What if cosmic inflation is wrong?
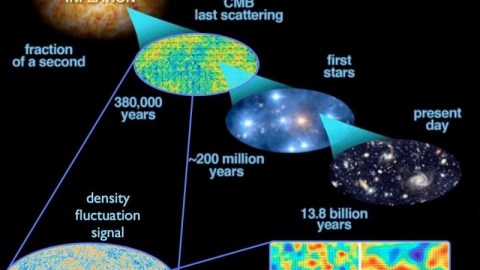
One of inflation’s co-founders lashes out against the community. But is there a scientific leg to stand on?
“…an understanding of the infinite tree of universes seems to be needed in order to make statistical predictions about the properties of our own universe, which is assumed to be a typical “branch” on the tree.” –Alan Guth
All scientific ideas, no matter how accepted or widespread they are, are susceptible to being overturned. For all the successes any idea may have, it only takes one experiment or observation to falsify it, invalidate it, or necessitate that it be revised. Beyond that, every scientific idea or model has a limitation to its range of validity: Newtonian mechanics breaks down close to the speed of light; General Relativity breaks down at singularities; evolution breaks down when you reach the origin of life. Even the Big Bang has its limitations, as there’s only so far back we can extrapolate the hot, dense, expanding state that gave rise to what we see today. Since 1980, the leading idea for describing what came before it has been cosmic inflation, for many compelling reasons. But recently, a spate of public statements has shown a deeper controversy:
- In February, a group of theorists, including one of inflation’s co-founders, claimed that inflation had failed.
- The mainstream group of inflationary cosmologists, including inflation’s inventor, Alan Guth, wrote a rebuttal.
- This prompted the original group to dig in further, denouncing the rebuttal.
- And earlier this week, a major publication and one of the rebuttal’s co-signers highlighted and gave their perspective on the debate.
There are three things going on here: the problems with the Big Bang that led to the development of cosmic inflation, the solution(s) that cosmic inflation provides and generic behavior, and subsequent developments, consequences, and difficulties with the idea. Is that enough to cast doubt on the entire enterprise? Let’s lay it all out for you to see.
Ever since we first recognized that there are galaxies beyond our own Milky Way, all the indications have shown us that our Universe is expanding. Because the wavelength of light is what determines its energy and temperature, then the fabric of expanding space stretches those wavelengths to be longer, causing the Universe to cool. If the Universe is expanding and cooling as we head into the future, then that means it was closer together, denser, and hotter in the past. As we extrapolate farther and farther back, the hot, dense, uniform Universe tells us a story about its past.
We arrive at a point where galaxy clusters, individual galaxies or even stars haven’t had time to form due to the influence of gravity. We can go even earlier, where the amount of energy in particles and radiation make it impossible for neutral atoms to form; they’d immediately be blasted apart. Even earlier, and atomic nuclei are blasted apart, preventing anything more complex than a proton or neutron from forming. Even earlier, and we begin creating matter/antimatter pairs spontaneously, due to the high energies present. And if you go all the way back, as far as your equations can take you, you’d arrive at a singularity, where all the matter and energy in the entire Universe were condensed into a single point: a singular event in spacetime. That was the original idea of the Big Bang.
If that were the way things worked, there would be a number of puzzles based on the observations we had.
- Why would the Universe be the same temperature everywhere? The different regions of space from different directions wouldn’t have had time to exchange information and thermalize; there’s no reason for them to be the same temperature. Yet the Universe, everywhere we looked, had the same background 2.73 K temperature.
- Why would the Universe be perfectly spatially flat? The expansion rate and the energy density are two completely independent quantities, yet they must be equal to one part in 1024 in order to produce the flat Universe we have today.
- Why are there no leftover high-energy relics, as practically every high-energy theory predicts? There are no magnetic monopoles, no heavy, right-handed neutrinos, no relics from grand unification, etc. Why not?
In 1979, Alan Guth had the idea that an early phase of exponential expansion preceding the hot Big Bang could solve all of these problems, and would make additional predictions about the Universe that we could go and look for. This was the big idea of cosmic inflation.
This type of expansion, exponential expansion, is different from what happened for the majority of the Universe’s history. When your Universe is full of matter and radiation, the energy density drops as the Universe expands. As the volume expands, the density goes down, and so the expansion rate goes down, too. But during inflation, the Universe is filled with energy inherent to space itself, so as the Universe expands, it simply creates more space, and that keeps the density the same, and prevents the expansion rate from dropping. This, all at once, solves the three puzzles as follows:
- The Universe is the same temperature everywhere today because disparate, distant regions were once connected in the distant past, before the exponential expansion drove them apart.
- The Universe is flat because inflation stretched it to be indistinguishable from flat; the part of the Universe that’s observable to us is so small relative to how much inflation stretched it that it’s unlikely to be any other way.
- And the reason there are no high-energy relics is because inflation pushed them away via the exponential expansion, and then when inflation ended and the Universe got hot again, it never achieved the ultra-high temperatures necessary to create them again.
By the early 1980s, not only did inflation solve those puzzles, but we also began coming up with models that successfully recovered a Universe that was isotropic (the same in all directions) and homogeneous (the same in all location), consistent with all our observations.
These predictions are interesting, but not enough, of course. For a physical theory to go from interesting to compelling to validated, it needs to make new predictions that can then be tested. It’s important not to gloss over the fact that these early models of inflation did exactly that, making six important predictions:
- The Universe should be perfectly flat. Yes, that was one of the original motivations for it, but at the time, we had very weak constraints. 100% of the Universe could be in matter and 0% in curvature; 5% could be matter and 95% could be curvature, or anywhere in between. Inflation, quite generically, predicted that 100% needed to be “matter plus whatever else,” but curvature should be 0%. This prediction has been validated by our ΛCDM model, where 5% is matter, 27% is dark matter and 68% is dark energy; curvature is still 0%.
- There should be an almost scale-invariant spectrum of fluctuations. If quantum physics is real, then the Universe should have experienced quantum fluctuations even during inflation. These fluctuations should be stretched, exponentially, across the Universe. When inflation ends, these fluctuations should get turned into matter and radiation, giving rise to overdense and underdense regions that grow into stars and galaxies, or great cosmic voids. Because of how inflation proceeds in the final stages, the fluctuations should be slightly greater on either small scales or large scales, depending on the model of inflation. For perfect scale invariance, a parameter we call n_s would equal 1 exactly; n_s is observed to be 0.96.
- There should be fluctuations on scales larger than light could have traveled since the Big Bang. This is another consequence of inflation, but there’s no way to get a coherent set of fluctuations on large scales like this without something stretching them across cosmic distances. The fact that we see these fluctuations in the cosmic microwave background and in the large-scale structure of the Universe — and didn’t know about them in the early 1980s — further validates inflation.
- These quantum fluctuations, which translate into density fluctuations, should be adiabatic. Fluctuations could have come in different types: adiabatic, isocurvature, or a mixture of the two. Inflation predicted that these fluctuations should have been 100% adiabatic, which should leave unique signatures in both the cosmic microwave background and the Universe’s large-scale structure. Observations bear out that yes, in fact, the fluctuations were adiabatic: of constant entropy everywhere.
- There should be an upper limit, smaller than the Planck scale, to the temperature of the Universe in the distant past. This is also a signature that shows up in the cosmic microwave background: how high a temperature the Universe reached at its hottest. Remember, if there were no inflation, the Universe should have gone up to arbitrarily high temperatures at early times, approaching a singularity. But with inflation, there’s a maximum temperature that must be at energies lower than the Planck scale (~1019 GeV). What we see, from our observations, is that the Universe achieved temperatures no higher than about 0.1% of that (~1016GeV) at any point, further confirming inflation.
- And finally, there should be a set of primordial gravitational waves, with a particular spectrum. Just as we had an almost perfectly scale-invariant spectrum of density fluctuations, inflation predicts a spectrum of tensor fluctuations in General Relativity, which translate into gravitational waves. The magnitude of these fluctuations are model-dependent on inflation, but the spectrum has a set of unique predictions. This sixth prediction is the only one that has not been verified observationally.
So inflation has a tremendous number of successes to its name. But since the late 1980s, theorists have spent a lot of time cooking up a variety of inflationary models. They’ve found some incredibly odd, non-generic behavior in some of them, including exceptions that break some of the predictive rules, above. In general, the simplest inflationary models are based on a potential: you draw a line with a trough or well at the bottom, the inflationary field starts off at some point away from that bottom, and it slowly rolls down towards the bottom, resulting in inflation until it settles at its minimum. Quantum effects play a role in the field, but eventually, inflation ends, converting that field energy into matter and radiation, resulting in the Big Bang.
But you can make multi-field models, fast-roll models instead of slow-roll models, contrived models that have large departures from flatness, and so on. In other words, if you can make the models as complex as you want, you can find one that gives departures from the generic behavior described above, sometimes even resulting in departures from one or more of these six predictions.
This is what the current controversy is all about! One side goes so far as to claim that because you can contrive models that can give you almost arbitrary behavior, inflation fails to rise to the standard of a scientific theory. The other side claims that inflation makes these generic, successful predictions, and that the better we measure these parameters of the Universe, the more we constrain which models are viable, and the closer we come to understanding which one(s) best describe our physical reality.
The facts that no one disputes are that without inflation, or something else that’s very much like inflation (stretching the Universe flat, preventing it from reaching high energies, creating the density fluctuations we see today, causing the Universe to begin at the same temperatures everywhere, etc.), there’s no explanation for the initial conditions the Universe starts off with. Alternatives to inflation have that hurdle to overcome, and right now there is no alternative that has displayed the same predictive power that the inflationary paradigm brings. That doesn’t mean that inflation is necessarily right, but there sure is a lot of good evidence for it, and many of the “possible” models that can be concocted have already been ruled out. Until an alternative model can achieve all of inflation’s successes, cosmic inflation will remain the leading idea for where our hot Big Bang came from.
Ethan Siegel is the author of Beyond the Galaxy and Treknology. You can pre-order his third book, currently in development: the Encyclopaedia Cosmologica.