Throwback Thursday: The 95th Anniversary of Relativity’s Confirmation
How the Solar Eclipse of 1919 changed our understanding of the Universe forever.
“Oh leave the Wise our measures to collate. One thing at least is certain, light has weight. One thing is certain and the rest debate. Light rays, when near the Sun, do not go straight.” –Arthur Eddington
Back in the 19th century, Newtonian gravity reigned supreme. Not only did it explain the accelerated motion of all objects here on Earth, but it also accounted for the motion of all the planets. Most spectacularly, it made an incredibly bold prediction when it came to the planet Uranus, which was only discovered in the 1780s.
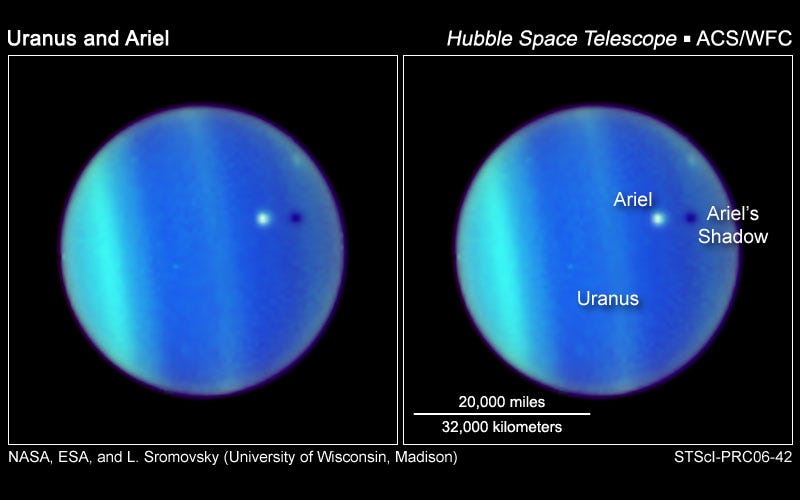
You see, if you applied Newton’s law of gravitation to Uranus, you’d get a very specific prediction for how it ought to have been moving at all points in its orbit. Mercury, Venus, Earth, Mars, Jupiter and Saturn all followed the Newtonian prediction perfectly, but when it came to Uranus — which had been observed for a period of a little over 60 years by the mid-19th century — there was something amiss.
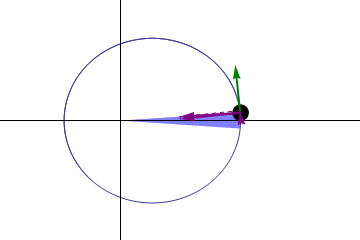
You see, based on Newton’s gravity, Kepler’s three laws could be derived:
- Planets move in ellipses with the Sun at one focus.
- Planets move along that ellipse at such a speed that they sweep out equal areas in equal times.
- The period of a planet’s orbit squared is proportional to its semimajor axis (i.e., for a circular orbit, the radius) cubed.
And while the first and third laws held for Uranus, the second one didn’t! You see, Uranus appeared to move too quickly as compared to its predicted speed at first, then slowed down to the expected speed, but then slowed down even further, to below its predicted speed. And this appeared to fly in the face of Newton’s theories.
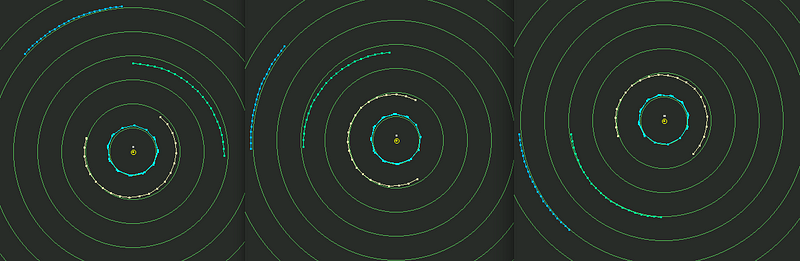
But this could be explained, theorists realized, if there were another massive planet exterior to Uranus that was tugging on it. While the planet led Uranus in its orbit (L), it would cause it to accelerate and move a little too quickly, while they were roughly aligned (middle), Uranus would move at the predicted speed, and when it fell behind (R), Uranus would slow down.
And in 1846, when observers discovered Neptune in the predicted location, it looked like another stupendous victory for Newtonian gravity. So when observations improved and we discovered a slight problem with Mercury’s orbit, you can only imagine what ensued.
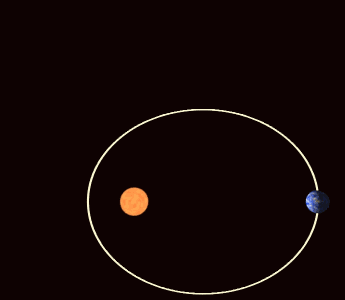
All planetary orbits precess a little bit, meaning that as they make ellipses around the Sun, they don’t return to the same starting point as they complete their orbits. Much of this is predicted by Newtonian physics, but there was a little bit of Mercury’s orbit — an extra 43″ per century out of a total of 5599″ — that Newtonian physics couldn’t account for.
Why was Mercury’s orbit precessing at the rate observed? Three alternate hypotheses came up:
- there was an inner planet to Mercury, which was causing the perihelion advance,
- Newton’s law of gravity needed to be slightly modified; perhaps instead of a 1/r^2 law, it was actually 1/r^(2 + ϵ), or
- Newtonian gravity needed to be replaced with a more complete theory of gravitation.
Of course, the smart money was on the first option. It was so strongly assumed that it was even named: Vulcan.
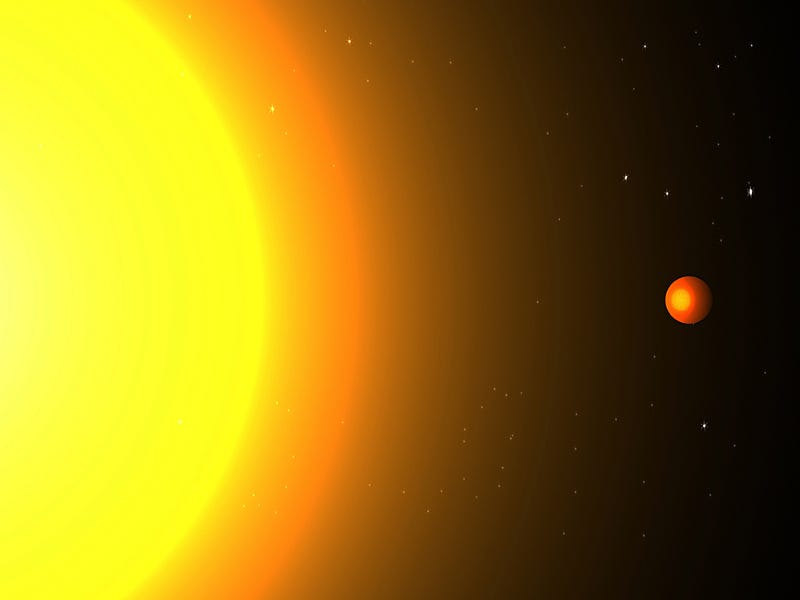
But after exhaustive searches for a new mass near the Sun, no planet was found. This minuscule difference between Mercury’s predicted orbit and the ever-improving observations was significant enough that it led some to consider that Newton’s Law of Universal Gravitation might be wrong.
Newton said that mass and separation distance was what determined gravity. There was a force that he called “action at a distance” that made everything attract. But during the time from 1909-1916, a new theory came about.

The same guy who discovered the photoelectric effect, special relativity, and E=mc^2 came up with a new theory of gravity. Instead of an “action at a distance” due to mass, this new theory said that space gets bent by the presence of matter and energy, and causes everything — even massless things — to bend and deform beneath what we see as gravity.
Now this new theory was very interesting for a few reasons. First off, it accounted for those extra 43″ (just 0.011 degrees) per century that Newton’s gravity did not. Second, it predicted — as a simple solution — the existence of black holes. And third, it predicted that something very exciting and testable would happen: that light would be bent by gravity.
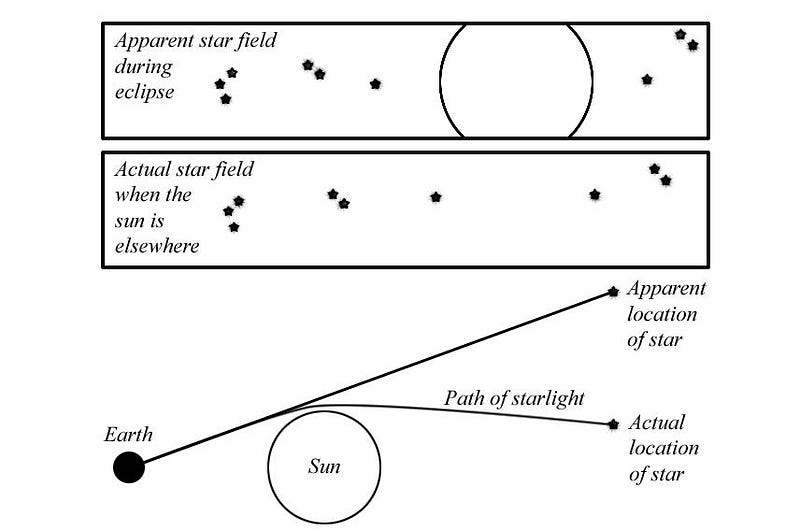
Big deal, said Newton’s advocates. If I take E=mc^2, and I know that light has energy, I can just substitute E/c^2 for “mass” in Newton’s equations, and get a prediction that Newton’s gravity would bend light, too. But were Newton’s and Einstein’s predictions identical?
It just so happened that Einstein’s bending — near the limb of the Sun, our most massive nearby gravitational source — was predicted to be twice as much as Newton’s bending. Lucky for us, a total Solar Eclipse isn’t an entirely rare event, and during the moment of totality, we run into the very rare phenomenon of stars being visible during the day.
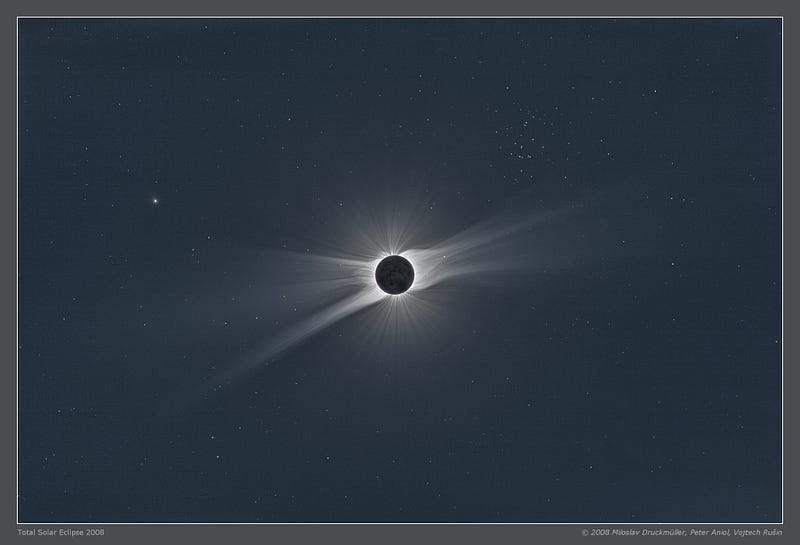
These measurements were first attempted during the solar eclipse of June 8, 1918, but clouds prevented the U.S. Naval Observatory from making the key measurements. So when the next one came around — the May 29, 1919 solar eclipse — everyone was prepared.
The director of Cambridge Observatory, Sir Arthur Eddington, led an expedition to Africa to observe the total solar eclipse of May 29, 1919, and coordinated another one to Sobral, Brazil to make similar observations. Eddington set out to map the position of the stars when they were close to the Sun, and see how the Sun bent the light. Would it match up with Einstein’s prediction, Newton’s prediction, or would it not bend the starlight at all?
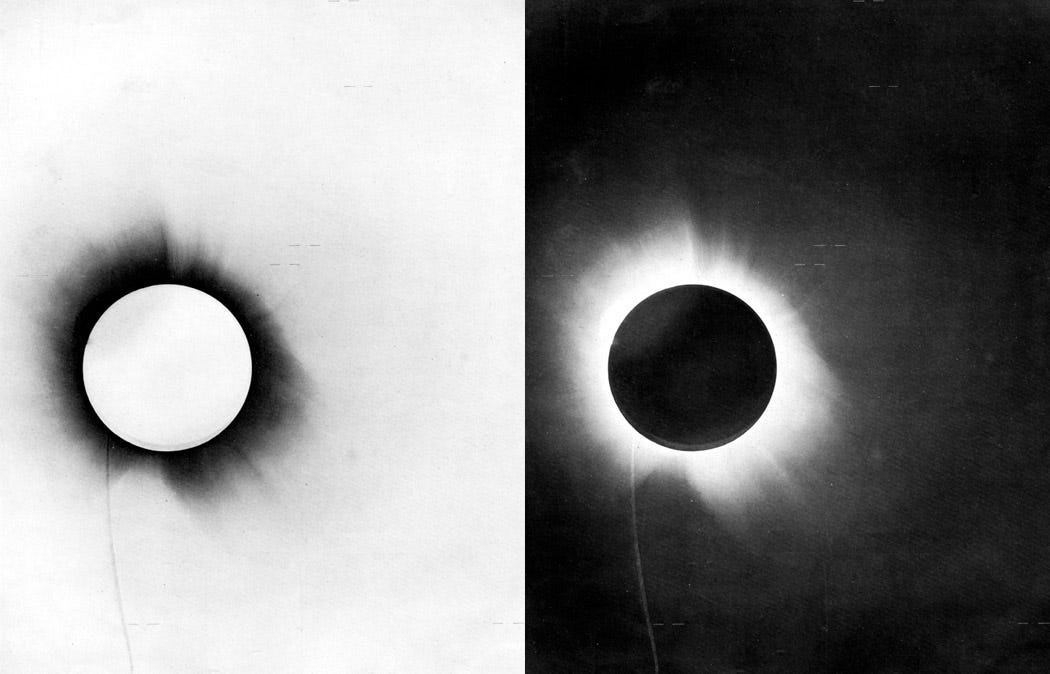
When the observations came in, it turned out that Einstein’s predictions were vindicated, and both no light bending and the Newtonian prediction for light bending were ruled out. Subsequent eclipses and other tests have further discerned the differences between Newtonian and Einsteinian gravity, and general relativity emerges victorious in every scenario. In fact, an archival photo from the 1900 eclipse has since been unearthed, and it agreed with Einstein’s prediction, too. In theory, we could have verified relativity even earlier!
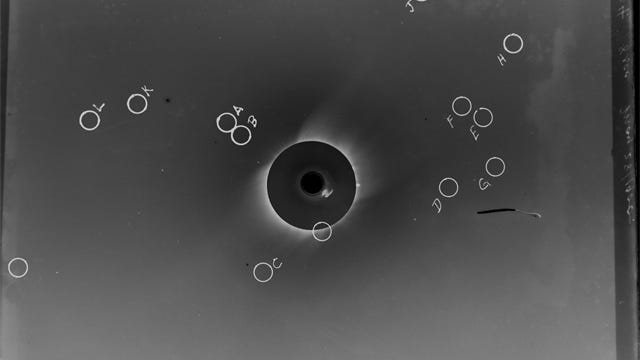
But on this day in 1919, our understanding of the Universe changed forever. Six months later, when the analysis was complete, the international press had a field day.
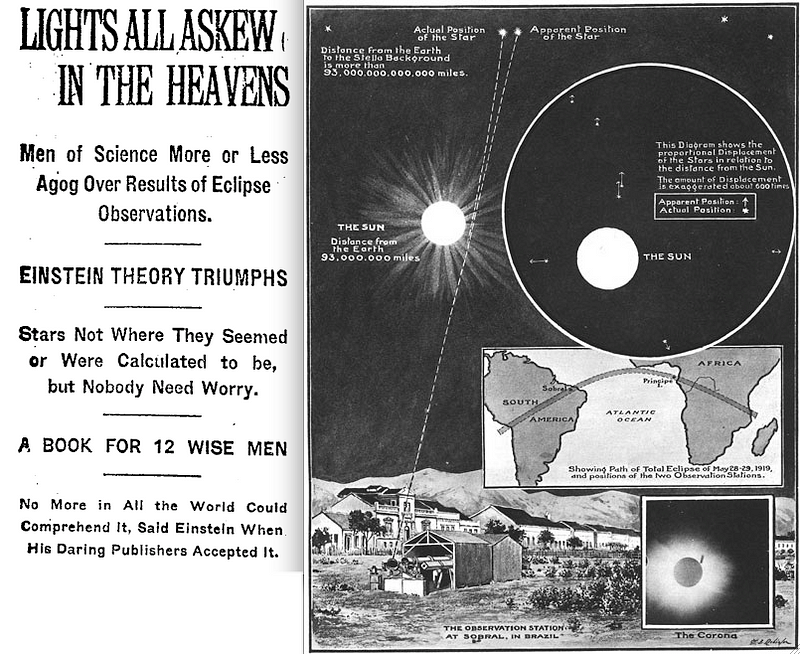
And on the 95th anniversary of this historical event, we can look back and find that every single prediction of Einstein’s gravity that’s ever been tested — from gravitational lensing to binary pulsar decay to time dilation in a gravitational field — has confirmed General Relativity as perhaps the most successful physical theory of all-time.
Enjoyed this? Leave a comment at the Starts With A Bang forum on Scienceblogs!