This Multi-Trillion Dollar Disaster Is Coming, And Solar Astronomy Is Our Prime Defense
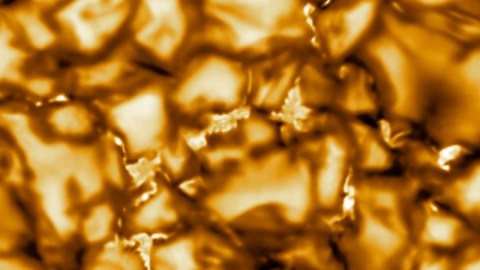
The NSF’s new, cutting-edge solar observatory shows us the Sun as never before. Here’s why we need to know.
On December 12, 2019, the world’s most powerful solar observatory — the National Science Foundation’s Daniel K. Inouye Solar Telescope — opened its eyes for the first time. With a whopping 4-meter diameter primary mirror and a unique, off-center design, the Inouye Solar Telescope is capable of imaging features as small as 30 km in size on the Sun. Already, in its first light images released on January 29, 2020, features in-between the Texas-sized convective cells were revealed for the first time ever.
But the Inouye Solar Telescope offers so much more than just gorgeous images of our parent star; it’s one of a number of solar astronomy projects that are all working together to protect our planet from a multi-trillion dollar disaster that’s definitely coming: a catastrophic solar flare. It could come anytime this year or not for another few centuries, but studying the Sun is the only way to be prepared. Here’s the science behind these beautiful images and videos.
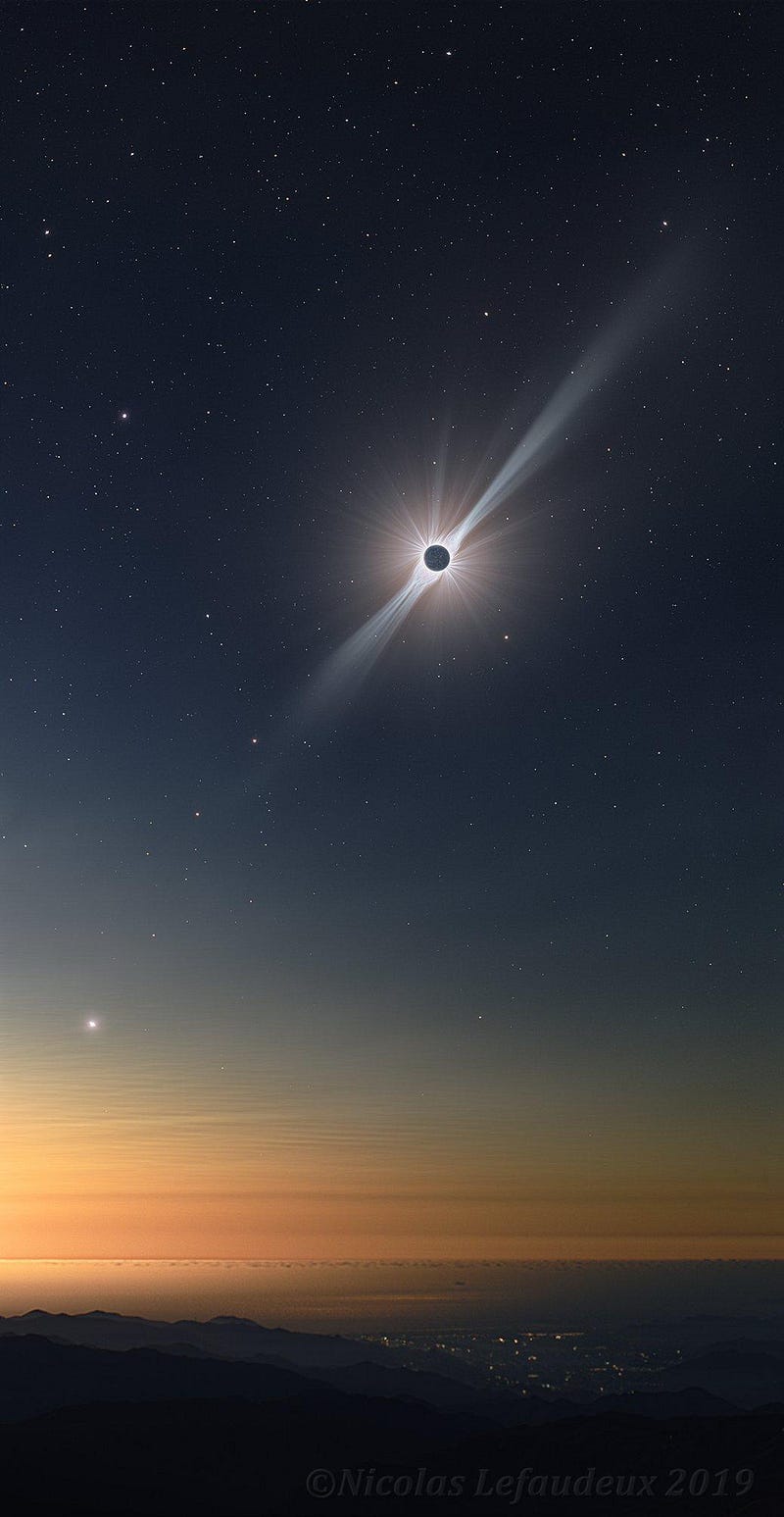
Until 1859, solar astronomy was extremely simple: scientists studied the light from the Sun, the sunspots that occasionally dotted the Sun’s surface, and viewed the corona during solar eclipses. But in 1859, solar astronomer Richard Carrington happened to be looking at the Sun, tracking a large, irregular sunspot, when something unprecedented occurred: a “white light flare” was observed, intensely bright and moving across the spot itself for around 5 minutes before disappearing entirely.
This turned out to be the first-ever observation of what we now call a solar flare. Some 18 hours later (about three to four times the speed of most solar flares), the largest geomagnetic storm in recorded history occurred on Earth. Aurorae were observed around the world: miners awoke in the Rockies; newspapers could be read by the aurora’s light; the bright green curtain appeared in Cuba, Hawaii, Mexico and Colombia. Telegraph systems, even when disconnected, experienced their own induced currents, causing shocks and even starting fires.
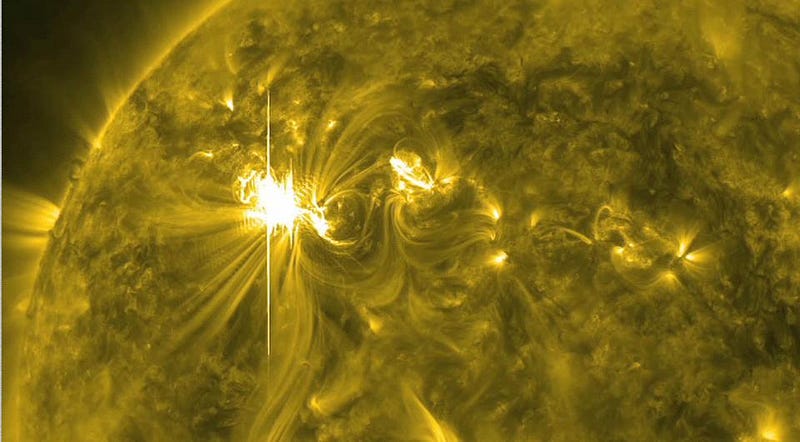
If such an event were to occur today, the infrastructure we have for electricity and electronics would experience devastating effects that could easily cause trilions of dollars in damage. The problem is that geomagnetic storms, formed when certain space weather events penetrate our magnetosphere and interact with the atmosphere, can cause massive currents to flow even in electronic circuits that are completely disconnected.
A key science goal for solar astronomy is to understand how the interplay between the Sun, the space weather that causes these storms, and the effects on Earth itself are all related. This is why the NSF’s Inouye Solar Telescope has, as its prime science goal, to measure the magnetic field of the Sun at three different layers:
- at the photosphere,
- in the chromosphere,
- and throughout the solar corona.
With its enormous 4-meter diameter and its five science instruments — four of which are spectro-polarimeters designed for measuring the Sun’s magnetic properties — it will measure the magnetic fields on and around the Sun as never before.
Measuring the magnetic field at various layers on the Sun is the most important thing we can do for predicting space weather, which comes as a surprise to most people. In the late 1980s, everyone was talking about solar flares as the drivers of space weather, and that’s what most discussions still focus on. However, that only tells a tiny part of the story, since sometimes solar flares can cause spectacular geomagnetic storms on Earth, but at other times, they have no effect.
Our first major step towards understanding the role of magnetic fields came in 1995, when NASA’s SOHO observatory was launched. What it saw was not just solar flares occurring at the photosphere, but a new type of phenomenon: coronal mass ejections (CMEs), which originate farther away from the Sun than the photosphere. If you’ve ever seen a blue animation of the Sun where the solar disk is blocked by a coronagraph, you’ve seen an image from SOHO.
When CMEs come to Earth, that’s what causes a space weather event. A solar flare without a CME won’t be capable of causing a large geomagnetic storm; one of the things that SOHO taught us is that that the Earth’s magnetic field will protect us from normal solar flares extremely well, leading to a minor auroral event at most.
But many solar flares will lead to coronal mass ejections, particularly if there’s a solar prominence nearby. Prominences are high-density collections of material that reside in the corona, and CMEs typically occur where the prominences found on the Sun magnetically break, which leads to the ejection of material. The CMEs themselves are directionally oriented, and it’s only the ones that wind up striking Earth that put us at risk. When a CME goes off to the side, there’s no worry; but when we see an annular CME from our perspective, that’s when they’re headed right for us.
But even solar flares that cause CMEs that are directed right at Earth don’t necessarily cause geomagnetic storms; there needs to be one other piece of the puzzle that lines up just right: there needs to be the right magnetic connection. Remember that magnets typically have North and South poles, where like poles (North-North or South-South) repel, but opposite poles (North-South or South-North) attract.
Earth has its own magnetic field, which — from a distance — looks kind of like a bar magnet aligned close to our axis of rotation. If the magnetic field of the material ejected during a CME is aligned with Earth’s field, the solar particles will be repelled, and no geomagnetic event will occur on Earth. But if the fields are anti-aligned, like they almost certainly were 161 years ago for the infamous Carrington event, you’ll get a spectacular (and possibly dangerous) event, with the greatest auroral displays and much, much more.
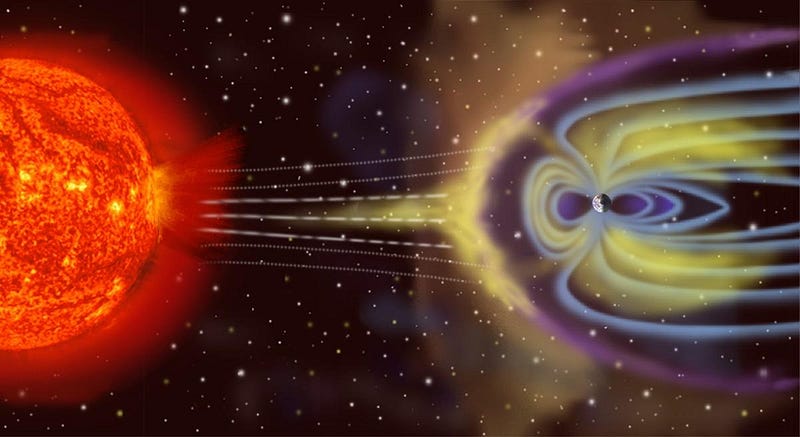
Since the 2000s, our best tools for measuring the magnetic fields of the charged particles from CMEs that head towards Earth are the slew of satellites and observatories placed at the L1 Lagrange point: a point in space located about 1,500,000 km away from Earth on the Sun-facing side. Unfortunately, that’s already 99% of the way from the Sun to the Earth; we typically only get about ~45 minutes from when a CME arrives at L1 until it arrives on Earth, and either produces a geomagnetic storm or not.
Ideally, what our next generation of solar observatories would bring us is a great increase in the amount of time we’ll have to know whether we need to take the appropriate mitigating actions when such a potentially catastrophic coronal mass ejection occurs. There are plenty of things we can do, but we need more than an hour of advanced notice in order to do them.
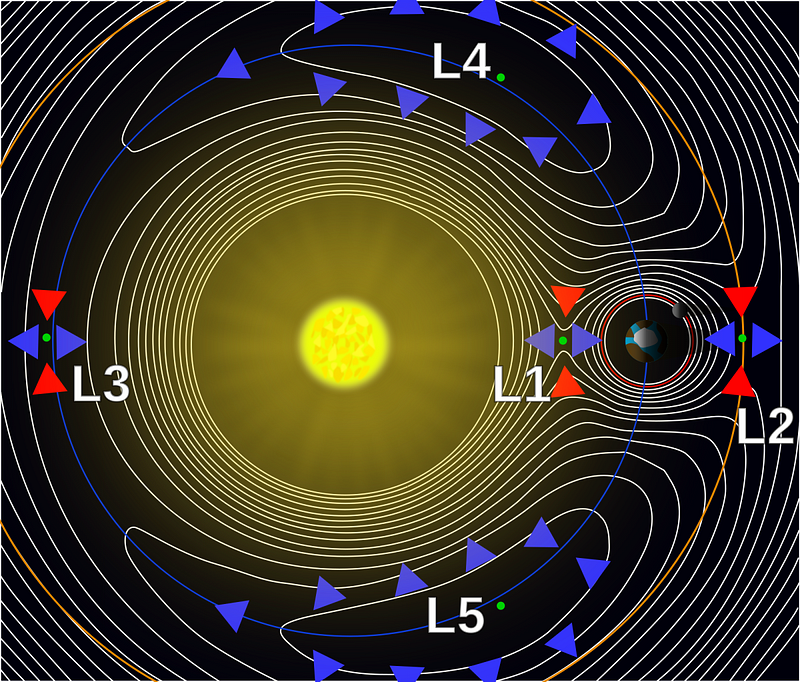
The way we can best mitigate the damage from space weather events on Earth is to have power companies cut off the currents in their electrical grids, and disconnect (and sufficiently ground) stations and substations instead, so that the induced current doesn’t flow into homes, businesses and industrial buildings. Because of the enormous magnitude of the currents, they need to be safely and gradually ramped down, which typically takes around a day, rather than an hour, to enact.
The key to knowing whether a CME has the appropriate component of its magnetic field aligned or anti-aligned well in advance of its arrival on Earth is to measure the magnetic field on the Sun; instead of ~45 minutes of lead time, you can get the full ~3 days or so that it typically takes ejected coronal material to travel from the Sun to the Earth.
The Inouye Solar Telescope is precisely this amazing solar-measuring magnetometer that we need to make these observations.
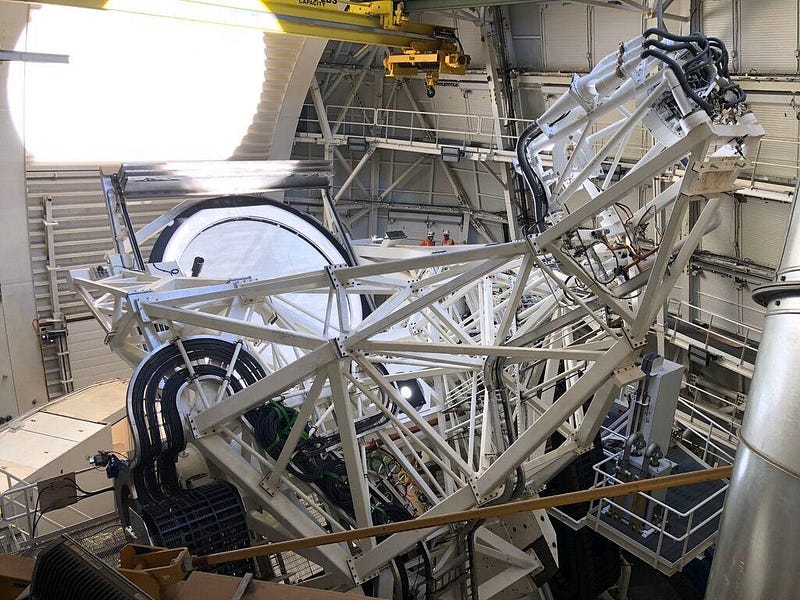
Practically every problem we’re trying to solve about the Sun is a magnetic problem. If we want to understand what’s occurring at the Sun’s photosphere, it’s driven by the heating from the inner layers of the Sun, but is distributed according to the magnetic field and its distribution throughout the Sun’s outer layers. Magnetic connectivity extends from the photosphere to the chromosphere to the corona, which provides heating, winds, and enables the corona to be so energetic.
The winds generated in the hot corona creates the magnetic connection between the Earth and the Sun, and in fact between the Sun and the remainder of the Solar System, relevant for aurorae on planets even in the outer Solar System. No matter how well we measure the other properties of material from the Sun — velocity, kinematics, energy, calorimetry, etc. — the magnetic properties are key to understanding what drives the Sun’s processes.
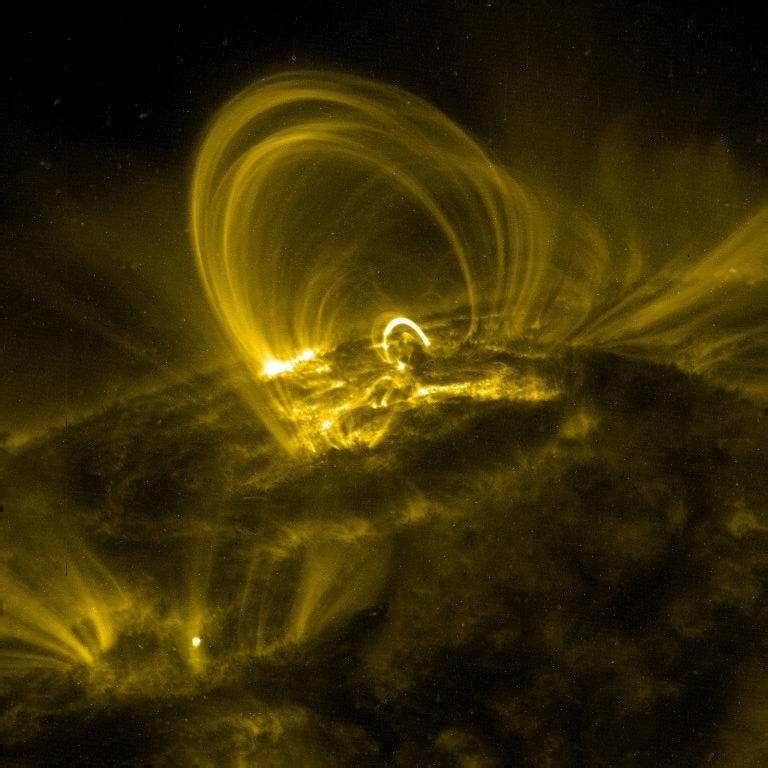
To understand what’s going to impact Earth and how, we need a comprehensive understanding of what’s occurring not only on the Sun itself, but from the particles ejected from it at every level:
- from the photosphere,
- through the chromosphere,
- to the corona,
- through interplanetary space,
- through the L1 Lagrange point,
- and onto our planet itself.
A combination of the Inouye Solar Telescope, the Parker Solar Probe, the upcoming Solar Orbiter mission, along with L1 satellites such as SOHO and SDO, will enable us to understand the magnetic connection between the Sun and the Earth as never before. The NSF’s Inouye Solar Telescope, which measured not only the Texas-sized convective cells on the Sun to better precision than ever but also features lining the space between those cells for the first time, is an indispensible part of that.
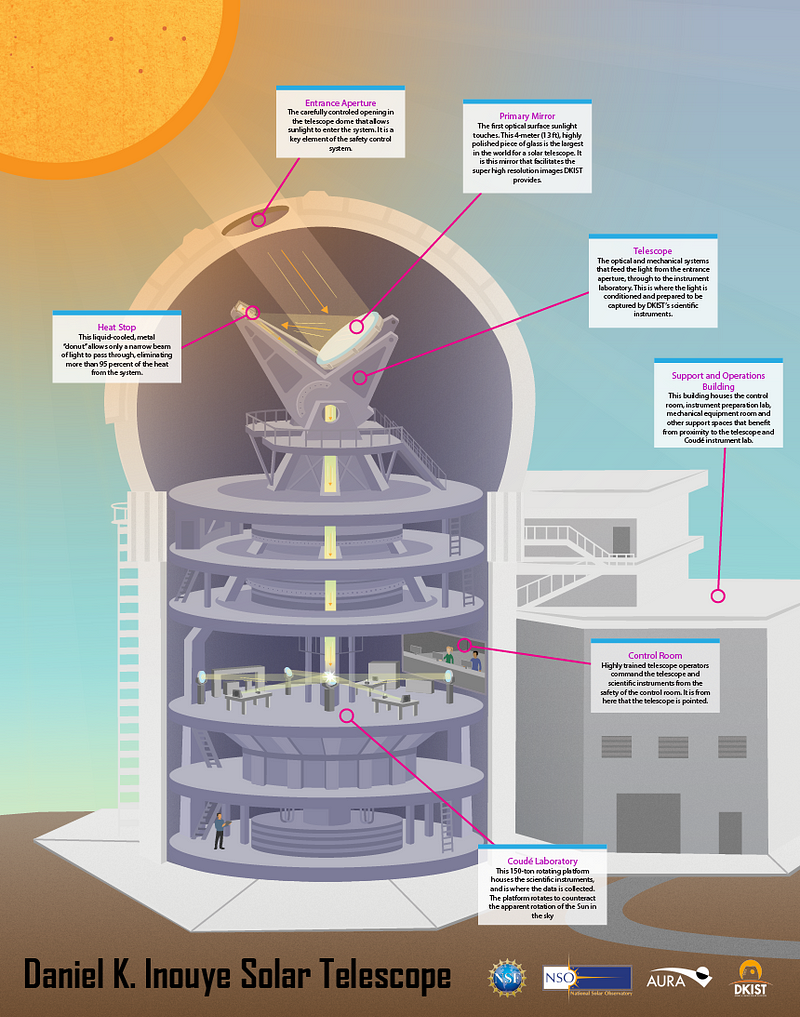
Although the largest solar flares are rare, they do occur with some regularity. Some of them create coronal mass ejections; some coronal mass ejections head directly towards Earth; some of the ones that do head towards Earth have exactly the right properties to create spectacular aurorae and potentially catastrophic geomagnetic storms. Only now, with this new generation of solar astronomy tools, are we finally in position to scientifically prepare for the inevitable disaster.
For decades, we’ve avoided the ruination of our modern infrastructure through sheer luck alone. A Carrington-level event, if it were to strike us unawares, would certainly cause trillions of dollars worth of damage worldwide. With the advent of these new heliophysics-focused observatories, led by the NSF’s Daniel K. Inouye Solar Telescope, we’ll finally have the opportunity to know when “the big one” is coming.
Ethan Siegel thanks Claire Raftery, Thomas Rimmele, and (especially) Valentin Pillet for useful discussions and interviews about solar astronomy and DKIST.
Ethan Siegel is the author of Beyond the Galaxy and Treknology. You can pre-order his third book, currently in development: the Encyclopaedia Cosmologica.