There Was No Big Bang Singularity

It’s one of the biggest assumptions we’ve ever made about the Universe. Here’s why it’s wrong.
Almost everyone has heard the story of the Big Bang. But if you ask anyone, from a layperson to a cosmologist, to finish the following sentence, “In the beginning, there was…” you’ll get a slew of different answers. One of the most common ones is “a singularity,” which refers to an instant where all the matter and energy in the Universe was concentrated into a single point. The temperatures, densities, and energies of the Universe would be arbitrarily, infinitely large, and could even coincide with the birth of time and space itself.
But this picture isn’t just wrong, it’s nearly 40 years out of date! We are absolutely certain there was no singularity associated with the hot Big Bang, and there may not have even been a birth to space and time at all. Here’s what we know and how we know it.
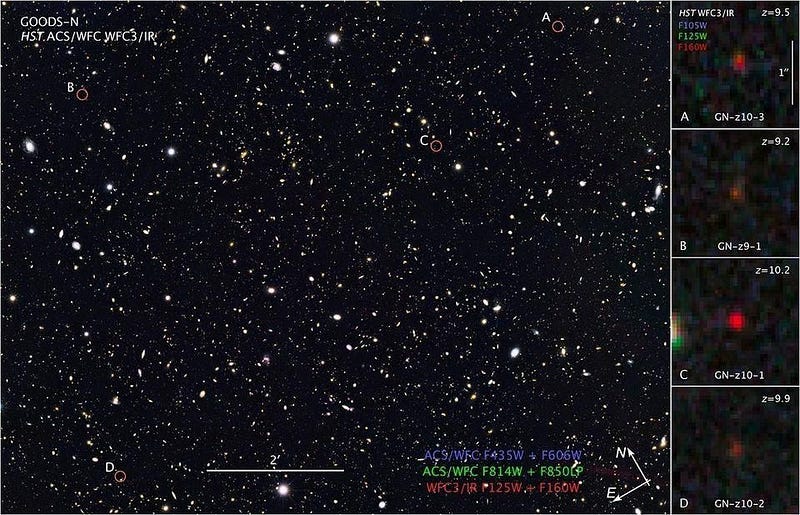
When we look out at the Universe today, we see that it’s full of galaxies in all directions at a wide variety of distances. On average, we also find that the more distant a galaxy is, the faster it appears to be receding from us. This isn’t due to the actual motions of the individual galaxies through space, though; it’s due to the fact that the fabric of space itself is expanding.
This was a prediction that was first teased out of General Relativity in 1922 by Alexander Friedmann, and was observationally confirmed by the work of Edwin Hubble and others in the 1920s. It means that, as time goes on, the matter within it spreads out and becomes less dense, since the volume of the Universe increases. It also means that, if we look to the past, the Universe was denser, hotter, and more uniform.
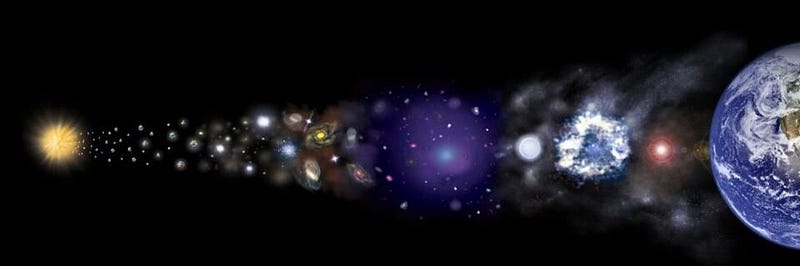
If you were to extrapolate back farther and farther in time, you’d begin to notice a few major changes to the Universe. In particular:
- you’d come to an era where gravitation hasn’t had enough time to pull matter into large enough clumps to have stars and galaxies,
- you’d come to a place where the Universe was so hot you couldn’t form neutral atoms,
- and then where even atomic nuclei were blasted apart,
- where matter-antimatter pairs would spontaneously form,
- and where individual protons and neutrons would be dissociated into quarks and gluons.
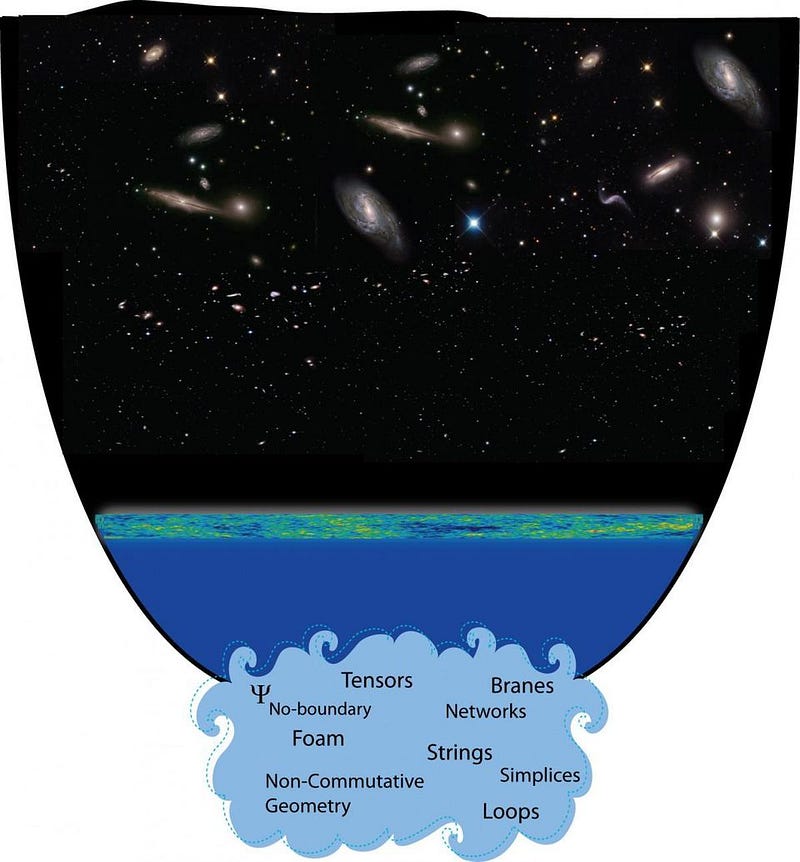
Each step represents the Universe when it was younger, smaller, denser, and hotter. Eventually, if you kept on extrapolating, you’d see those densities and temperatures rise to infinite values, as all the matter and energy in the Universe was contained within a single point: a singularity. The hot Big Bang, as it was first conceived, wasn’t just a hot, dense, expanding state, but represented an instant where the laws of physics break down. It was the birth of space and time: a way to get the entire Universe to spontaneously pop into existence. It was the ultimate act of creation: the singularity associated with the Big Bang.
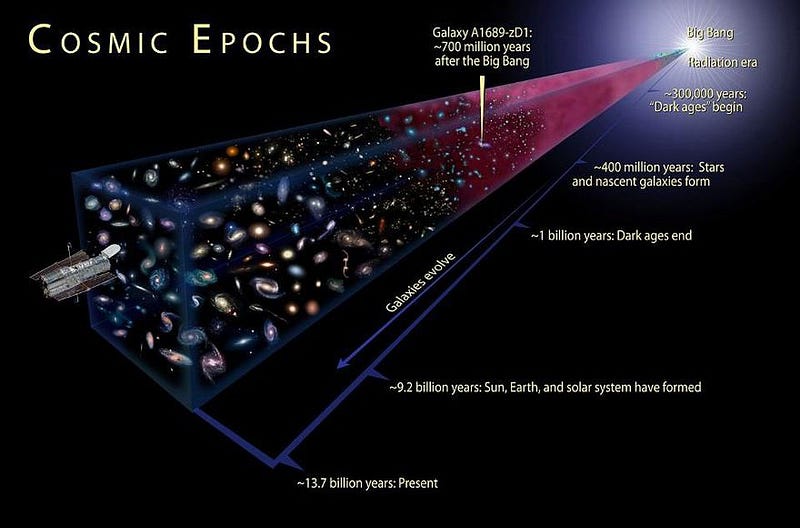
Yet, if this were correct, and the Universe had achieved arbitrarily high temperatures in the past, there would be a number of clear signatures of this we could observe today. There would be temperature fluctuations in the Big Bang’s leftover glow that would have tremendously large amplitudes. The fluctuations that we see would be limited by the speed of light; they would only appear on scales of the cosmic horizon and smaller. There would be leftover, high-energy cosmic relics from earlier times, like magnetic monopoles.
And yet, the temperature fluctuations are only 1-part-in-30,000, thousands of times smaller than a singular Big Bang predicts. Super-horizon fluctuations are real, robustly confirmed by both WMAP and Planck. And the constraints on magnetic monopoles and other ultra-high-energy relics are incredibly tight. These missing signatures have a huge implication: the Universe never reached these arbitrarily large temperatures.
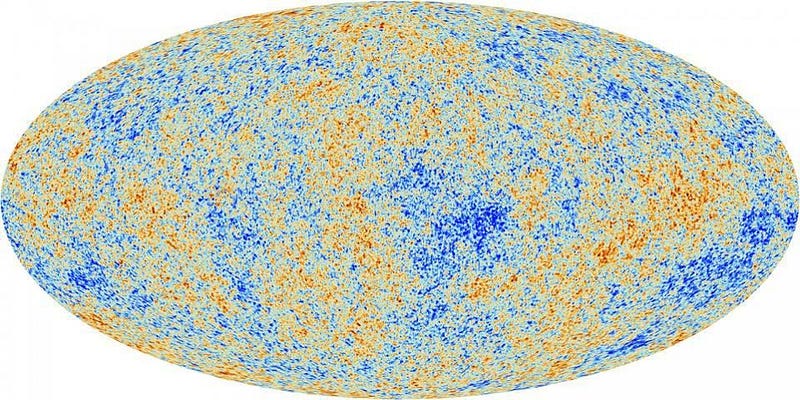
Instead, there must have been a cutoff. We cannot extrapolate back arbitrarily far, to a hot-and-dense state that reaches whatever energies we can dream of. There’s a limit to how far we can go and still validly describe our Universe. In the early 1980s, it was theorized that, before our Universe was hot, dense, expanding, cooling, and full of matter and radiation, it was inflating. A phase of cosmic inflation would mean the Universe was:
- filled with energy inherent to space itself,
- which causes a rapid, exponential expansion,
- that stretches the Universe flat,
- gives it the same properties everywhere,
- with small-amplitude quantum fluctuations,
- that get stretched to all scales (even super-horizon ones),
and then inflation comes to an end.
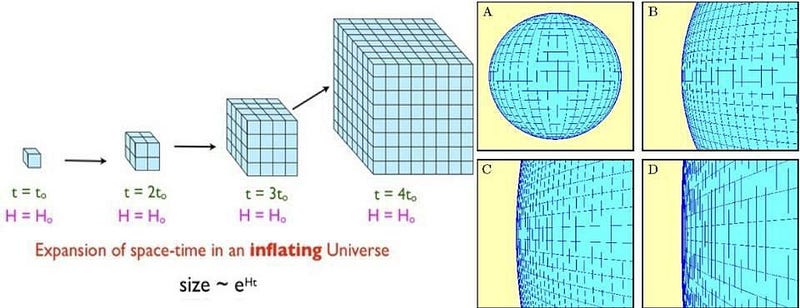
When it does, it converts that energy, which was previously inherent to space itself, into matter and radiation, which leads to the hot Big Bang. But it doesn’t lead to an arbitrarily hot Big Bang, but rather one that achieved a maximum temperature that’s at most hundreds of times smaller than the scale at which a singularity could emerge. In other words, it leads to a hot Big Bang that arises from an inflationary state, not a singularity.
The information that exists in our observable Universe, that we can access and measure, only corresponds to the final ~10^-33 seconds of inflation, and everything that came after. If you want to ask the question of how long inflation lasted, we simply have no idea. It lasted at least a little bit longer than 10^-33 seconds, but whether it lasted a little longer, a lot longer, or for an infinite amount of time is not only unknown, but unknowable.
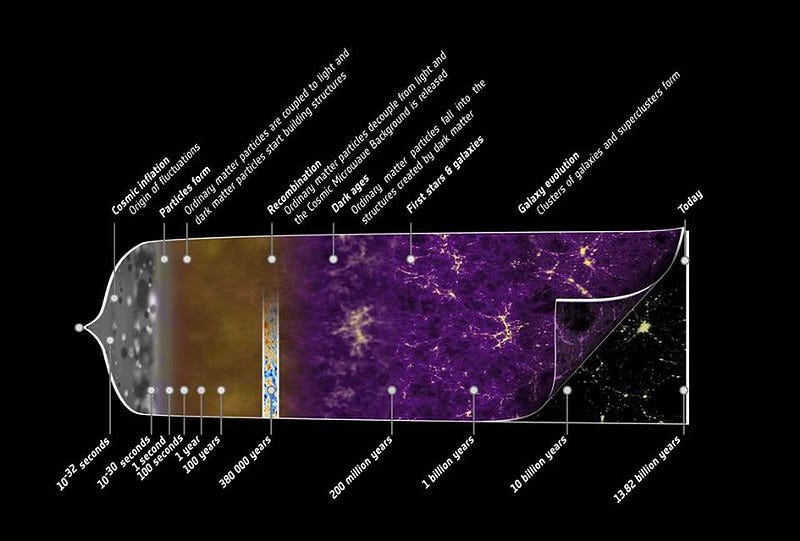
So what happened to start inflation off? There’s a tremendous amount of research and speculation about it, but nobody knows. There is no evidence we can point to; no observations we can make; no experiments we can perform. Some people (wrongly) say something akin to:
Well, we had a Big Bang singularity give rise to the hot, dense, expanding Universe before we knew about inflation, and inflation just represents an intermediate step. Therefore, it goes: singularity, inflation, and then the hot Big Bang.
There are even some very famous graphics put out by top cosmologists that illustrate this picture. But that doesn’t mean this is right.
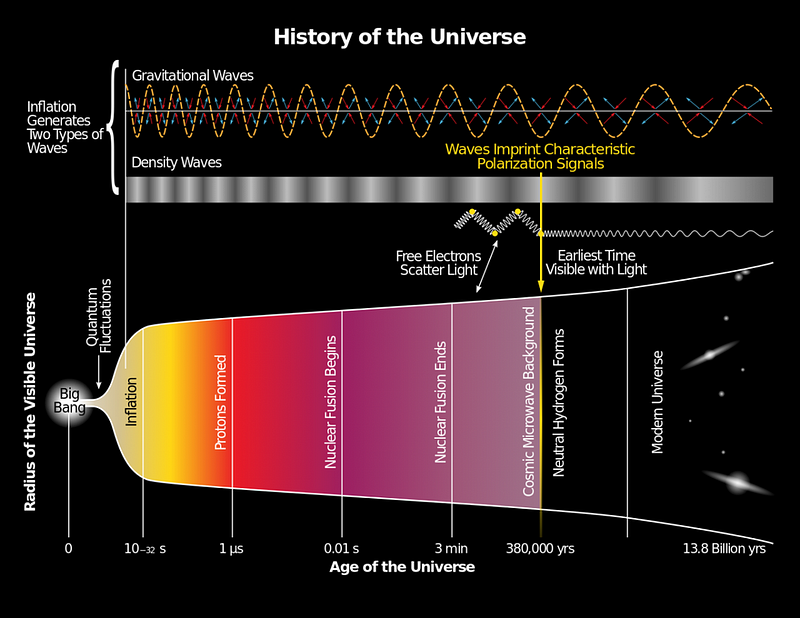
In fact, there are very good reasons to believe that this isn’t right! One thing that we can mathematically demonstrate, in fact, is that it’s impossible for an inflating state to arise from a singularity. Here’s why: space expands at an exponential rate during inflation. Think about how an exponential works: after a certain amount of time goes by, the Universe doubles in size. Wait twice as long, and it doubles twice, making it four times as large. Wait three times as long, it doubles thrice, making it 8 times as large. And if you wait 10 or 100 times as long, those doublings make the Universe 2¹⁰ or 2¹⁰⁰ times as large.
Which means if we go backwards in time by that same amount, or twice, or thrice, or 10 or 100 times, the Universe would be smaller, but would never reach a size of 0. Respectively, it would be half, a quarter, an eighth, 2^-10, or 2^-100 times its original size. But no matter how far back you go, you never achieve a singularity.
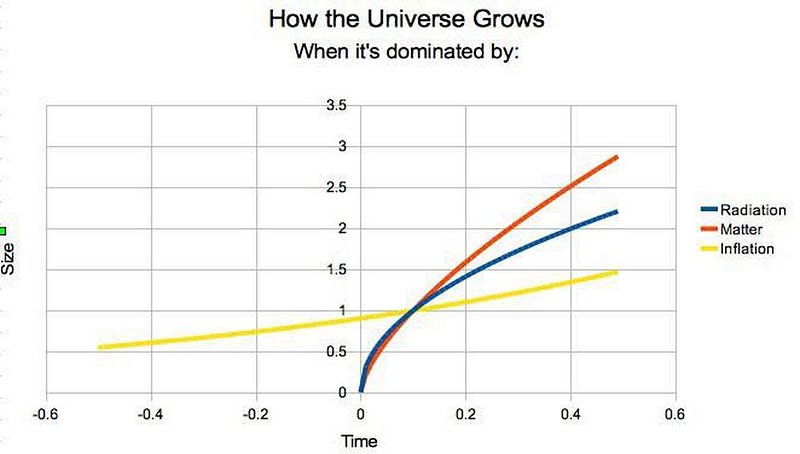
There is a theorem, famous among cosmologists, showing that an inflationary state is past-timelike-incomplete. What this means, explicitly, is that if you have any particles that exist in an inflating Universe, they will eventually meet if you extrapolate back in time. This doesn’t, however, mean that there must have been a singularity, but rather that inflation doesn’t describe everything that occurred in the history of the Universe, like its birth. We also know, for example, that inflation cannot arise from a singular state, because an inflating region must always begin from a finite size.
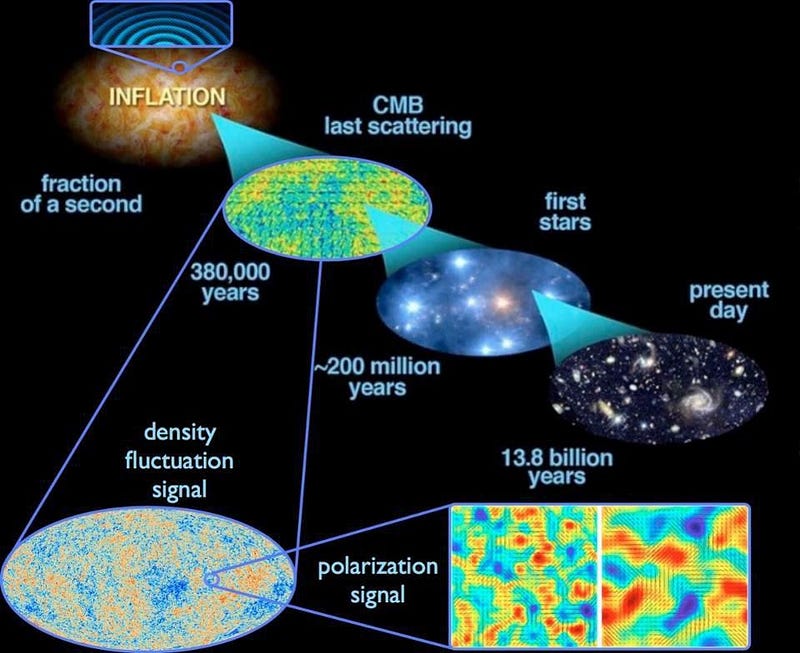
Every time you see a diagram, an article, or a story talking about the “big bang singularity” or any sort of big bang/singularity existing before inflation, know that you’re dealing with an outdated method of thinking. The idea of a Big Bang singularity went out the window as soon as we realized we had a different state — that of cosmic inflation — preceding and setting up the early, hot-and-dense state of the Big Bang. There may have been a singularity at the very beginning of space and time, with inflation arising after that, but there’s no guarantee. In science, there are the things we can test, measure, predict, and confirm or refute, like an inflationary state giving rise to a hot Big Bang. Everything else? It’s nothing more than speculation.
Ethan Siegel is the author of Beyond the Galaxy and Treknology. You can pre-order his third book, currently in development: the Encyclopaedia Cosmologica.