Throwback Thursday: What is the Weak Force?
And does it have its own type of charge, like all the other forces?
“Time is a sort of river of passing events, and strong is its current; no sooner is a thing brought to sight than it is swept by and another takes its place, and this too will be swept away.” –Marcus Aurelius
Every one of us does our best to come up with an accurate picture of reality, and that includes the Universe, from the smallest subatomic particles to the largest scales fathomable. But given how bizarre and counterintuitive some of our physical laws are — even at a fundamental level — this can be a daunting task for even those of us who are professional theoretical physicists.
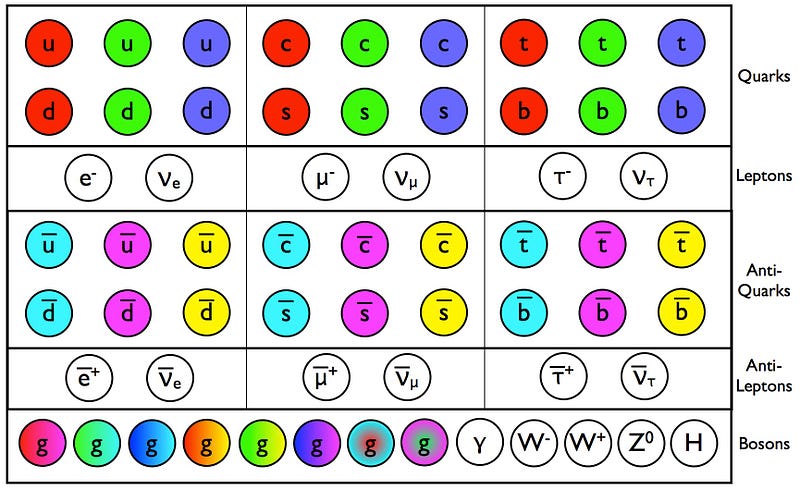
When we colloquially talk about the different forces — the four fundamental ones — here are the things we traditionally have to say about them:
- The gravitational force acts on anything with matter, energy and/or momentum. Space is curved (or gravitons are exchanged, at a quantum level), the force is always attractive, and it affects everything in the Universe.
- The electromagnetic force acts on any particle with an electric charge. Like charges repel one another, opposite charges attract, and electromagnetic forces are mediated by the photon. It occurs over infinite distances, but neutral objects exert no force.
- The strong force acts on any particle with a color charge, which are quarks and gluons exclusively. It holds individual quarks together in baryons (like protons or neutrons) and mesons, and binds atomic nuclei together. It operates over short distances only, falling off rapidly for color-neutral bound states.
- And the weak force… is responsible for radioactive decays. It’s mediated by the W-and-Z bosons, and occurs over incredibly short distances only.
Does it bother you that there’s no description of an interaction here, for the weak force? Of an attraction or repulsion? In other words, it isn’t really described as a force when we talk about it!
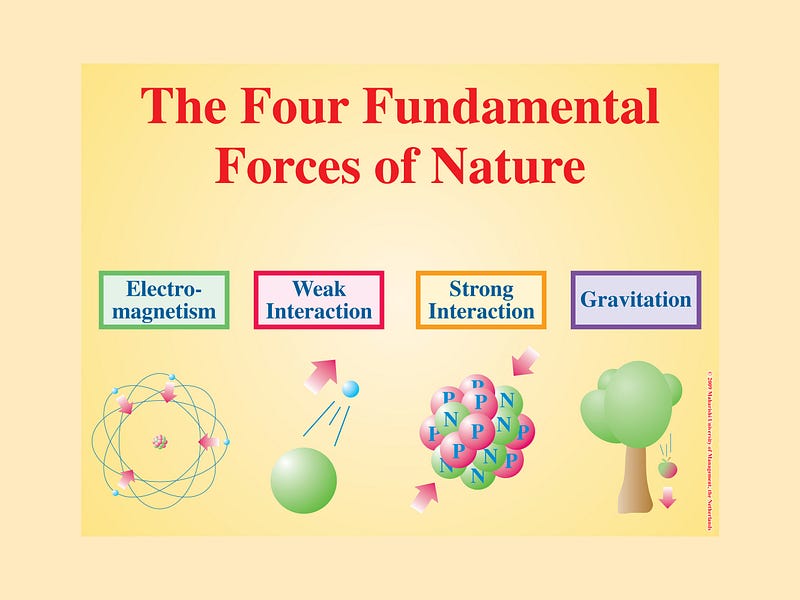
But it should be. Let’s back up for a moment.
These are all forces, although we should clarify what that means. For particles as we can measure them, the application of a force causes that particle’s momentum to change over time: what we commonly refer to as an acceleration in our day-to-day experience.
For three of these forces, it’s pretty straightforward, no matter how you look at it. Let’s go a little bit more in-depth into each one.

In gravitation, the total amount of energy (which is mostly mass in our common experience, but includes all forms of energy) causes spacetime to distort, and every other particle in that Universe — and hence in that distorted spacetime — has its motion altered by the presence of everything with energy. This is, at least, how it works in our classical (non-quantum) theory of gravity.
There may be a more fundamental theory out there, a quantum theory of gravity, in which hypothetical particles known as gravitons are exchanged, causing every particle in the Universe to experience what we perceive as the gravitational force.
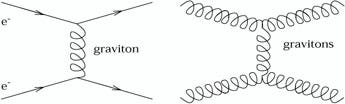
Keep this in mind as we move on to the others:
- Particles have a property, or something inherent to them, that enables them to feel (or not feel) a certain type of force.
- Other particles, force-carrying particles, interact with the ones that have the right properties to experience that force.
- As a result of these interactions, the particles change their momentum, or accelerate, in common parlance.
So let’s look at the others.
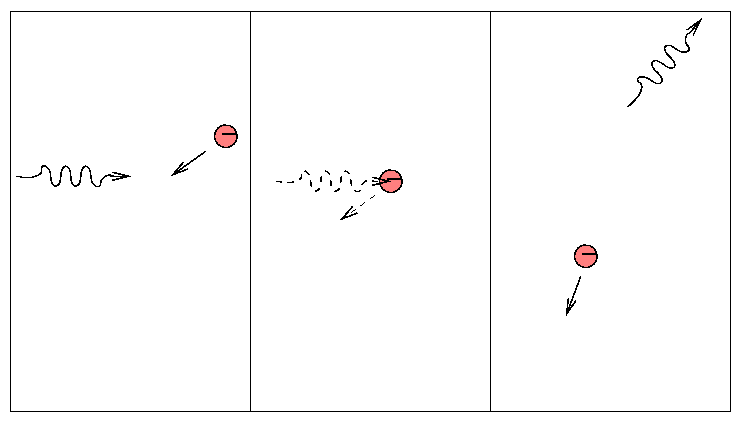
In electromagnetism, the fundamental property is electric charge. Unlike gravity, that charge can be either positive or negative. The photon, which is the force-carrying particle related to charge, causes like charges to repel and opposite charges to attract.
What’s also worth mentioning is that moving charges, or electric currents, experience a different manifestation of the electromagnetic force: magnetism. This happens for gravity, too, and is known as gravitomagnetism. There’s no need to go in-depth on this point, but I do want you to keep in mind that there’s not only a charge and a force carrier, but that there are currents (which arise from moving charges) as well.
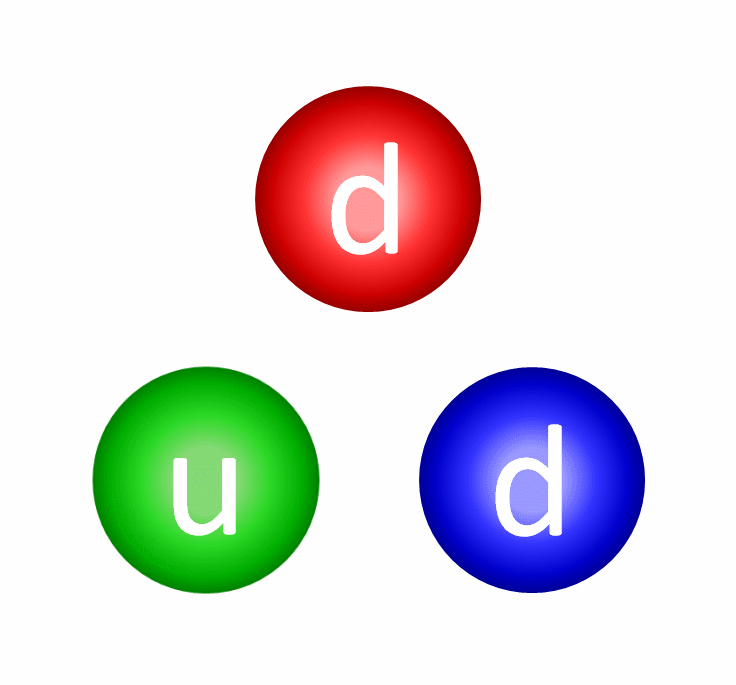
There’s the strong nuclear force, which has three fundamental types of charge, and which you can read about in-depth here. While all particles contain energy (and thus are influenced by gravity), and while the quarks, half of the leptons and a couple of the bosons contain electric charges (and couple to electromagnetism), only the quarks and gluons contain color charge, and can experience the strong nuclear force.
Since there are huge collections of masses, gravity is easy to observe. And since the strong nuclear force and electromagnetism are so incredibly powerful, they’re easy to observe as well.
But what about that last force: the weak force?
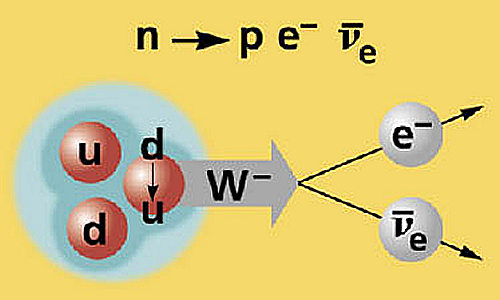
We normally talk about the weak interaction in the context above: some type of radioactive decay. It’s either a heavy quark-or-lepton decaying into lighter, more stable quarks-and-leptons. The weak force certainly does this, among other things. But that doesn’t sound like our other forces, does it?
But it turns out the weak force is really a force, you just don’t hear about it working the conventional way very often… because it’s so weak! In particular, since it normally involves charged particles, it’s typically dwarfed by the electromagnetic force, which is some 10,000,000 times stronger at even the tiny, short distance of a single proton.
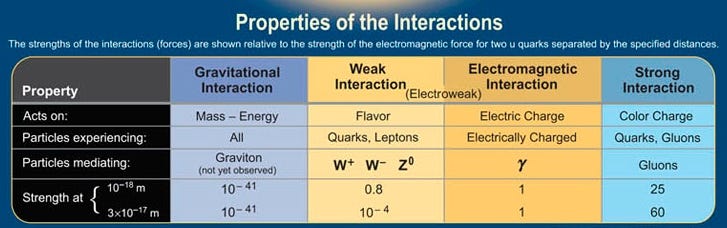
You see, a charged particle always has an electric charge whether it’s moving or not, but the electric current that it makes is dependent on its motion relative to other particles. It’s the current that defines magnetism, which is just as important as the “electric” part of electromagnetism. Composite particles like protons and neutrons have intrinsic magnetic moments, as does the (fundamental) electron.
Well, quarks and leptons each come in six different flavors: up, down, strange, charm, top and bottom for the quarks, and electron / electron-neutrino, muon / mu-neutrino, and tau / tau-neutrino for the leptons. And each one of those quarks-and-leptons has an electric charge associated with it (even though it’s zero for the neutrinos), but they also have a flavor property associated with it. If we unify the electromagnetic and the weak forces (to create a more fundamental electroweak force; see here), then every one of these particles gets something you can think of as a weak charge (or an electroweak current), and a weak coupling constant to go with it. This is something predicted in gory detail by the Standard Model, but that’s been incredibly difficult to test, primarily due to the fact that the electromagnetic force is so much stronger!

But we went from “difficult-to-test” to “we successfully measured it” just two years ago! The critical experiment released their first results (published in PRL; full paper available here) in 2013, and was actually — for the first time — able to measure the impact of the weak force. This is extraordinary, since the strength of this force is just a couple of hundred parts per billion compared to the impact that the electromagnetic force has!
And that allowed the team of experimenters to determine the (dimensionless) weak couplings of the up and down quarks,
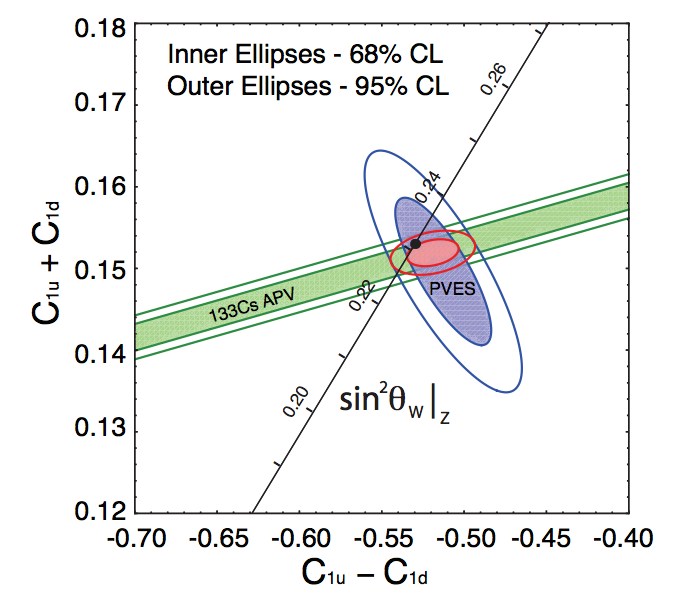
and therefore the weak charges of the proton and neutron. Before I get on to the results, let me tell you what the best Standard Model predictions are for the weak charges:
- Q_W(p) = 0.0710 ± 0.0007,
- Q_W(n) = -0.9890 ± 0.0007.
Well, based on the data they obtained for this high-energy scattering, they were able to experimentally determine that:
- Q_W(p) = 0.063 ± 0.012,
- Q_W(n) = -0.975 ± 0.010.
Which, within the measurement errors, agrees tremendously! Now, they state at the end of their paper that they will eventually have 25 times more data, which means the errors should go down — when all is said and done — by a factor of 5, or the square-root of the amount of data. Instead of errors of ±0.010-to-0.012 on their numbers, they should be able to get down to errors of ± 0.002! And if there are any surprises, or disagreements with the Standard Model, this would be tremendous.
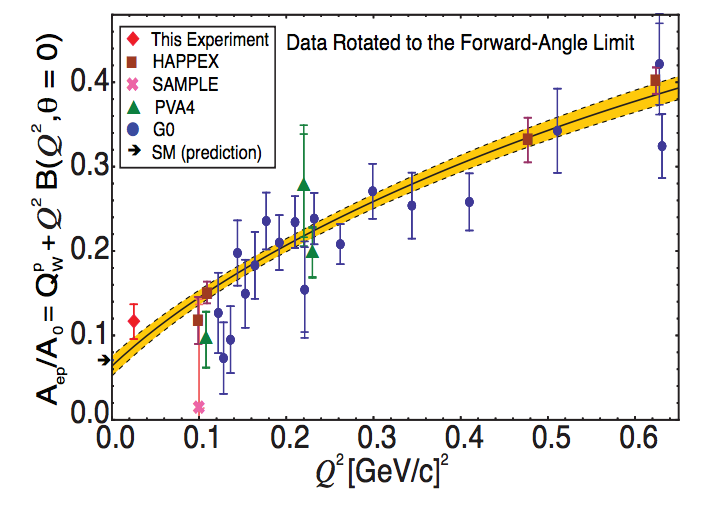
Looking at the preliminary data, though, that sure doesn’t appear likely!
So there is a weak charge associated with particles, we just don’t talk about it because it’s been so mind-numbingly hard to measure. But at long last, we’ve done it, and to the best of our capabilities, we’ve found that the Standard Model is right on!
Leave your comments at our forum, and support Starts With A Bang on Patreon!