What Happened Before The Big Bang?
We normally think of the Big Bang as the very beginning of our Universe, but we now know the story goes back even before it. Here’s how.
“The aim of science is not to open the door to infinite wisdom, but to set a limit to infinite error.” –Bertolt Brecht
One of the most frequent questions I get about the Universe — as a cosmologist — isn’t quite about the Big Bang in and of itself.
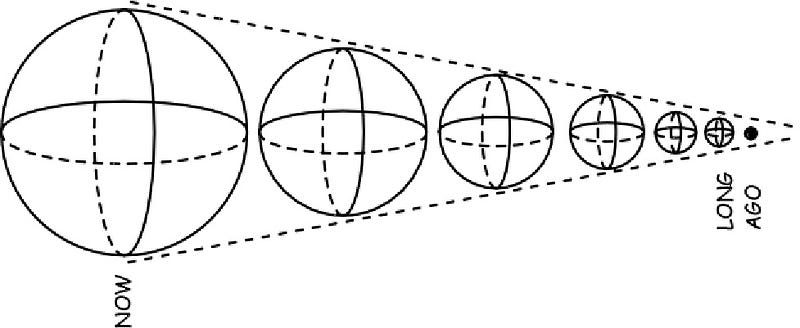
The Big Bang is a remarkable idea, of course, that says that, based on the observations that the Universe is expanding and cooling today, it was hotter, denser, and physically smaller in the past. This gets particularly exciting when we extrapolate very far back in the history of the Universe.
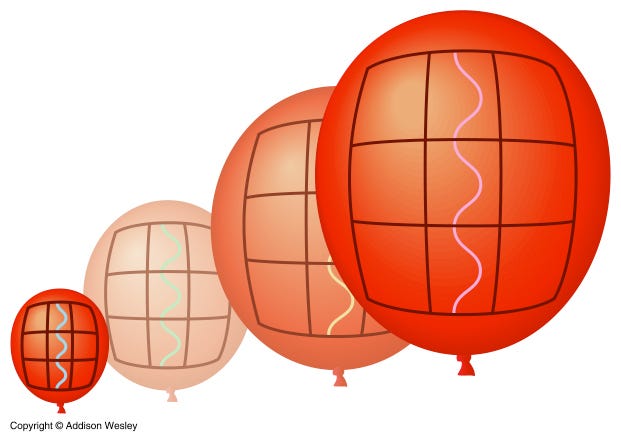
At some point in the past, it was so hot that individual atoms would have been blasted apart by the radiation in the Universe. This means that — as we come forward in time past that point — there was a point when all the nuclei and electrons in the Universe became stable, neutral atoms for the first time.
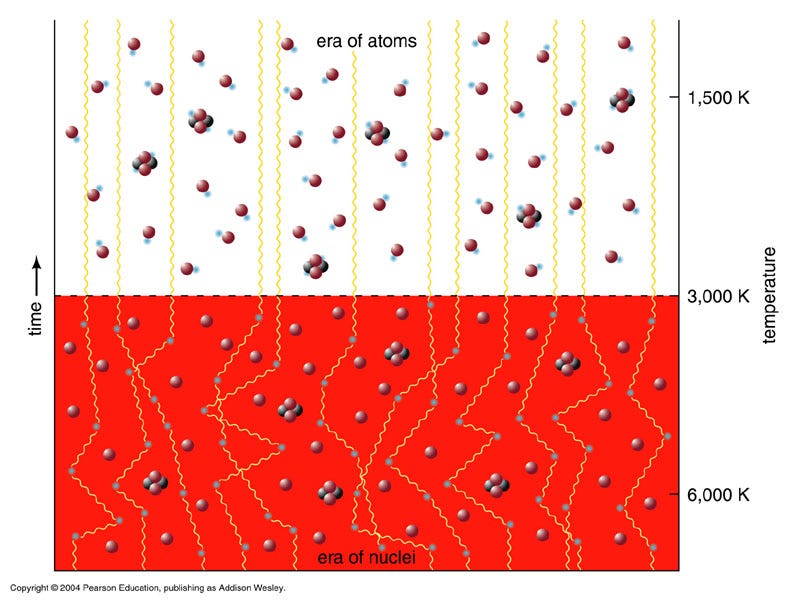
And before that, it was so hot that individual nuclei would have been blasted apart. But you might think that this means we can go back to arbitrarily high temperatures, densities, and arbitrarily small sizes. You might be tempted to go all the way to a point in time where spacetime collapses into a singularity, and where all the matter and energy in the Universe were present at a single point, of infinite temperature and infinite density.
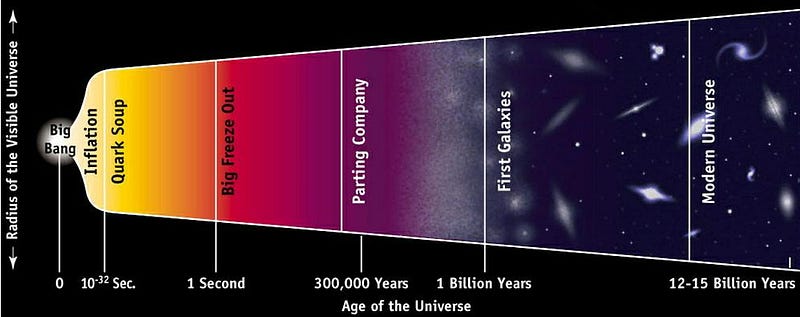
Indeed, this is one of the most tempting things to try, and it’s what our picture of the Universe used to be, prior to about 1980.
But physically, it’s also incomplete. (Lots of good scientists and science institutions goof this, too. See here, for example, or even more recently, this piece.) You see, we know that this isn’t what happened in the Universe’s past, because of what we observe when we look — in detail — at a snapshot of the Universe’s early history, from back when those neutral atoms formed for the first time.
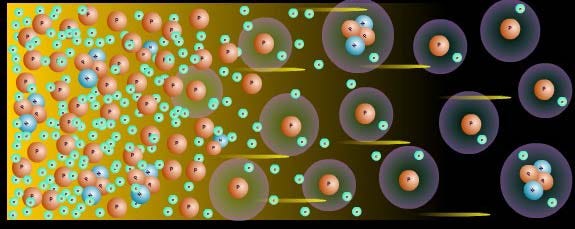
What we learn is that there’s an upper limit to how hot the Universe ever was in its early history. And although it may have been very hot — up to energies between 10^16 and 10^17 GeV, or about 10 trillion times higher in energy than the Large Hadron Collider can create — that’s actually quite small in some capacity. Even ~10^16-17 GeV is small compared to the scale where we’d need to talk about singularities (which is another factor of ~1,000 hotter), or where quantum gravity/string theory effects would become important: that’s the scale where our conventional laws of physics (General Relativity and Quantum Field Theory) break down.
We learn this from looking at the magnitude and distribution of the temperature fluctuations in the Universe imprinted in the snapshot alluded to earlier: in the Cosmic Microwave Background.
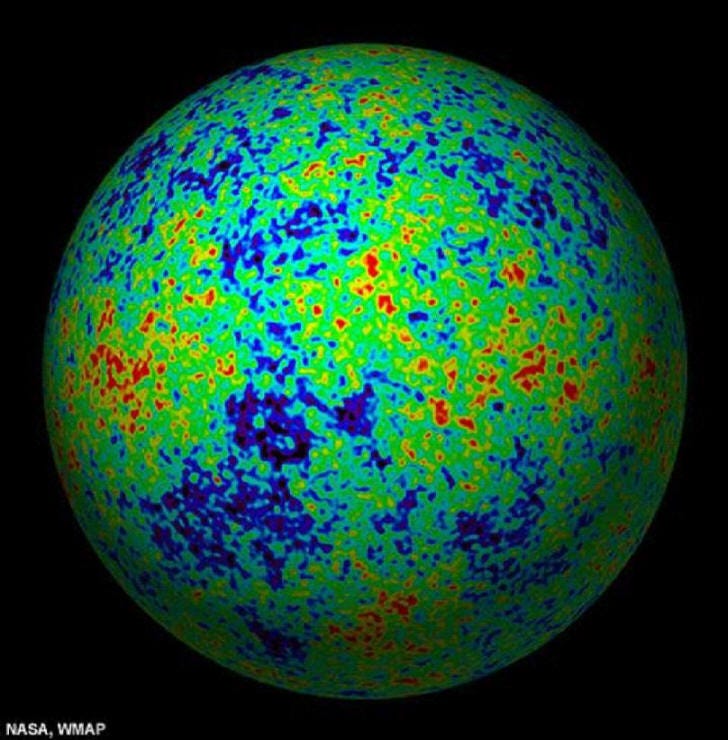
(If you prefer a Mercator projection — the way you typically see a map of Earth — click here.)
What these fluctuations tell us is that, at some point in the very early history of the Universe — where we can be accurately described by this hot, dense, radiation-filled, Big Bang-esque model — the Universe was filled with small-magnitude temperature fluctuations (of a few parts in 100,000) on all measurable scales, where each scale is observed to have the same-magnitude pattern of fluctuations.
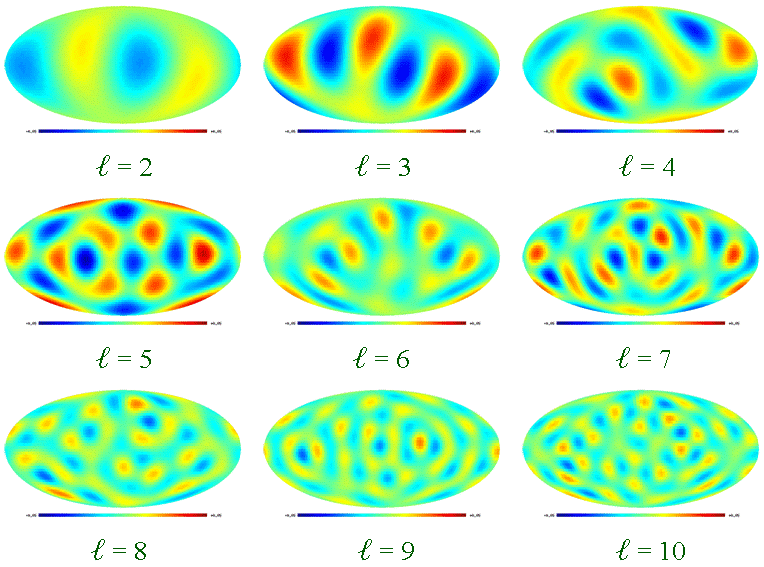
As the Universe expands and cools, gravity works to pull the matter and energy in on itself, making overdensities bigger and underdensities smaller, while radiation pressure works to wash those fluctuations out. Normal matter (protons, neutrons, and electrons) interacts with photons and itself, creating “bouncy” features in this pattern of fluctuations, while dark matter can feel the radiation pressure and the gravitational tugs, but has no cross-section with either normal matter, photons or itself.
As a result, we learn what the different components of the Universe are.
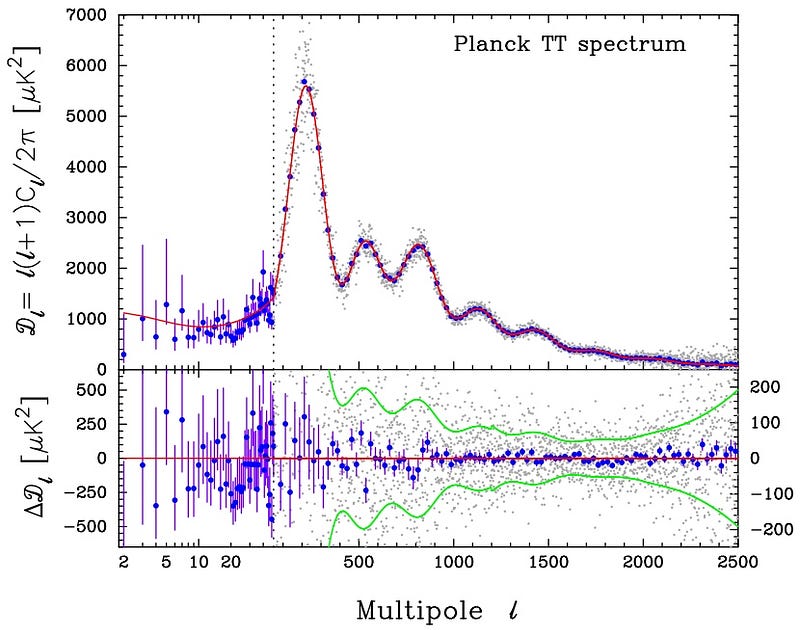
Two important observations that come out of this are that, as far as curvature goes, the Universe is spatially flat, rather than curved positively (like a sphere) or negatively (like the seat of a saddle), and that it has the same temperature properties in all directions, even in regions that have never had an opportunity to exchange information (or transmit photons) between one another.
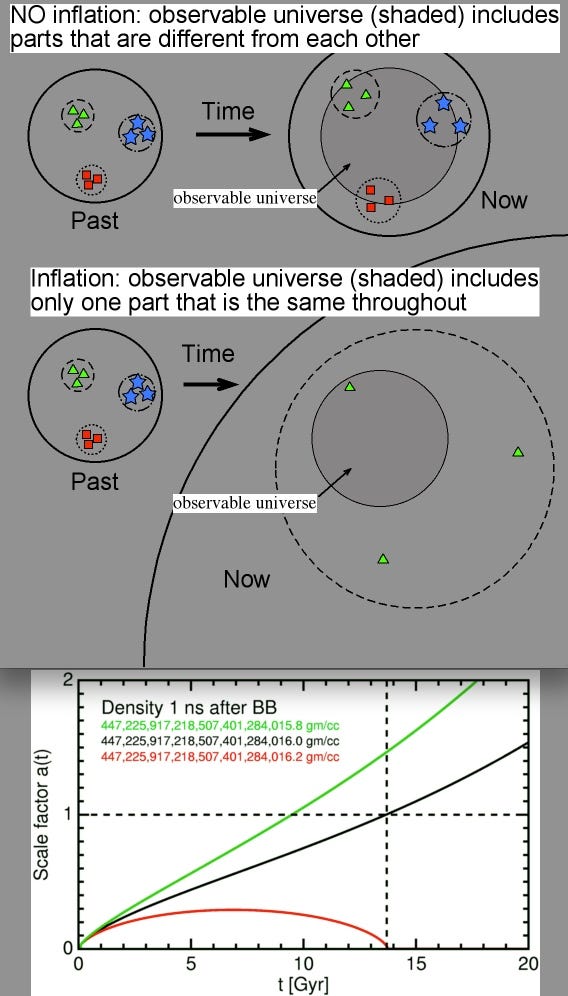
These two things could be remarkable, finely-tuned coincidences (or, you know, just how things happen to be, for no reason), but they could also be indicative of something preceding the Big Bang. In particular, a phase of exponential expansion of the Universe — known as cosmological inflation — would compel these two things to be true.
But cosmic inflation also carries a number of predictions with it: that there would be no magnetic monopoles or other leftover relics from grand unified theories, that there would be no topological defects (e.g., cosmic strings, domain walls) in the large scale structure of the Universe, and that the temperature fluctuations found in the Cosmic Microwave Background would follow a special type of distribution.
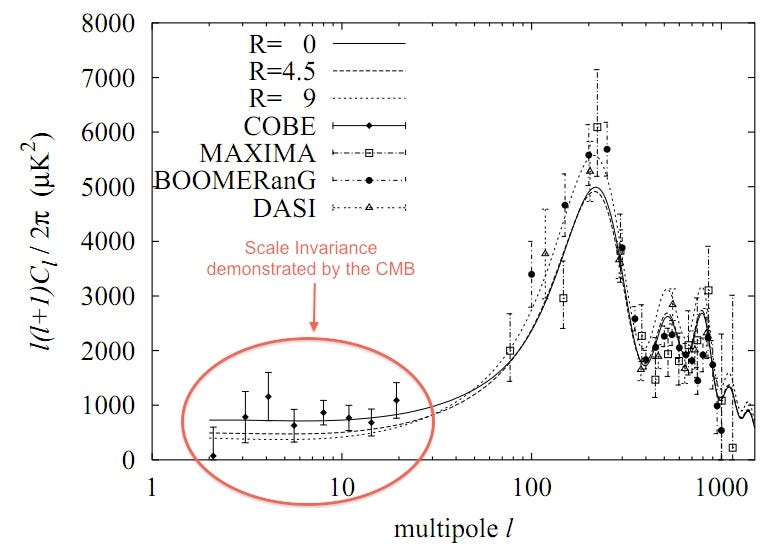
Not only do we find strong evidence against leftover relics and topological defects, but we measured this Harrison-Zel’dovich spectrum very accurately back in the 1990s, which was predicted by inflation more than a decade before it was observed! In other words, the spectrum of fluctuations is precisely consistent with what the theory of cosmological inflation predicted!
What inflation — our best scientific theory as to what preceded the Big Bang — tells us about “what came before the Big Bang” is, perhaps, very surprising.
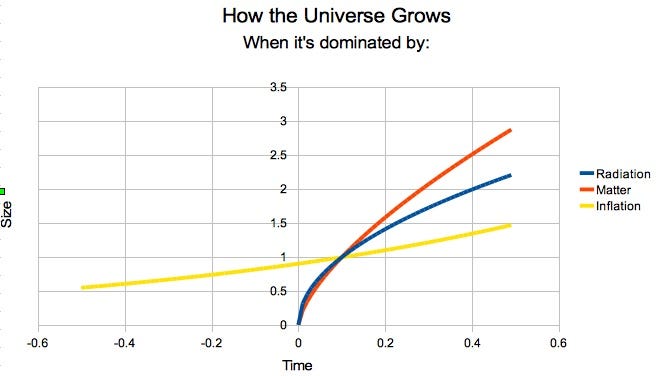
If the Universe was filled with matter (orange) or radiation (blue), as shown above, there must be a point at which these infinite temperatures and densities are reached, and thus, a singularity. But in the case of inflation (yellow), everything changes. First off, we don’t necessarily have a singularity, and we definitely don’t have one at what we traditionally think of as “the moment of the Big Bang.” Instead, we have what’s known as a past-timelike-incomplete spacetime.
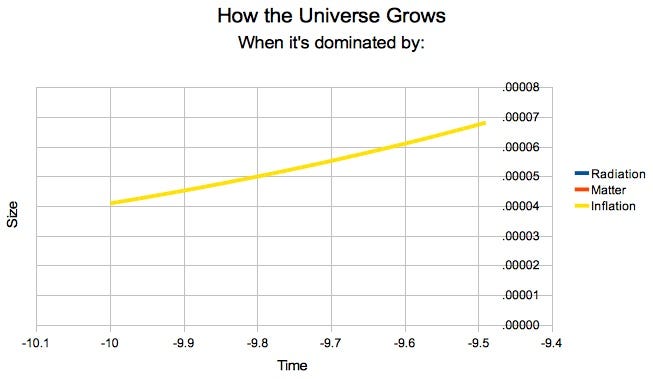
In other words, we not only don’t know whether there was a singularity at some point in the very distant, pre-inflation past, or whether inflation was truly eternal, we don’t even know whether inflation occurred for less than a yoctosecond or more than the present age of the (post-Big Bang) Universe!
Our prospects for finding out, furthermore, are quite dim, as — by its very nature — practically every model of cosmic inflation wipes out any information about the Universe that existed prior to the last billionth-of-a-yoctosecond before inflation ended, and our Universe began.
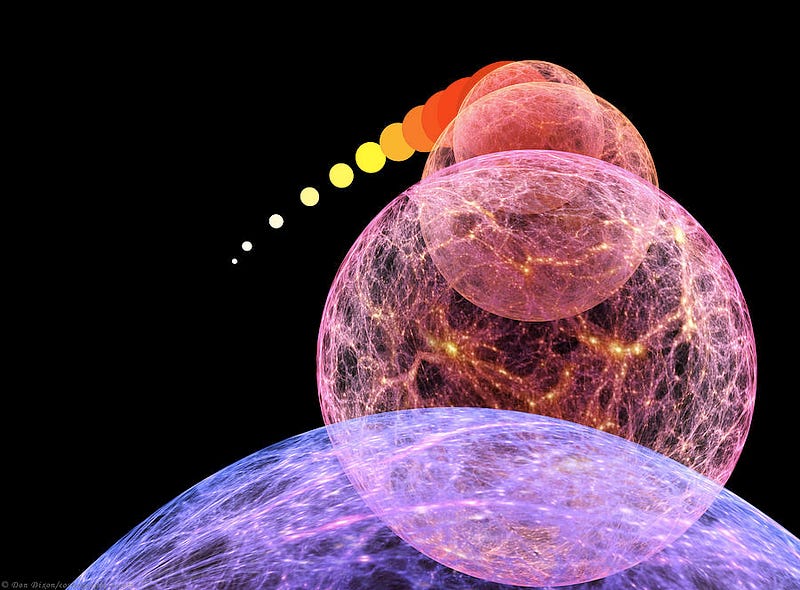
So, before the Universe was hot, dense, expanding, cooling, and filled with matter and antimatter? There was inflation, the phase of exponential expansion that stretched the Universe flat, made it the same average temperature in all directions, wiped out any ultra-massive relic particles and topological defects, created the temperature fluctuations that led to the large-scale structure of today’s Universe, and ended 13.8 billion years ago, setting up the Big Bang that gave rise to the observable Universe we know and love. If inflation lasted any longer than that last billionth-of-a-yoctosecond that affects our observable Universe and the laws of physics we know still hold, then we almost certainly live in a multiverse as well, where our observable Universe is just one Universe out of many.
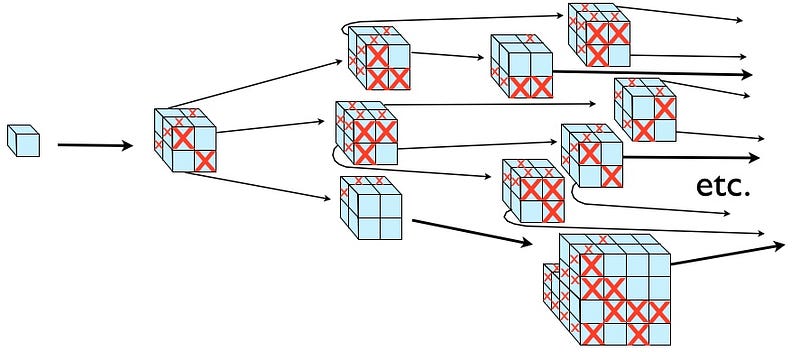
But what came before this inflationary state? We only have theoretical possibilities, with likely no data or information from that time contained within our observable Universe to guide us. It may have been eternal to the past, there may have been a true singularity before it, or there may have been something else; as far as our current observations go, we haven’t even conceived of a way we could possibly know. Inflation comes before the Big Bang, and anyone who tells you differently is more than three decades out of date!
We’ll keep searching for clues as to the nature of inflation and what might have come before it, but for right now, don’t believe the hype. (And I’m looking at you, Steinhardt, Turok, and Greene, among others.) Keep all the options open as possibilities if you fancy them, but know that speculation is no replacement for the best that science has to offer right now!
An earlier version of this post originally appeared on the old Starts With A Bang blog at Scienceblogs.