The Stellar Story of Us
How the Universe made the elements and atoms that make up you and me, and everything else on Earth.
“Things are the way they are because they were the way they were.” -Fred Hoyle
When you look out at the world today — whether you’re looking at what the Earth has to offer or far beyond it into the Universe — there’s no denying that there’s an incredible wealth of diversity out there to be aware of, and to appreciate.
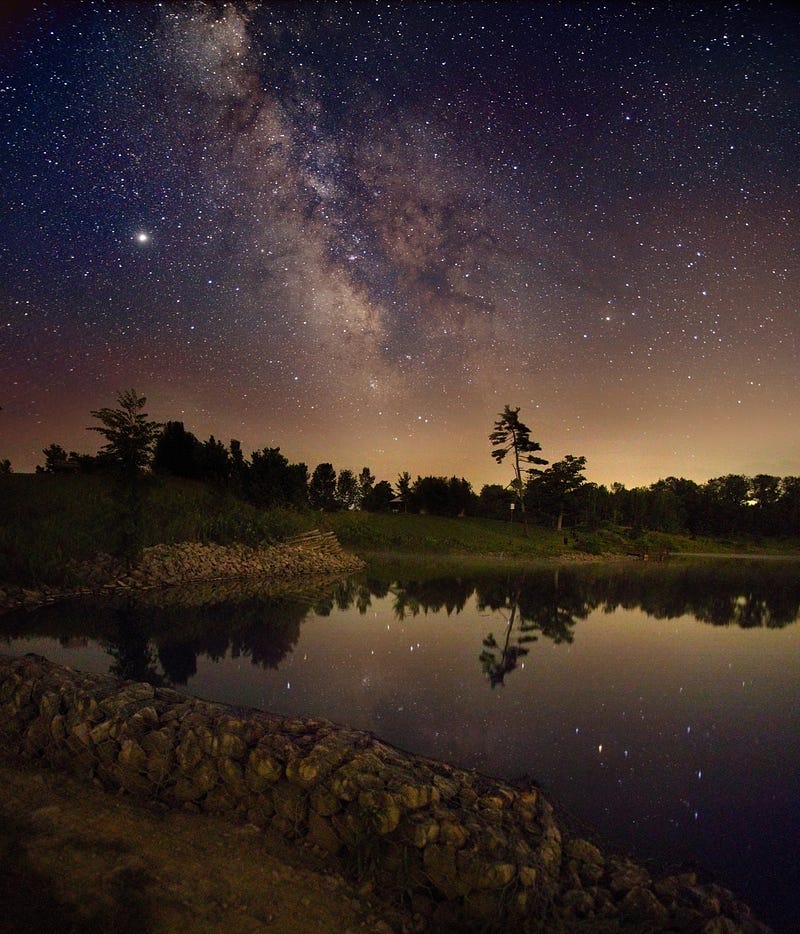
But when you look back at the first elements in the Universe — at the atomic nuclei that define the properties of atoms as they first existed — you find that this wondrous world with all its varied chemical bonds and molecular intricacies would have been all but impossible!
You see, our planet alone is home to some 91 naturally occurring elements, at least 59 of which are represented in each and every human body. These elements have a variety of physical and chemical properties, and each one is uniquely defined by the number of protons in its atomic nucleus. We typically classify these elements in the format you see below: the periodic table!

Our observable Universe, as far as we can tell, contains some 10^80 atoms in it, whose existence is only enabled by a fundamental matter-antimatter asymmetry that is only partially understood. In the hot, dense, early stages of the Big Bang, the primordial protons-and-neutrons were able to come together to create some helium-4, some trace isotopes of hydrogen and helium, and a tiny amount of lithium (and probably beryllium) to go along with a Universe still mostly made up of lone protons.
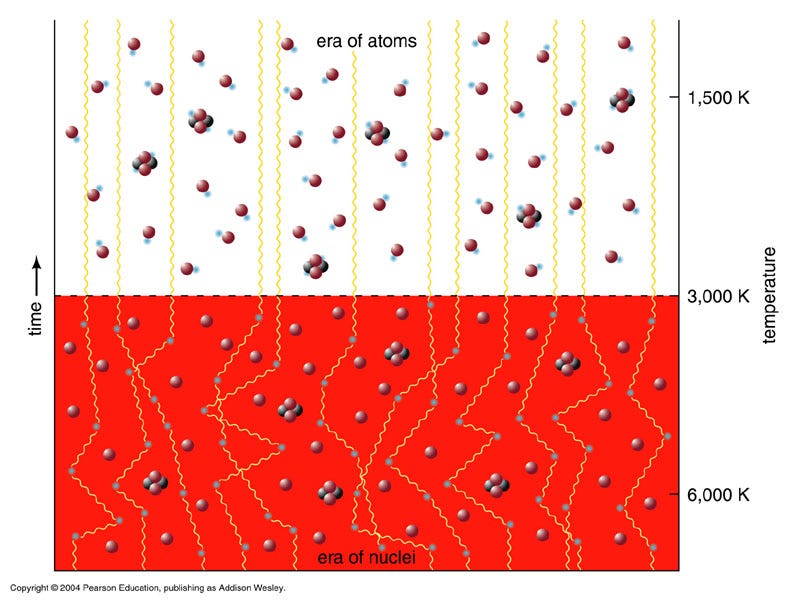
When the Universe cooled enough so that neutral atoms could form, the helium-4 nuclei and protons picked up electrons, forming common helium and hydrogen as we know them. Combined those two elements made up over 99.99% of the Universe at the time, with a few thousandths of a percent in other isotopes of helium and hydrogen and a few atoms per billion winding up as lithium, which beryllium-7 eventually decays into.
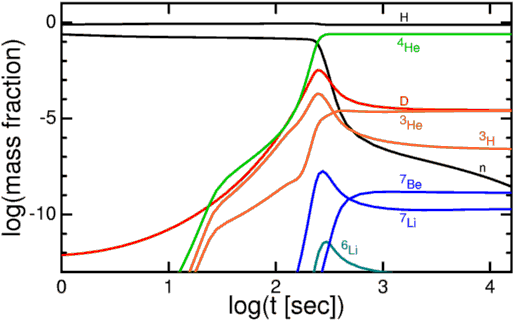
But what of all the other elements in the Universe? For the first few millions of years of our natural history, they simply didn’t exist; not a single atom of carbon, nitrogen, oxygen or the other elements we’re so familiar with was around. 13.8 billion years on, those “heavier” elements, the ones heavier than helium — known as metals in astronomy circles — make up about 1-2 percent of the Universe, by mass.
But that’s a very important 1-2 percent; it’s responsible for all the rocky planets and everything interesting we know about them!!!
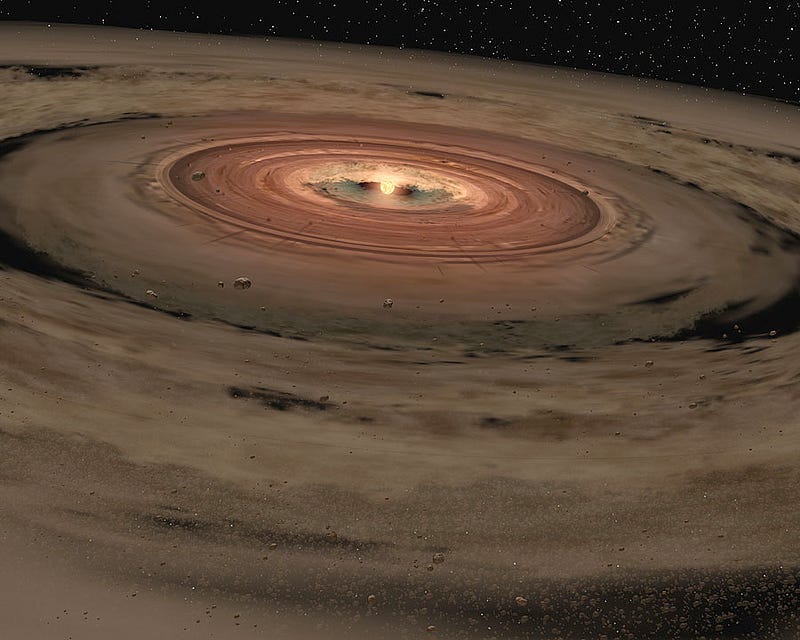
Where, then, did these heavier elements come from?
Believe it or not, we owe the existence of every one of these heavier elements to the cores of massive stars! Let’s take a look and see how that came to be.

All stars originated as giant molecular clouds of gas, which will gravitationally collapse under the right conditions (and given millions of years), giving rise to extremely overdense regions within it. As the densities and temperatures of the most dense regions in the cloud continue to increase, the most energetic particles contained within become ionized, and eventually reach a critical temperature where the hydrogen inside can begin a fusion chain where it winds up as helium!
Every star of above 0.08 solar masses — and our Sun is a G-class example — began their life in this way.
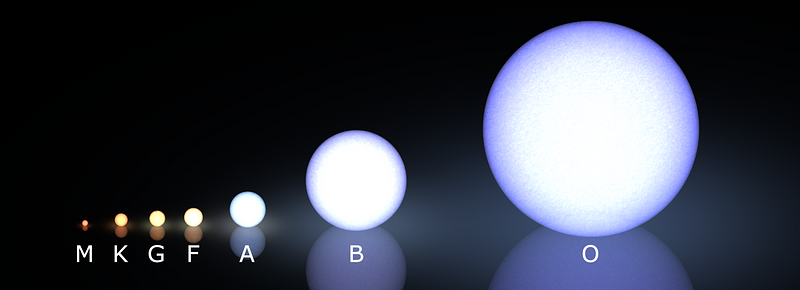
For the M-class stars — the reddest, coolest and least massive of the stars — helium is the end of the line. When the hydrogen fuel in their core is gone, the core will contract and heat up, but the temperatures it will reach are woefully insufficient for creating heavier elements. Instead, we’ll just wind up with a degenerate ball of helium: a white dwarf. These objects are tens-to-hundreds of thousands times the mass of Earth, but approximately the same physical size as our planet, and not the originators of the heavy elements we’re seeking.
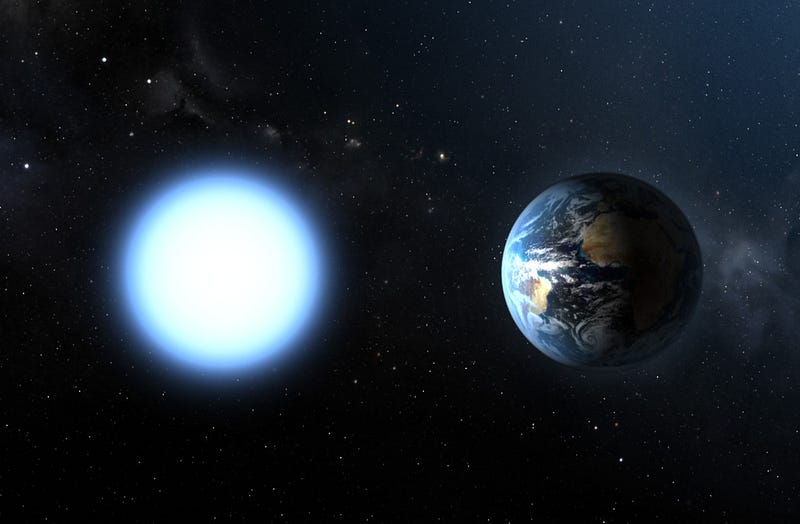
Heavier stars, on the other hand, get a lot more interesting very quickly. When a K-class star (or larger) runs out of hydrogen fuel in its core, all the radiation pressure that came about from nuclear fusion suddenly drops, and the star’s core can no longer sustain itself against gravitational collapse. As the core rapidly contracts, it heats up, increasing its temperature by tens of millions of degrees.
And in a star that’s 40 percent (or more) of the Sun’s mass, a very rare and special process begins to take place.
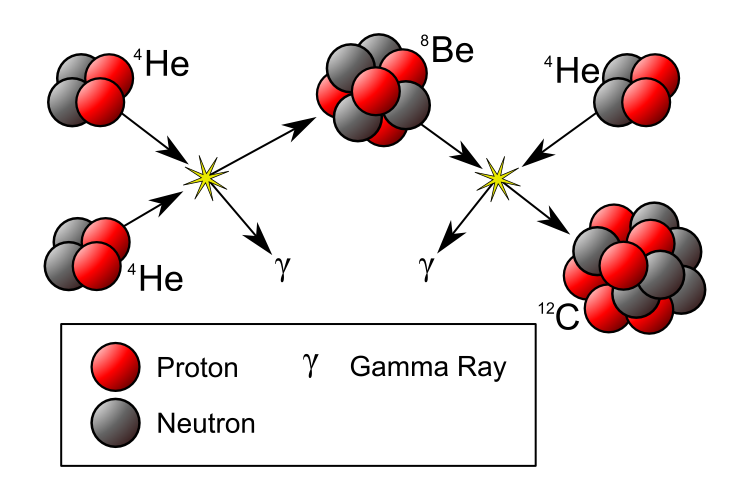
Two helium-4 nuclei can fuse together to create beryllium-8, a wildly unstable isotope of our periodic table’s fourth element. With a mean lifetime of less than 10^-16 seconds, it might seem that there’s no way to do anything with it before it decays back into helium-4s. Yet under the right conditions — conditions that demand tremendous temperatures and densities — a third helium-4 can get in there fast enough to create an excited state of carbon-12, which is the first stable, heavy element created in abundance! As this process takes off, these stars enter the red giant phases of their lives.
Stars that can fuse helium into carbon can also produce oxygen in the core, but as we get to greater masses (and higher temperatures), the continued addition of helium-4 to the nuclei allow us to climb the periodic table in steps of two!
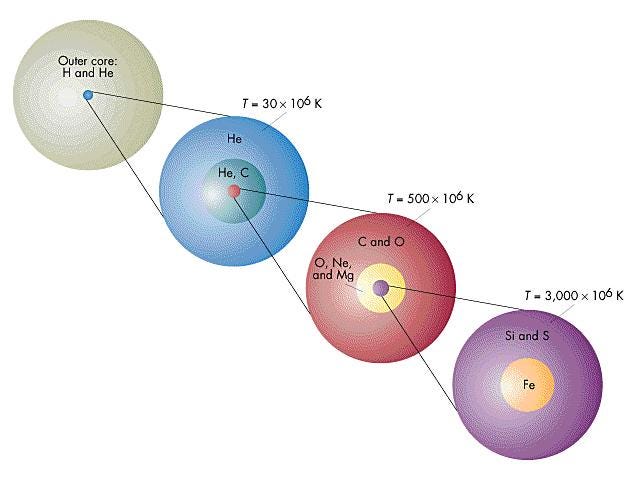
Our Sun will likely stop at neon, while a star like Sirius might make it all the way up to silicon and sulphur, and the brightest stars in the Pleiades will make it all the way to iron. Regardless, when any star that began as a K, G, F, A, or the lower-mass B-class stars runs out of its fusible material in its innermost core, gravitational collapse once again ensues, creating a white dwarf at the center and causing the outer layers to blow off in a planetary nebula.
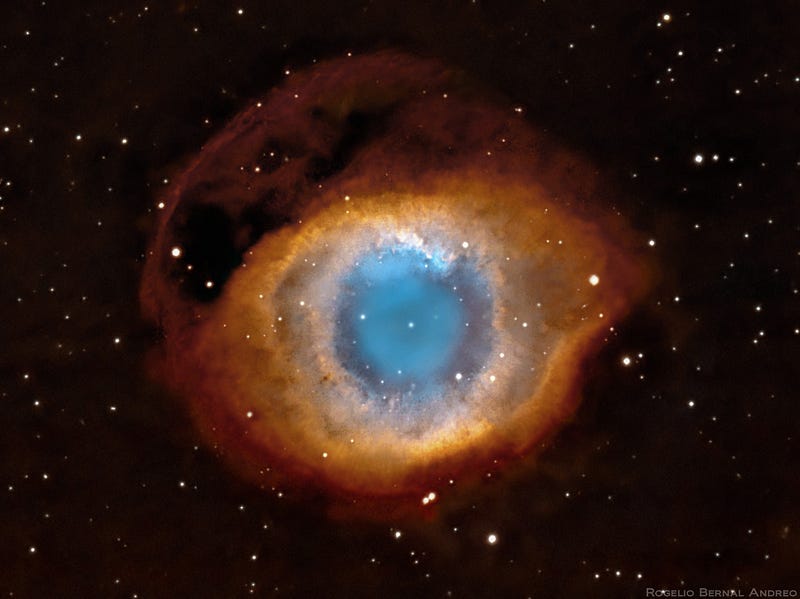
The different colors you see indicate the presence of different atoms, and can include elements all the way up to iron, nickel and cobalt. But if this were the primary way the Universe had of enriching itself, our world would look very different, as it would still be mostly hydrogen and helium, and would have practically none of the elements higher up in the periodic table.
To create those, we need to go to the most massive stars in the Universe: the brightest, bluest and shortest lived stars: the O-class and heaviest B-class stars!
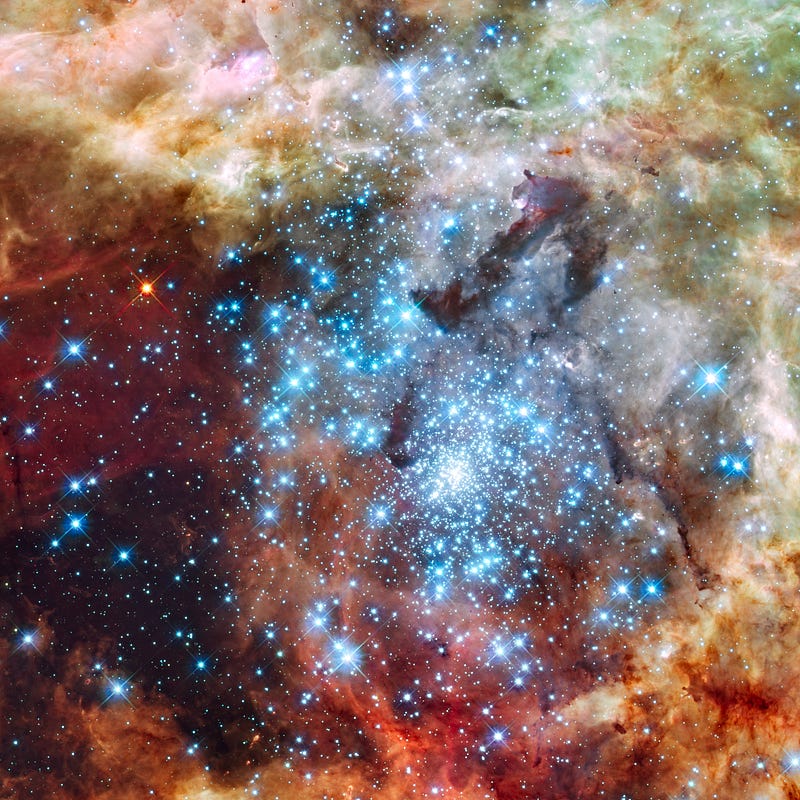
Acknowledgment: R. O’Connell (University of Virginia) and the Wide Field Camera 3 Science Oversight Committee.
These cosmic behemoths have no problem reaching iron in their core, and their interiors take on a shell-like appearance, with the innermost layers containing progressively heavier and heavier elements. Each shell continues nuclear fusion throughout the life of the star, and the temperatures are so great that a large number of free neutrons are produced as well.
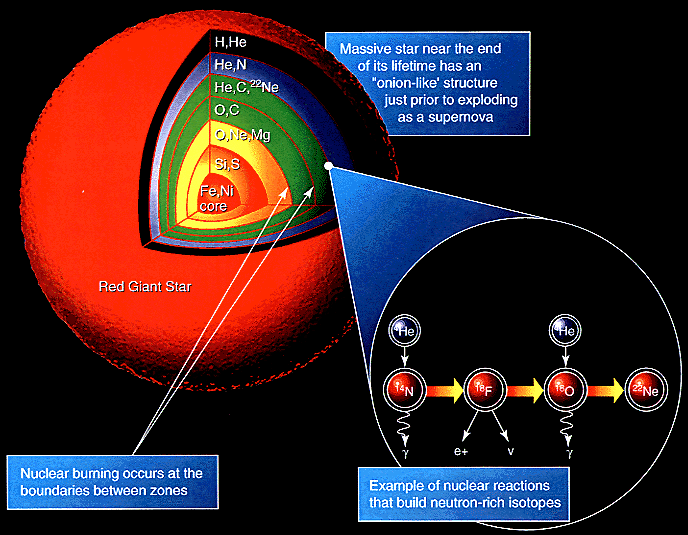
While the star is still burning through this fuel, neutrons can be added to nuclei slowly (known as the s-process), creating the odd-numbered elements in some abundance and also the first elements with atomic numbers into the 30s and 40s.
But when the inert core — which won’t fuse any further due to a “plateau” in binding-energy-per-nucleon — becomes massive enough and starts to contract, suddenly the very atoms themselves are unable to withstand gravitational collapse! The result is a runaway nuclear fusion reaction, and this time the core doesn’t just contract down, but rather the elements within fuse into a ball of pure neutrons!
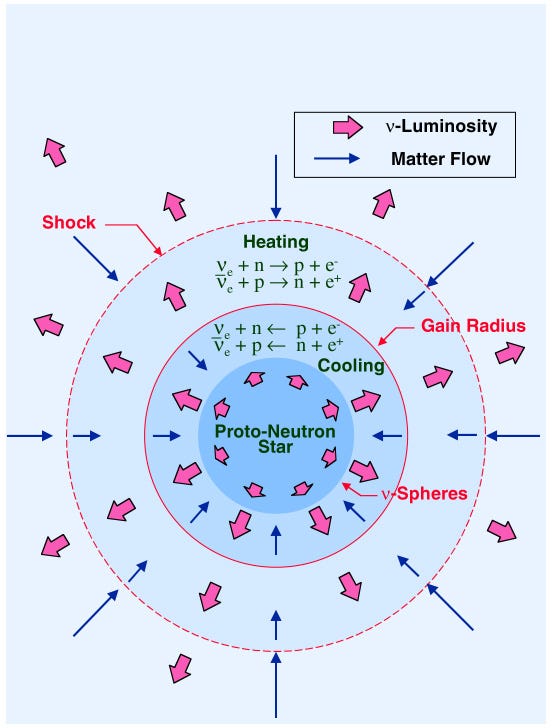
This time, there is nothing to prevent runaway gravitational collapse, and the core of the star shrinks all the way down to just a few kilometers in radius — a neutron star — or if it’s even more massive, a black hole! But the outer layers are where the most interesting physics happens.
A huge number of neutrons now bombard these heavy elements, at temperatures and energies not seen in the Universe since the very early stages of the Big Bang. And rather than slowly, the elements climb up the periodic table incredibly rapidly (through the r-process), creating all the elements of the periodic table and scattering them throughout interstellar space!
This is how the Universe became enriched; this is where the vast majority of heavy elements in the Universe came from! After many generations of stars have lived and died, the interstellar medium becomes rich with these heavy elements. While the most unstable ones (everything above plutonium in the periodic table) decay away relatively quickly, the vast majority of them stick around long enough to be detected naturally, especially if we look towards the center of the galaxy, where star formation and destruction is rampant.
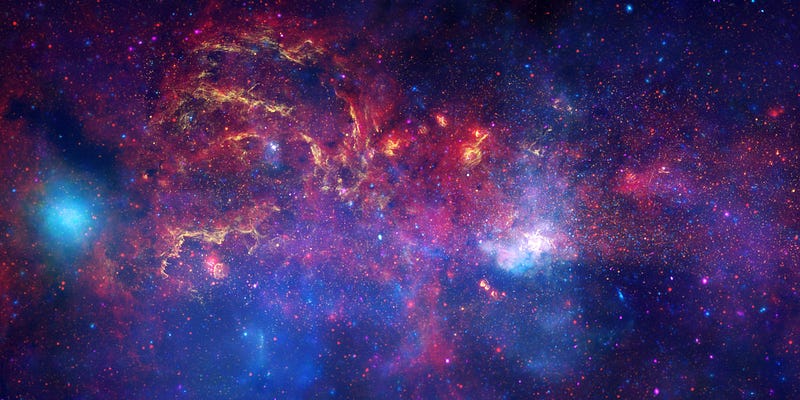
If we study the Solar System and ask what the typical abundance is of each type of atom, this is what we find. Notice the “sawtooth” pattern that favors even-numbered elements over odd-numbered elements; the fact that helium-4 plays such an essential role in building the heavier elements is the culprit here!
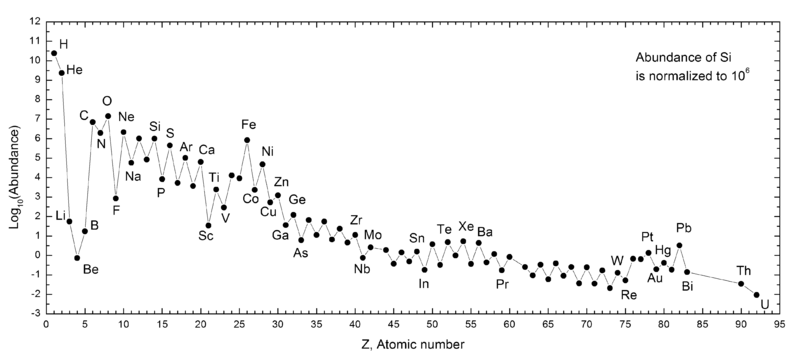
And it’s this very process — how the most massive stars fused elements in their cores, died in supernovae explosions, and enriched the Universe with heavier atoms — that allowed the Universe to create rocky planets, advanced chemicals, and in the end, life. That’s how we went from hydrogen, helium and not much else to the entire Universe we know today.
And that’s the stellar story of us!