Throwback Thursday: What is the Strong Force?
It’s what holds the nuclei in atoms together, overcoming electric repulsion. But how does it work?
“I found I could say things with color and shapes that I couldn’t say any other way — things I had no words for.” –Georgia O’Keeffe
When it comes to the Universe, it isn’t just the stuff that’s in it that’s important. Sure, when it comes to what makes everything up, you want to know about nuclei and electrons, as well as what makes those nuclei up, photons, and any other particle it’s possible to create.
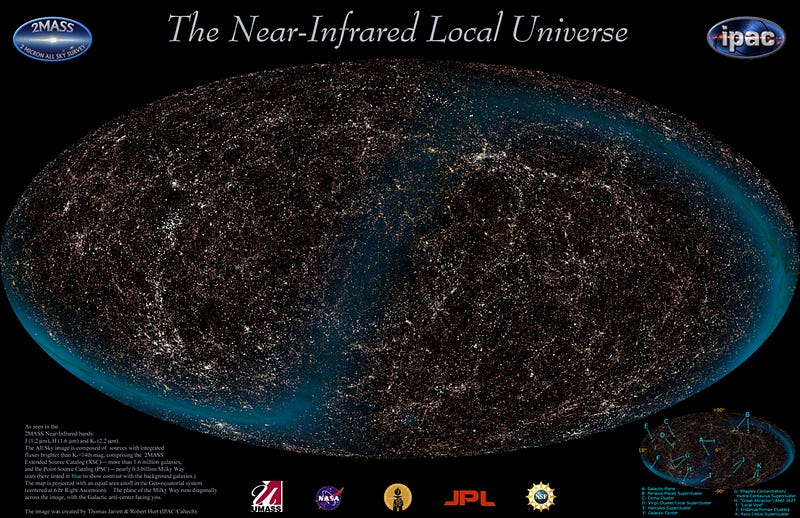
But if you want to really understand it, simply knowing what’s in it isn’t going to get you very far on its own. It’s also how all that stuff interacts with itself and everything else. To the best of our knowledge, there are four fundamental forces in the Universe, and they’re all absolutely essential to our existence.
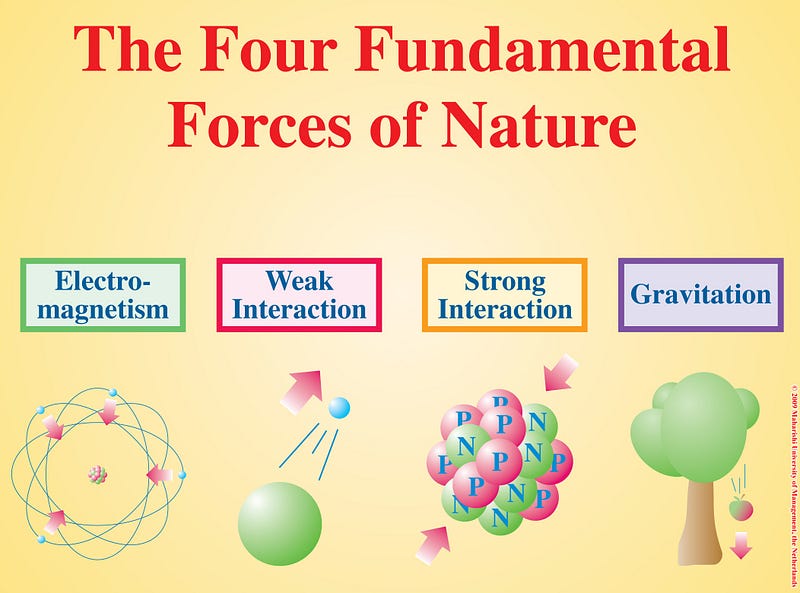
Some of them are familiar, like gravitation. On the largest scales in the Universe, gravitation is not only the most important force, but arguably the only important force in play. The amount of mass and energy inherent to objects determines how spacetime itself is curved, and this curvature of spacetime in turn determines how objects move and accelerate.
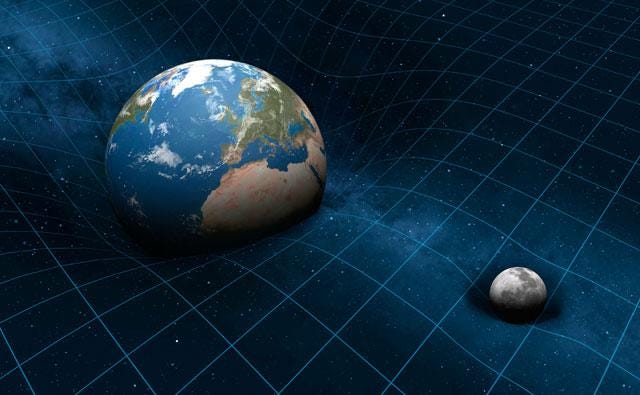
There’s no “anti-mass” or “anti-energy” that causes some objects to be gravitationally repelled while others are gravitationally attracted. Gravity is always attractive, and we can interpret mass/energy as the lone type of gravitational charge, if we want to.
But other forces and interactions can be more complicated than gravity in this regard. Take the electromagnetic force, for example, or the forces that occur when we examine charged particles.
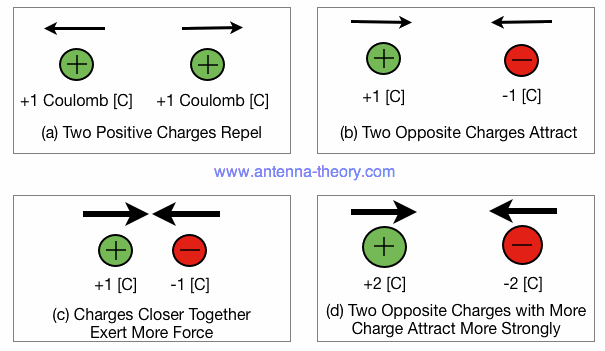
Instead of one type of charge where like-attracts-like, we have two types of electric charge: positive and negative, where like charges repel and unlike charges attract. It’s very different than gravity and somewhat more complicated, but there are some applications of this that ought to feel very familiar. We make extraordinary use of this feature, after all, in practically everything we do on Earth.
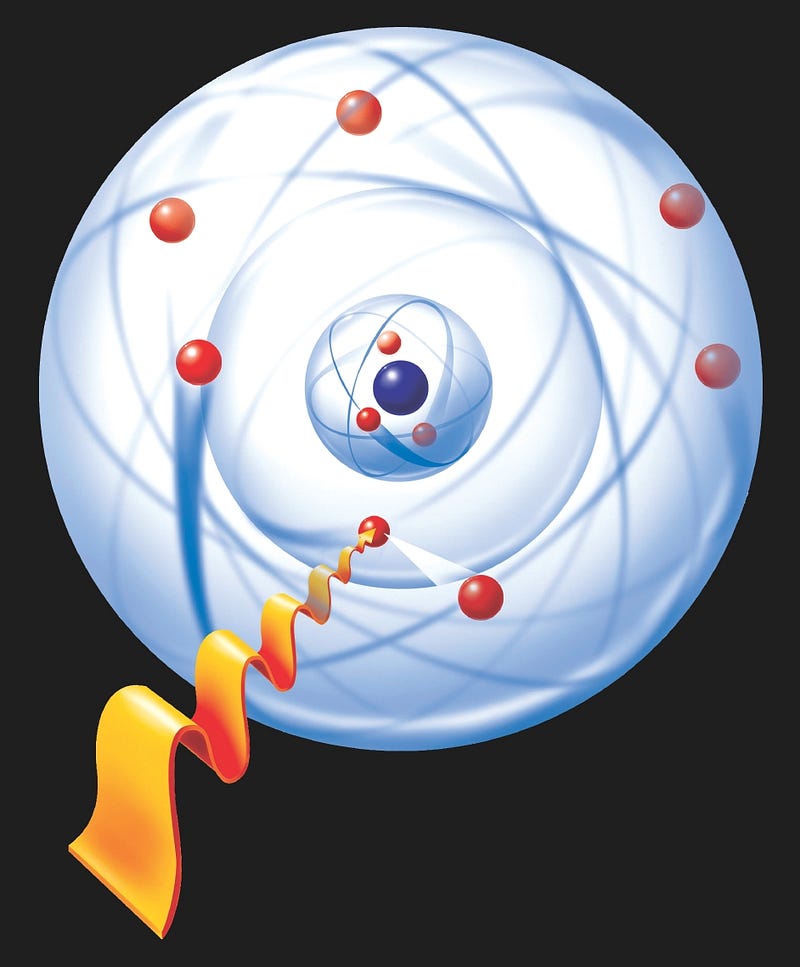
A neutral atom, for instance, is a good example of electromagnetism, where the positively charged nucleus is orbited by a swarm of negatively charged electrons. The electrons repel each other, but they’re all attracted to the nucleus even moreso. As long as the total charge of the atom is zero and there is no sufficiently strong external radiation, the atom will remain stable and neutral. This is the fundamental building block of every thing — living and non-living — on our world.
We even understand, at a fundamental quantum level, how this works. The attraction and repulsion between all charged particles is mediated by the same particle: the photon.
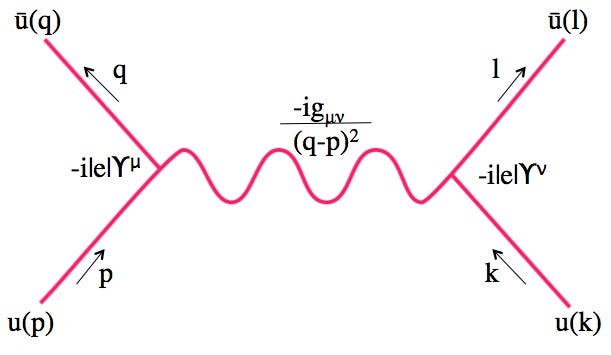
It just takes one particle to take care of both attraction and repulsion, because of the relatively simple structure — two charges, like-repels-like and opposites attract — of electromagnetism. But things get a lot more complicated if we go inside the nucleus, and ask just how it is, at a fundamental level, that these tiny, charged structures hold themselves together.
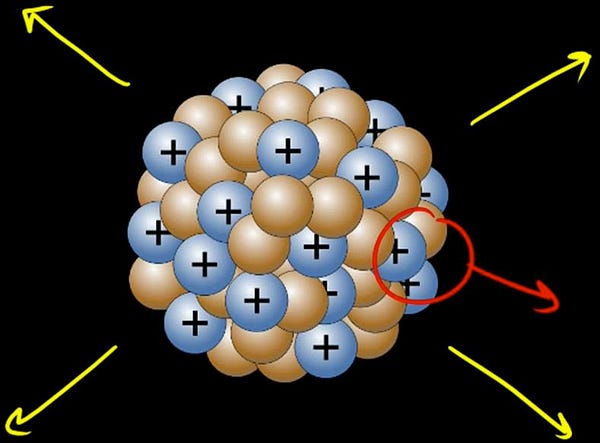
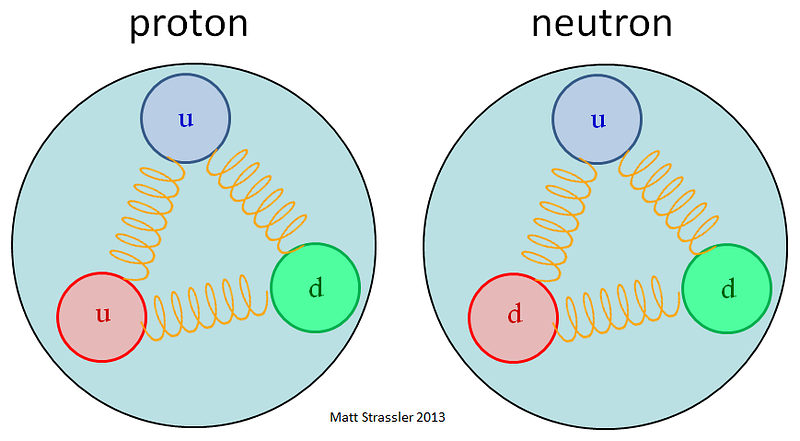
An atomic nucleus, of course, is made up of protons and neutrons, except for hydrogen, which is just a proton by itself. But seeing as how protons have a positive electric charge and neutrons have no electric charge at all, there must be some sort of extra force — a force even stronger than the electromagnetic force — to hold these nuclei together. Otherwise, anything made out of more than one proton (anything other than hydrogen) would push itself apart.
In fact, the creatively-named strong force is required to hold even the individual protons and neutrons themselves together. Because a proton and neutron themselves are not fundamental, but composed of even smaller, fractionally-charged particles known as quarks.
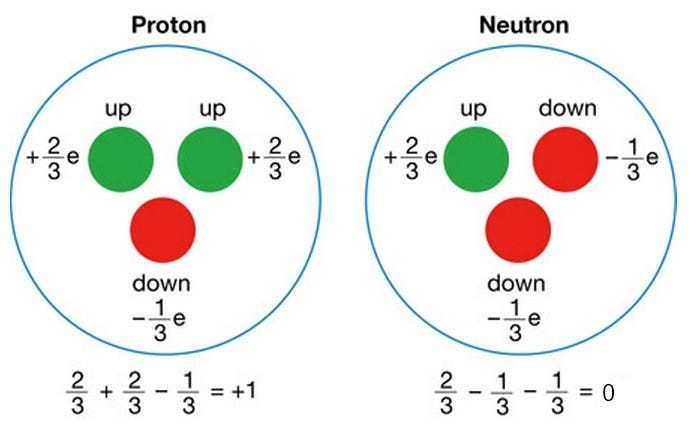
The electric forces inside of a proton, for instance, would cause the nucleus itself to fly apart if there weren’t another type of charge attached to each of these quarks: in addition to electric charge, they also possess color charge, which comes in not one type (like gravity), nor two (like electromagnetism), but three.
Only unlike gravitation and electromagnetism, you can’t just have a color charge off by itself: you need a red, green, and blue together, to add up to “colorless,” just like red, green, and blue light together add up to white.
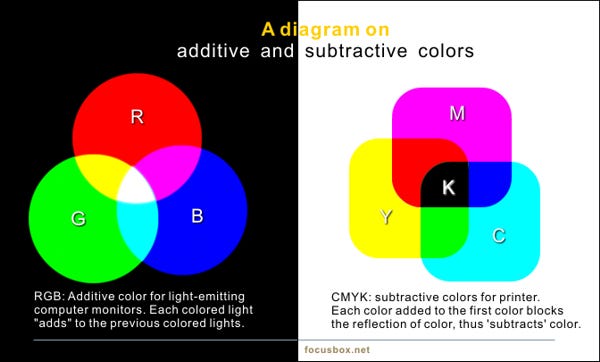
Just like there’s matter and antimatter, there are quarks and anti-quarks, and so there are colors (red, green and blue) and anti-colors: cyan is the anti-red, magenta is the anti-green, and yellow is the anti-blue. So to add up to “colorless,” you either need three quarks (or three anti-quarks), or one quark and one anti-quark.
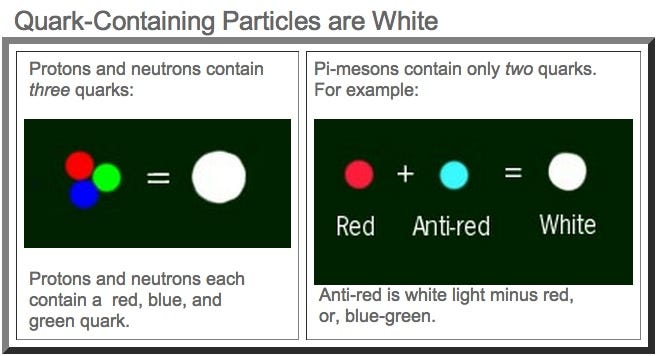
It’s a little bit weird: if red + green + blue makes white, but red + anti-red also makes white, does that mean that green+blue is the same as anti-red? Yes, yes it does, at least in terms of color. Which means you can pair a quark with either two other quarks, with an antiquark, or possiblyeven with three other quarks and one antiquark. As long as the color comes out white (or colorless), you’re in business.
And that’s why you can have combinations of three quarks, like protons and neutrons, or combinations of one quark and one anti-quark, like mesons. But unlike gravity, which bends spacetime, or electromagnetism, where photons (with no charge) are exchanged, the strong force works by exchanging a new type of particle — the gluon — which carries both a color and an anti-color!
These gluons are responsible for holding both individual particles — like protons, neutrons, and pions — together, as well as for binding larger atomic nuclei together.
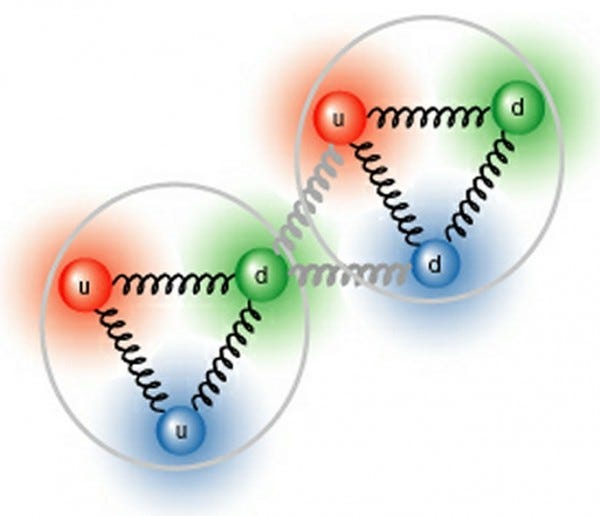
How does this work? With three colors (red, green, and blue) and three anti-colors (anti-red = cyan, anti-green = magenta, and anti-blue = yellow), you might think there are nine types of gluons that you can get from matching each color with each anti-color. That’s a good first thought, and it’salmost right.
Imagine you’re a red quark, and you emit a red/anti-green gluon. You’re going to turn the red quark into a green quark, because color is conserved like that, and then that gluon is going to find a green quark, and turn it red. In this fashion, colors get exchanged.
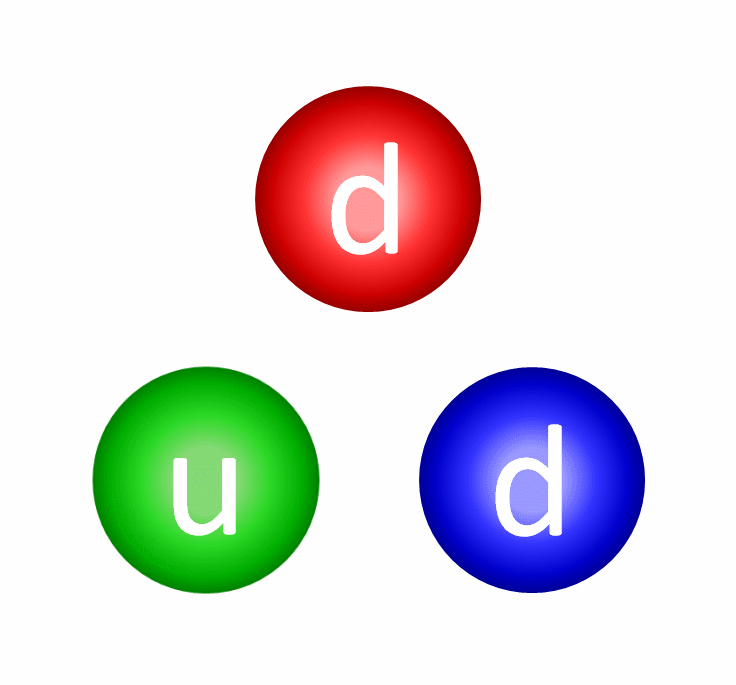
That turns out to be a good explanation for six of the gluons: red/anti-green, red/anti-blue, green/anti-red, green/anti-blue, blue/anti-red, and blue/anti-green.
But what about the others: there should also be red/anti-red, green/anti-green, and blue/anti-blue, right?
Almost, it turns out. Because each of those has no inherent color, those quantum states are allowed to mix together. In quantum physics, whenever mixing isn’t forbidden, it happens, and that happens whenever any theoretical systems have identical quantum numbers, as is the case here. So instead of “pure” red/anti-red, green/anti-green and blue/anti-blue, you get mixtures of red/anti-red, green/anti-green, and blue/anti-blue states.
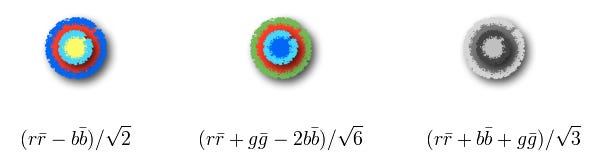
But one of them — the one that’s an equal mixture of all three color/anti-color pairs — is truly colorless, and doesn’t physically exist. So there are only eight physical gluons. (The actual math of this comes from the group theory of SU(3), if you’re interested in learning the rigorous explanation.)
And it’s the exchange of these gluons between the quarks and antiquarks that keep protons, neutrons, mesons, baryons, and all other atomic nuclei together. This is why, if you try to rip any two quarks or antiquarks apart, the energy required increases, eventually reaching a point where you’ll simply pull a particle/antiparticle pair out of the vacuum, creating extra particles in the process.
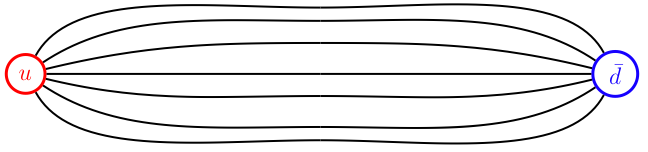
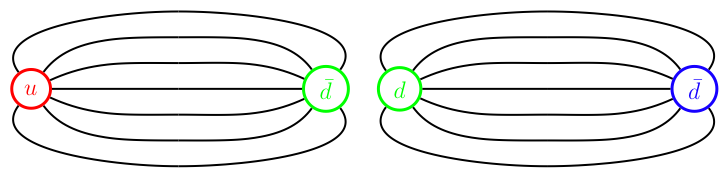
There is much more to the strong interactions than what I’ve described here, and if you want to go deeper, I recommend this jaunt by Nobel Laureate Frank Wilczek. Whether you do or not, the strong force is what holds every atomic nucleus together; without it, we’d simply be a lifeless sea of fundamental particles, too repulsive to hold together in any meaningful fashion, and incapable of building any elements other than hydrogen in the entire Universe.
No stars would ever shine, no complex molecules would ever form, and there would never be a rocky planet anywhere in the Universe: just clumps of gas and great, empty voids.
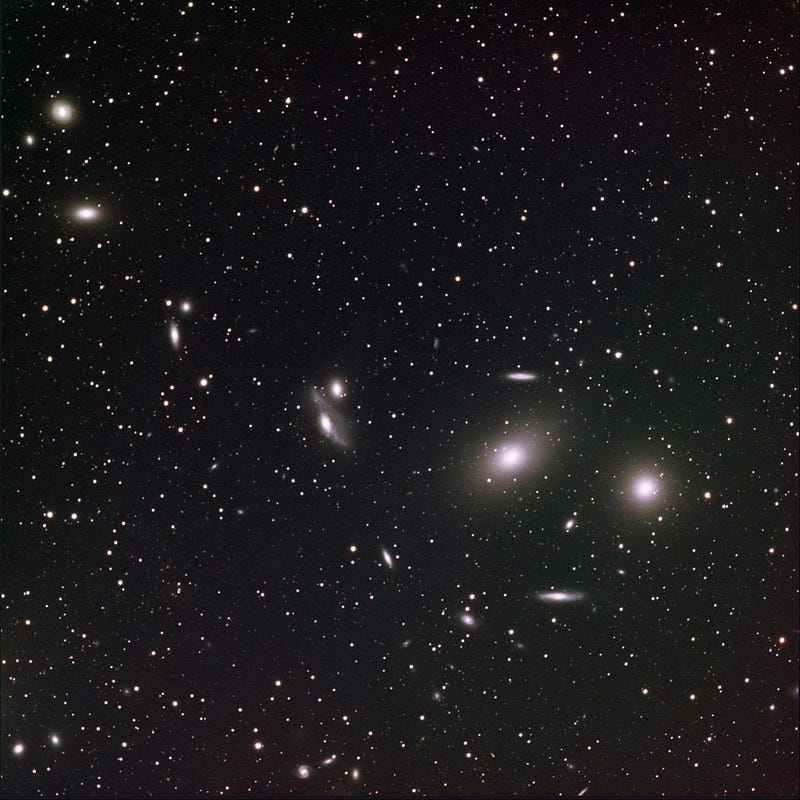
And yet, here we are, so much more than a cosmic soup, with galaxies, stars, planets, heavy elements, molecules, life, and you and me. It’s the strongest force in the Universe, and we owe everything interesting about our existence to it. Without it, none of this would be possible.
Leave your comments at the Starts With A Bang forum on Scienceblogs!