There is sound in space, thanks to gravitational waves
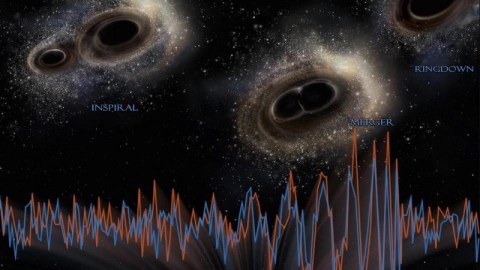
A live-blog event happened a week ago, but you can catch the entire thing anew here, right now!
“We have never observed infinity in nature. Whenever you have infinities in a theory, that’s where the theory fails as a description of nature. And if space was born in the Big Bang, yet is infinite now, we are forced to believe that it’s instantaneously, infinitely big. It seems absurd.” –Janna Levin
It’s long been said that there’s no sound in space, and that’s true, to a point. Conventional sound requires a medium to travel through, and is created when particles compress-and-rarify, making anything from a loud “bang” for a single pulse to a consistent tone for repeating patterns. In space, where there are so few particles that any such signals die away, even solar flares, supernovae, black hole mergers, and other cosmic catastrophes go silent before they’re ever heard. But there’s another type of compression-and-rarefaction that doesn’t require anything other than the fabric of space itself to travel through: gravitational waves. Thanks to the first positive detection results from LIGO, we’re hearing the Universe for the very first time.
Gravitational waves were something that needed to exist for our theory of gravity to be consistent, according to General Relativity. Unlike in Newton’s gravity, where any two masses orbiting one another would remain in that configuration forever, Einstein’s theory predicted that over long enough times, gravitational orbits would decay. For something like the Earth orbiting the Sun, you’d never live to experience it: it would take 10¹⁵⁰ years for Earth to spiral into the Sun. But for more extreme systems, like two neutron stars orbiting one another, we could actually see the orbits decaying over time. In order to conserve energy, Einstein’s theory of gravity predicted that energy must be carried away in the form of gravitational waves.
These waves are maddeningly weak, and their effects on the objects in spacetime are stupendously tiny. But if you know how to listen for them — just as the components of a radio know how to listen for those long-frequency light waves — you can detect these signals and hear them just as you’d hear any other sound. With an amplitude and a frequency, they’re no different from any other wave. General Relativity makes explicit predictions for what these waves should sound like, with the largest wave-generating signals being the easiest ones to detect. The largest amplitude sounds all? It’s the inspiral and merging “chirp” of two black holes that spiral into one another.
In September of 2015, just days after advanced LIGO began collecting data for the first time, a large, unusual signal was spotted. It surprised everyone, because it would have carried so much energy in just a short, 200 millisecond burst, that it would have outshone all the stars in the observable Universe combined. Yet that signal turned out to be robust, and the energy from that burst came from two black holes — of 36 and 29 solar masses — merging into a single 62 solar mass one. Those missing three solar masses? They were converted into pure energy: gravitational waves rippling through the fabric of space. That was the first event LIGO ever detected.
Now it’s over a year later, and LIGO is presently on its second run. Not only have other black hole-black hole mergers been detected, but the future of gravitational wave astronomy is bright, as new detectors will open up our ears to new types of sounds. Space interferometers, like LISA, will have longer baselines and will hear lower frequency sounds: sounds like neutron star mergers, feasting supermassive black holes, and mergers with highly unequal masses. Pulsar timing arrays can measure even lower frequencies, like orbits that take years to complete, such as the supermassive black hole pair: OJ 287. And combinations of new techniques will look for the oldest gravitational waves of all, the relic waves predicted by cosmic inflation, all the way back at the beginning of our Universe.
There’s so much to hear, and we’ve only just started listening for the first time. Thankfully, astrophysicist Janna Levin — author of the fantastic book, Black Hole Blues and Other Songs from Outer Space — is poised to give the public lecture at Perimeter Institute tonight, May 3rd, at 7 PM Eastern / 4 PM Pacific, and it will be live-streamed here and live-blogged by me in real time! Join us then for even more about this incredible topic, and I can’t wait to hear her talk.
The live blog will begin a few minutes prior to 4:00 PM Pacific; join us here and follow along!
3:50 PM: It’s ten minutes until showtime, and to celebrate, here are ten fun facts (or as many as we can get in) about gravity and gravitational waves.
1.) Instead of “action at a distance,” where an invisible force is exerted between masses, general relativity says that matter and energy warp the fabric of spacetime, and that warped spacetime is what manifests itself as gravitation.
2.) Instead of traveling at infinite speed, gravitation only travels at the speed of light.
3.) This is important, because it means that if any changes occur to a massive object’s position, configuration, motion, etc., the ensuing gravitational changes only propagate at the speed of light.
3:54 PM: 4.) This means that gravitational waves, for example, can only propagate at the speed of light. When we “detect” a gravitational wave, we’re detecting the signal from when that mass configuration changed.
5.) The first signal detected by LIGO occurred at a distance of approximately 1.3 billion light years. The Universe was about 10% younger than it is today when that merger occurred.
6.) If gravitation traveled at infinite speed, planetary orbits would be completely unstable. The fact that planets move in ellipses around the Sun mandates that if General Relativity is correct, the speed of gravity must equal the speed of light to an accuracy of about 1%.
3:57 PM: 7.) There are many, many more gravitational wave signals than what LIGO has seen so far; we’ve only detected the easiest signal there is to detect.
8.) What makes a signal “easy” to see is a combination of its amplitude, which is to say, how much it can deform a path-length, or a distance in space, as well as its frequency.
9.) Because LIGO’s arms are only 4 kilometers long, and the mirrors reflect the light thousands of times (but no more), that means LIGO can only detect frequencies of 1 Hz or faster.
10.) For slower signals, we need longer lever-arms and greater sensitivities, and that will mean going to space. That’s the future of gravitational wave astronomy!
4:01 PM: We made it! Time to begin and introduce Janna Levin! (Pronounce “JAN-na”, not “YON-na”, if you were wondering.)
4:05 PM: Here’s the big announcement/shot: the first direct recording of the first gravitational wave. It took 100 years after Einstein first put forth general relativity, and she’s playing a recording! Make sure you go and listen! What does it mean to “hear” a sound in space, after all, and why is this a sound? That’s the purpose, she says, of her talk.
4:08 PM: If you consider what’s out there in the Universe, we had no way of knowing any of this at the time of Galileo. We were thinking about sunspots, Saturn, etc., and were completely unable to conceive of the great cosmic scales or distances. Forget about “conceiving of other galaxies,” we hadn’t conceived of any of this!
4:10 PM: Janna is showing one of my favorite videos (that I recognize) from the Sloan Digital Sky Survey! They took a survey of 400,000 of the nearest galaxies and mapped them in three dimensions. This is what our (nearby) Universe looks like, and as you can see, it really is mostly empty space!
4:12 PM: She makes a really great point that she totally glosses over: only about 1-in-1000 stars will ever become a black hole. There are over 400 stars within 30 light years of us, and zero of them are O or B stars, and zero of them have become black holes. These bluest, most massive and shortest-lived stars are the only ones that will grow into black holes.
4:15 PM: When you consider “where did Einstein’s theory come from,” Janna makes a great point: the idea of the equivalence principle. If you have gravity, you might consider that you feel “heavy” in your chair, for example. But this reaction that you have is the exact same reaction you’d feel if you were accelerating, rather than gravitating. It’s not the gravity that you feel, it’s the effects of the matter around you!
https://www.youtube.com/watch?v=D3LBvh07a1I
4:17 PM: The band OKGO did a video flying in the vomit comet. Janna can’t show the whole thing, with audio, for copyright reasons, and highly recommends it. Luckily for you, thanks to the internet… here it is! Enjoy at your leisure!
4:19 PM: There’s another huge revelation for gravity: the way we understand how things work comes from watching how things fall. The Moon is “falling” around the Earth; Newton realized that. But the Earth is falling around the Sun; the Sun is “falling” around the galaxy; and atoms “fall” here on Earth. But the same rule applies to them all, so long as they’re all in free-fall. Amazing!
4:21 PM: Here’s a fun revelation: stop thinking of a black hole as collapsed, crushed matter, even though that might be how it originated. Instead, think about it as simply a region of empty space with strong gravitational properties. In fact, if all you did was assign “mass” to this region of space, that would perfectly define a Schwarzschild (non-charged, non-rotating) black hole.
4:23 PM: If you were to fall into a black hole the mass of the Sun, you’d have about a microsecond, from crossing the event horizon (according to Janna) until you were crushed to death at the singularity. This is consistent with what I once calculated, where, for the black hole at the center of the Milky Way, we’d have about 10 seconds. Since the Milky Way’s black hole is 4,000,000 times as massive as our Sun, the math kind of works out!
4:26 PM: How would you detect a gravitational wave? Honestly, it would be like being on the surface of the ocean; you’d bob up and down along the surface of space, and there was a big argument in the community as to whether these waves were real or not. It wasn’t until Joe Weber came along and decided to try and measure these gravitational waves, using a phenomenal device — an aluminum bar — that would vibrate if a rippling wave “plucked” the bar very slightly.
Weber saw many such signals that he identified with gravitational waves, but these, unfortunately, were never reproduced or verified. He was, for all of his cleverness, not a very careful experimenter.
4:29 PM: There’s a good question from Jon Groubert on twitter: “I have a question about something she said — there is something inside a black hole, isn’t there? Like a heavy neutron star.” There should be a singularity, which is either point-like (for a non-rotating singularity) or a one-dimensional ring (for a rotating one), but not condensed, collapsed, three-dimensional matter.
Why not?
Because in order to remain as a structure, a force needs to propagate and be transmitted between particles. But particles can only transmit forces at the speed of light. But nothing, not even light, can move “outward” towards the exit of a black hole; everything moves towards the singularity. And so nothing can hold itself up, and everything collapses into the singularity. Sad, but the physics makes this inevitable.
4:32 PM: After Weber’s failures (and fall from fame), the idea of LIGO came along by Rai Weiss in the 1970s. It took more than 40 years for LIGO to come to fruition (and over 1,000 people to make it happen), but the most fantastic thing was that it was experimentally possible. By making two very long lever-arms, you could see the effect of a passing gravitational wave.
4:34 PM: This is my favorite video illustrating what a gravitational wave does. It moves space itself (and everything in it) back and forth by a tiny amount. If you have a laser interferometer set up (like LIGO), it can detect these vibrations. But if you were close enough and your ears were sensitive enough, you could feel this motion in your eardrum!
4:35 PM: I’ve got some really good headphones, Perimeter, but unfortunately I can’t hear the different gravitational wave model signals that Janna is playing!
4:38 PM: It’s funny to think that this is the world’s most advanced vacuum, inside the LIGO detectors. Yet birds, rats, mice, etc., are all under there, and they chew their way into almost the vacuum chamber that the light travels through. But if the vacuum had been broken (it’s been constant since 1998), the experiment would have been over. In Louisiana, hunters shot at the LIGO tunnels. It’s horrifying how sensitive and expensive this equipment is, but yet how fragile it all is, too.
4:41 PM: Janna is doing a really great job telling this story in a suspenseful but very human way. We only saw the final few orbits of two orbiting black holes, drastically slowed down in the above movie. They were only a few hundred kilometers apart, those final four orbits took 200 millisecond, and that’s the entirety of the signal that LIGO saw.
4:43 PM: If you’re having trouble listening/hearing the events in the talk, listen to this video (above), in both natural pitch and increased pitch. The smaller black holes (roughly 8 and 13 solar masses) from December 26, 2015, are both quieter and higher pitched than the larger ones (29 and 36 solar masses) from September 14th in the same year.
4:46 PM: Just a little correction: Janna says this was the most powerful event ever detected since the Big Bang. And that’s only technically true, because of the limits of our detection.
When we get any black hole mergers, approximately 10% of the mass of the least massive black hole in a merger pair gets converted into pure energy via Einstein’s E = mc2. 29 solar masses is a lot, but there are going to be black holes of hundreds of millions or even billions of solar masses that have merged together. And we have proof.
4:49 PM: This is OJ 287, where a 150 million solar mass black hole orbits an ~18 billion solar mass black hole. It takes 11 years for a complete orbit to occur, and General Relativity predicts a precession of 270 degrees per orbit here, compared to 43 arc seconds per century for Mercury.
4:51 PM: Janna did an incredible job ending on time here; I’ve never seen an hour talk actually end after 50 minutes at a Perimeter public lecture. Wow!
4:52 PM: What would happen if Earth got sucked up into a black hole? (Q&A question from Max.) Although Janna’s giving a great answer, I’d like to point out that, from a gravitational wave point of view, Earth would be shredded apart, and we’d get a “smeared out” wave signal, that would be a much noisier, static-y signal. Once Earth got swallowed, the event horizon would grow just a tiny bit, as an extra three millionths of a solar mass increased the black hole’s radius by just that tiny, corresponding amount.
4:55 PM: What a fun talk, a great and snappy Q&A session, and a great experience overall. Enjoy it again and again, because the video of the talk is now embedded as a permalink. And thanks for tuning in!
Ethan Siegel is the author of Beyond the Galaxy and Treknology. You can pre-order his third book, currently in development: the Encyclopaedia Cosmologica.