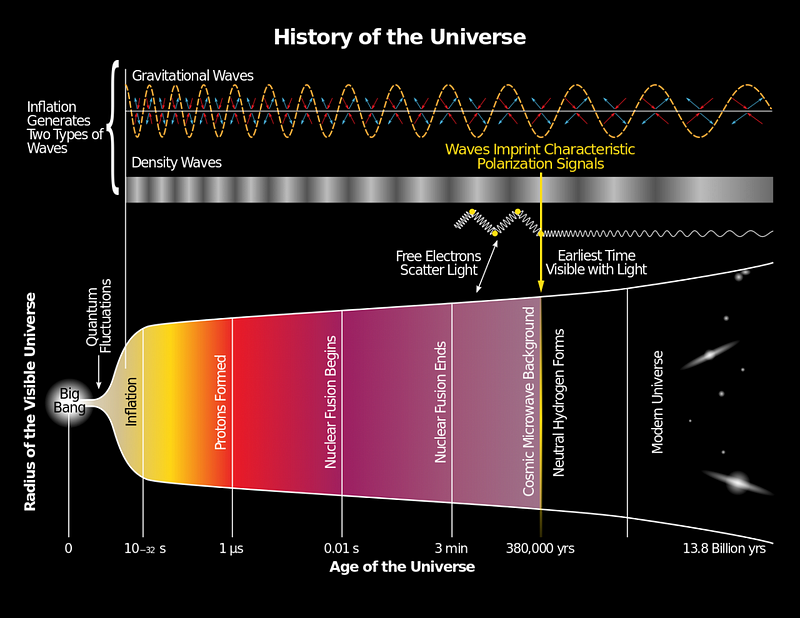
There’s currently a hotly contested race as to what will be chosen as the flagship NASA mission of the 2030s. Although many groups are proposing good missions, the biggest dream is a space-based, gravitational wave observatory in orbit around the Sun. A series of these could make our wildest gravitational wave dreams come true. We have the technology; we’ve proved the concept; we know the waves are there. The future of gravitational wave astronomy is limited only by what the Universe itself gives us, and how much we choose to invest in it. But this new era has already dawned. The only question is how bright this new field in astronomy is going to be. And that part of it is completely up to us.
This post first appeared at Forbes, and is brought to you ad-free by our Patreon supporters. Comment on our forum, & buy our first book: Beyond The Galaxy!