This Is How Hubble Will Use Its Remaining Gyroscopes To Maneuver In Space
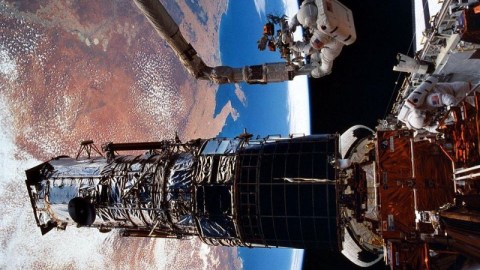
Every mechanical failure brings Hubble one step closer to its demise. But despite the recent setback, it’s still got plenty of life left.
If you want to view the distant Universe with the highest sensitivities and the smallest amounts of contamination possible, your best bet is to go to space. The Hubble Space Telescope, launched in April of 1990, is perhaps the most famous astronomical observatory in all of human history. Orbiting the Earth at an altitude of 550 kilometers (340 miles), at a speed of around 27,000 kph (17,000 mph), it completes a revolution around our planet every 95 minutes.
Simultaneously, the Earth spins on its axis and revolves around the Sun, which in turn moves through the galaxy at nearly 0.1% the speed of light. Yet, somehow, Hubble always manages to point at its astronomical targets stably and without difficulty, despite all of these motions. The key is in its guidance systems and, in particular, in its gyroscopes. Here’s how, despite a recent failure, Hubble is poised to keep revealing the Universe’s secrets for years to come.
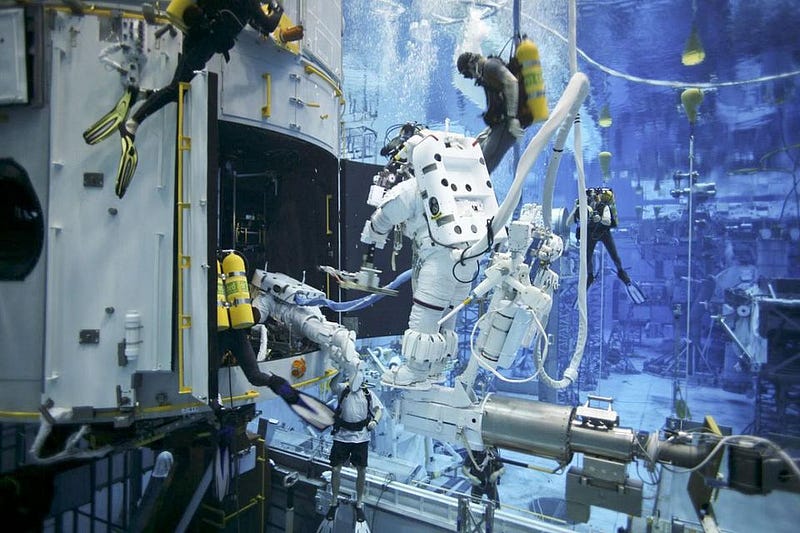
Pointing at a single object without wavering or faltering is no small task. From its location in space, Hubble doesn’t have to contend with the atmosphere, meaning that its resolution and imaging capabilities are limited only by the optics and instruments on board. Last upgraded in 2009, with the final Hubble servicing mission carried out from the Space Shuttle, Hubble is capable of delivering images with precision of just a few millionths of a degree.
But one of the key challenges is keeping your entire telescope steady and accurate in how it’s pointed. To that end, the Hubble Space Telescope was designed to lock onto a target and hold its position steady to precisions of just 0.007 arcseconds. To understand how impressive that is, that’s the equivalent of shining a laser beam onto a quarter and hitting George Washington’s eye, without fail, from a distance of 14 kilometers (8.7 miles) away.
Here on Earth, we take for granted how easy it is to orient pretty much anything. We can point whatever we want in whatever direction we want simply by manipulating it, either by hand or by machine.
But the only reason we’re able to do this is because there’s something to brace ourselves against: the Earth. When you exert a force on any object, that object pushes back against you with an equal and opposite force. That’s because of the law, first discovered by Newton, stating that every action has an equal and opposite reaction.
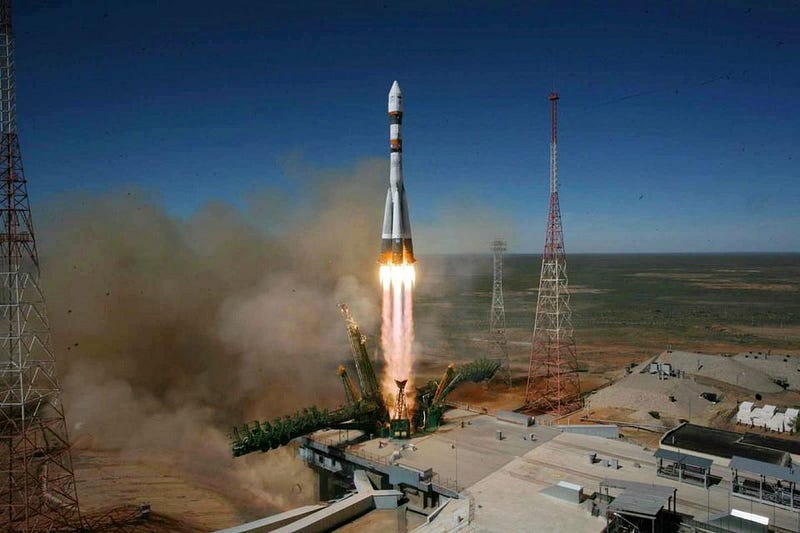
But in space, there’s nothing else to push against. However you’re moving, and that includes both your straight-line motion and your rotational motion, that’s how you’re going to continue to move. The only external forces come from gravitation and a very slight drag force from the atoms and particles that exist in interplanetary space.
If you were stuck facing towards the Sun and wanted to turn yourself to face away, you couldn’t. If you’re not spinning, you cannot start yourself spinning because there’s nothing to push against. And similarly, if you are spinning, you couldn’t slow yourself down, because there’s also nothing to push against. Whether you’re an object at rest or an object in motion, the only way that’s going to change is if there’s an external force.
This would work if you had a second object in space along with you for you to push against. Astronauts aboard the International Space Station can push against the hull of the station or another astronaut, and change their momentum or angular momentum. The cost? Whatever you push against has to change its momentum or angular momentum by an equal-and-opposite amount.
So then, what do you do if you’re a space telescope, up there on your own, without anything else to push against?
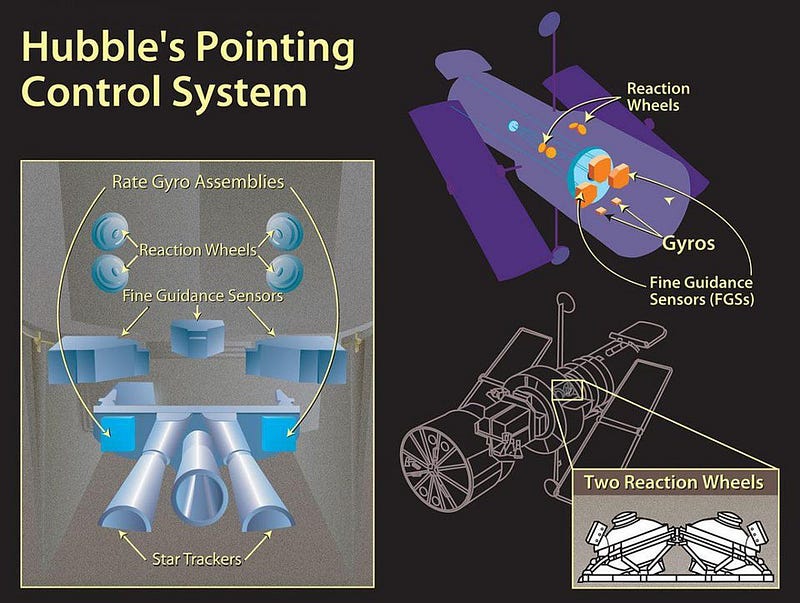
You need a component inside of you to be the thing you push off of to change your motion. If you were alone in space, for example, by swiveling your lower body clockwise, you could then cause your upper body to swivel counterclockwise; you could push off a different part of your body to change your orientation.
In a space telescope, we don’t have different components of our bodies to work with, but we do have different components of the telescope. And in the case of Hubble, we have an entire guidance system built on this principle.
The reaction wheels allow it to change its orientation, and the fine-guidance sensor allows it to determine how to orient itself. According to NASA itself:
To change angles, it uses Newton’s third law by spinning its wheels in the opposite direction. It turns at about the speed of a minute hand on a clock, taking 15 minutes to turn 90 degrees.
But keeping the telescope stable needs a key ingredient: gyroscopes.
Without those gyroscopes, tiny external forces would cause Hubble’s orientation to drift over time, and would make long-exposure images impossible. But with them, we can keep the telescope stable.
In 2009, during the final servicing mission, all six of Hubble’s gyroscopes were replaced, in the hopes of extending its life as long as possible. The gyroscopes maintain orientation and provide stability by pushing back against any force that attempts to change its orientation. For Hubble, each gyroscope contains a wheel that spins at 19,200 rpms, and three are required for optimal operating efficiency. The reason we need three is simple: there are three dimensions in space, and so three independent ways that a spacecraft could potentially change its orientation. With three gyroscopes operating at once, we can achieve maximal stability.
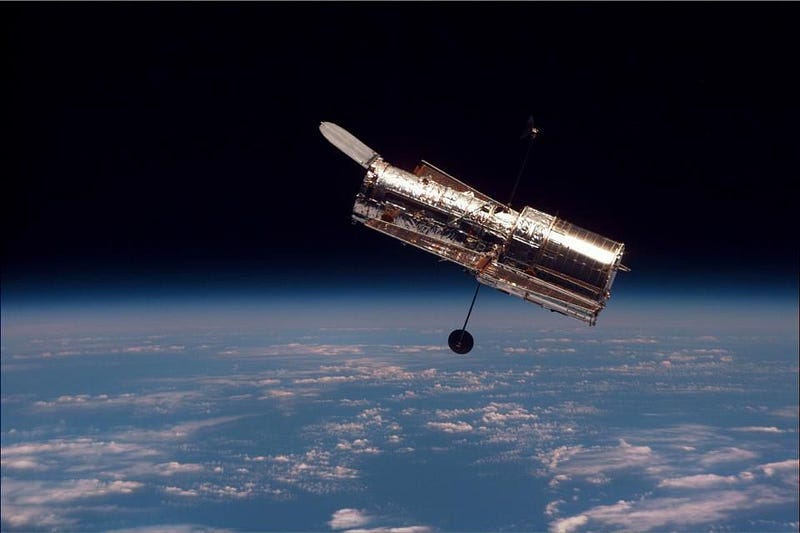
On October 5th, 2018, the Hubble Space Telescope entered safe mode, owing to the fact that one of the three gyroscopes actively being used to point-and-steady the telescope had failed. Engineers have fixed problems like this before, from the ground, by firing up another of the on-board gyroscopes and switching which three are used to stabilize the observatory. The gyroscope that failed wasn’t entirely surprising; it had been showing signs of trouble for about a year.
But there are already two other gyroscopes which had failed of the replaced six, and another which has shown signs of being problematic already. With two good gyroscopes and one partially-malfunctioning one, it’s a solemn reminder that Hubble won’t live forever, particularly with no ability for humanity to service it again.
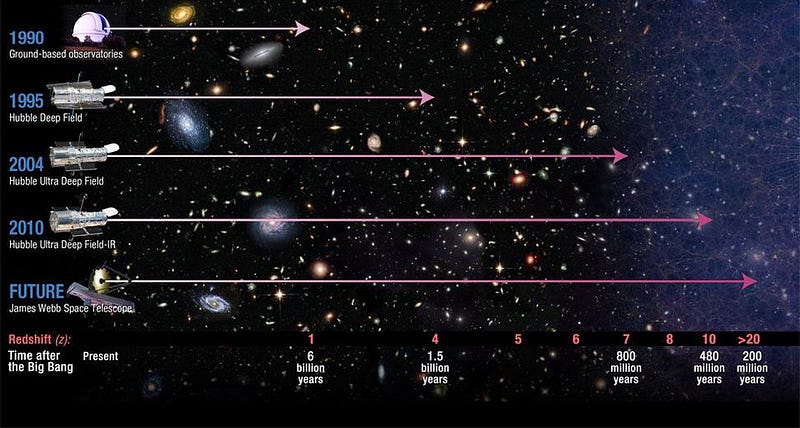
With two fully functioning gyroscopes, the team operating Hubble will switch to the final plan: operating in one-gyroscope mode. With three gyroscopes, you can point pretty much anywhere you want and keep your observatory stable; with fewer than that, your perspective on the sky suddenly becomes restricted.
That’s why the plan is to attempt to fix the partially-malfunctioning gyroscope remotely. If you succeed, you have three functioning gyroscopes and Hubble can continue to operate as normal. If they cannot cure the partially-malfunctioning gyroscope, they will power-down one of the functional gyroscopes and save it. You can observe nearly as much of the sky with one gyroscope as you can with two, but you basically double your telescope’s remaining life by using one gyroscope at a time instead of two together. At a cost of reduced sky coverage and slower pointing times, you can extend Hubble’s life.
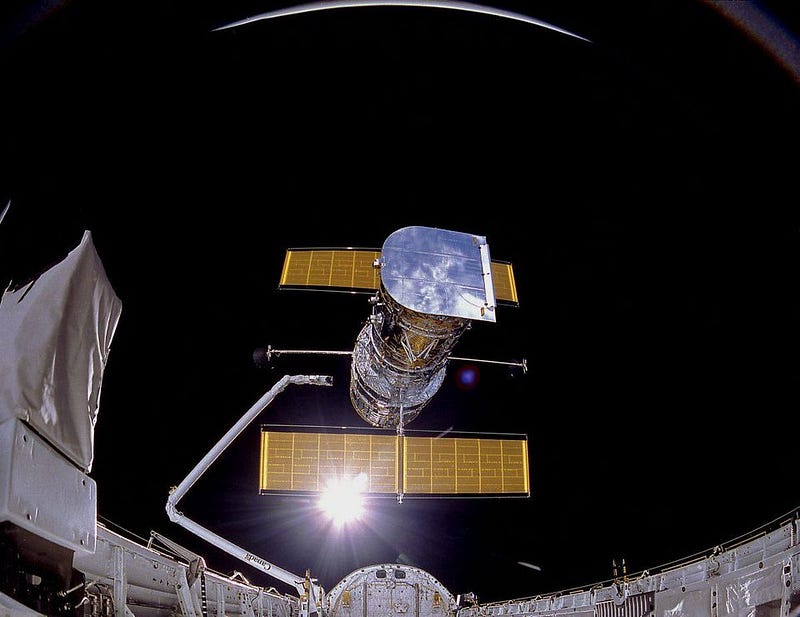
It might seem to be just another example of crumbling infrastructure in the United States, but you must neither underestimate Hubble nor the resourcefulness of astronomers and scientists and engineers overall. The two (or maybe three) remaining gyroscopes are of a new and upgraded design, designed to last five times as long as the original gyroscopes, which includes the one that recently failed. The James Webb Space Telescope, despite being billed as Hubble’s successor, is actually quite different, and will launch in 2021.
Even with one gyroscope, the Hubble Space Telescope should still be operational and capable of providing complementary observations to James Webb. This reduced-gyro mode has been planned for a long time. The only disappointment is that we may need to enter it so soon.
Ethan Siegel is the author of Beyond the Galaxy and Treknology. You can pre-order his third book, currently in development: the Encyclopaedia Cosmologica.