The Physics of Wormholes
The fastest way to make interstellar travel a reality might not be only science fiction for long!
It is humanity’s longstanding dream to venture to the stars. We long to set foot on other planets and potentially contact alien civilizations, assuming they are friendly. Yet our dreams have been dashed by the harsh reality of ordinary space travel’s limitations.
It took the Apollo astronauts, in the only manned missions to another world so far, more than three days to reach the moon. At the same speed, it would take millions of years to reach Proxima Centauri, the nearest star to the Sun.
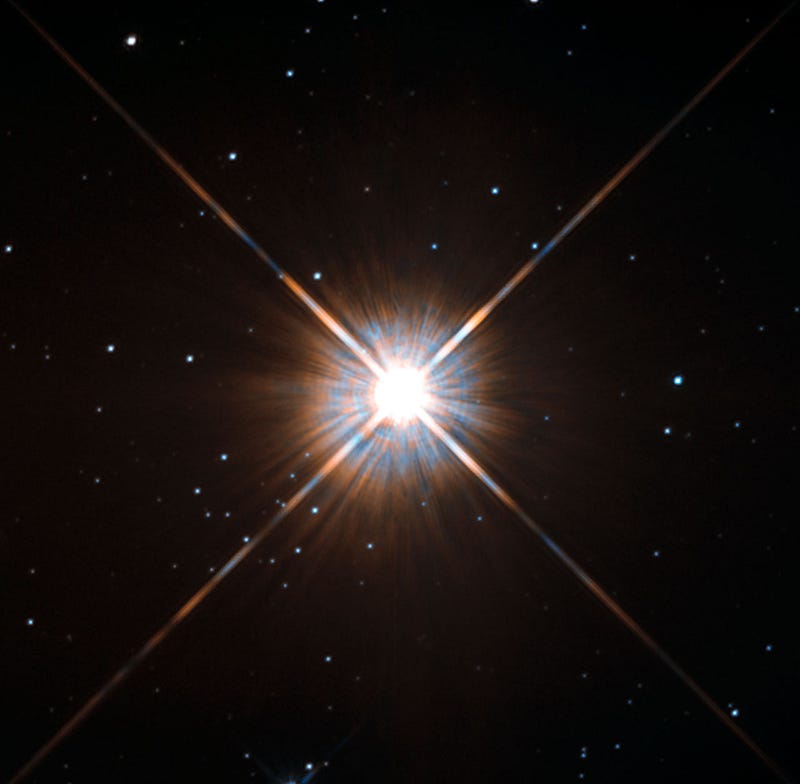
We can envision advances in propulsion technology that could boost that languid velocity to a more robust pace; but a factor of millions offers a daunting challenge. Even if, somehow, we managed to conquer such a formidable barrier, what about journeys to even farther stars?
If you’re thinking that it would take an Einstein to develop such a method, it was in fact the founder of relativity who first envisioned the spatial shortcuts that might someday make interstellar travel possible. Such hypothetical connections between two otherwise separate parts of the sheet of our universe, now known as wormholes, were originally referred to as Einstein-Rosen bridges. They were proposed by Einstein and his assistant Nathan Rosen in a classic 1935 paper called The Particle Problem in the General Theory of Relativity.
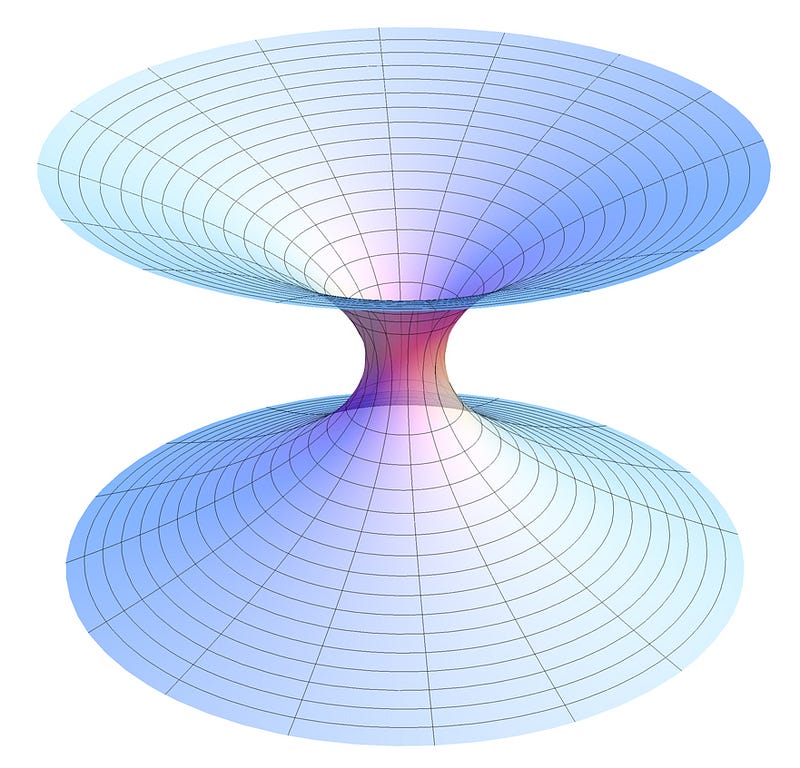
Einstein’s motivation for proposing these connections had nothing to do with space travel. At that point in his career, he was striving to perform a kind of magic trick to transform his masterful general theory of relativity from a balance of substance and geometry to a “purer” vision of geometry alone.
In the standard approach to general relativity, clumps of matter and energy weigh down upon the fabric of reality, distorting it, and causing other objects to divert in their paths. Matter warps geometry, which, in turn, guides matter. It is like an acrobat jumping onto a trampoline, bending it downward, and forcing another performer, walking on that slanted surface, to take a wobbly route around him instead of a straight line.
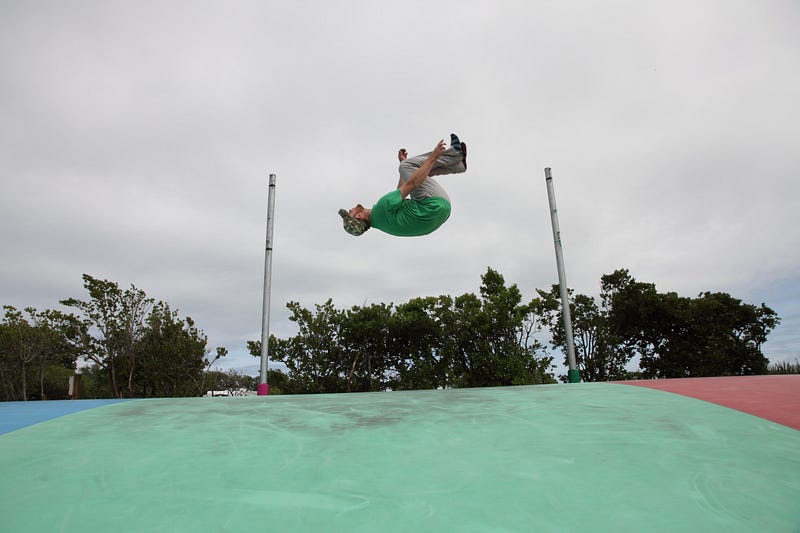
Similarly, in the solar system, the Sun warps spacetime in its vicinity, leading the planets to follow elliptical paths rather than linear routes.
The gravitational field has its own energy, yet is represented on the geometry side of Einstein’s equations, instead of the matter and energy side. Einstein felt this to be an imbalance. Envisioning a theory of everything that would model the entire universe, including its content, through sheer geometry, Einstein looked for solutions of the general relativity that resembled particles. He and Rosen discovered bridgelike connections between disparate parts of the cosmic cloth and hoped those would do the trick. The links bore little resemblance to actual particles, however, and they ultimately abandoned the idea.
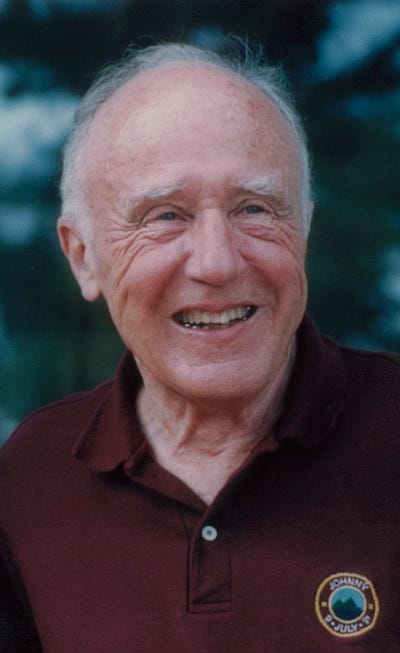
Through the late 1950s and 1960s, Princeton physicist John Wheeler embarked upon a similar quest — the geometrization of reality — calling it geometrodynamics. One major difference between Einstein’s approach and Wheeler’s is that while the former opposed probabilistic quantum mechanics, the latter embraced it and hoped to find a quantum theory of geometry representing everything under the sun.
Dubbing Einstein-Rosen bridges, “wormholes,” Wheeler sought a way that particles would emerge from a kind of spacetime foam as similar entities.
These wormholes would randomly appear as quantum fluctuations in the froth of geometry. Field lines would loop through the wormholes to produce known particle properties such as charge. In such fashion, order would arise from sheer randomness.
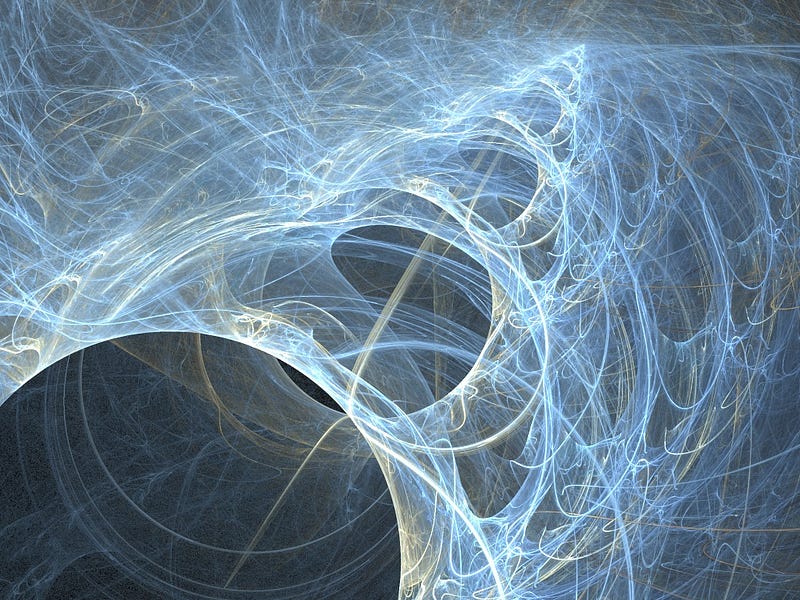
One simple type of wormhole connects Schwarzschild solutions of general relativity in two different sheets. The Schwarzschild solution represents the space-warping effects of a static, uncharged sphere of mass. Wheeler recognized that it also offers a way of modeling the end states of highly compact, collapsed stellar cores — what he called “black holes.” Thus black holes in separate parts of the universe could, in principle, be linked by a wormhole connection. However, further analysis convinced Wheeler that such connections would be unstable.
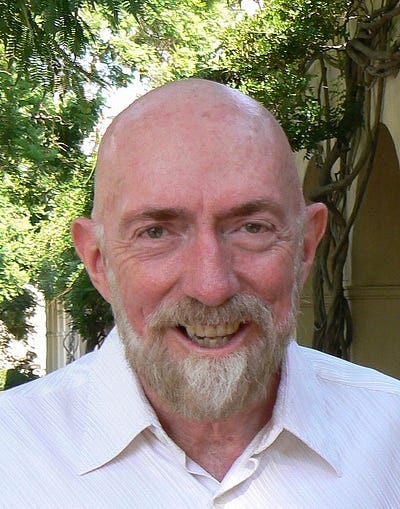
In the late 1980s, Caltech physicist Kip Thorne, who had been a PhD student of Wheeler’s and a collaborator with him on a popular general relativity textbook, took up the topic of wormholes for another purpose: exploring their potential as interstellar shortcuts.
Thorne’s motivation was a query by Carl Sagan. While working on the novel Contact, about a first encounter between humans and an extraterrestrial civilization, Sagan needed a plot device that would enable a rapid transgalactic journey. He thought about Schwarzschild wormholes spawned in black holes, but Thorne convinced him that they wouldn’t work. Even if they could somehow by stabilized, black hole connections would crush and irradiate voyagers. Hapless astronauts would be stretched out and burnt like deep-fried Twizzlers as they approached the black hole’s central singularity, which is a point of infinite density. In short, Schwarzschild black hole wormholes would be death traps, not speedways. (Rotating or “Kerr” black holes, another type, have ring, rather than point singularities, and could potentially allow survival under some circumstances.)
Thorne thought hard about how to fulfill Sagan’s goal of a family-friendly (or at least seasoned astronaut-friendly) wormhole. He assigned the problem to his research student Michael Morris. General relativity’s magnificent flexibility offers the possibility of constructing virtually any type of geometry by shaping the distribution of mass and energy into the right configuration.
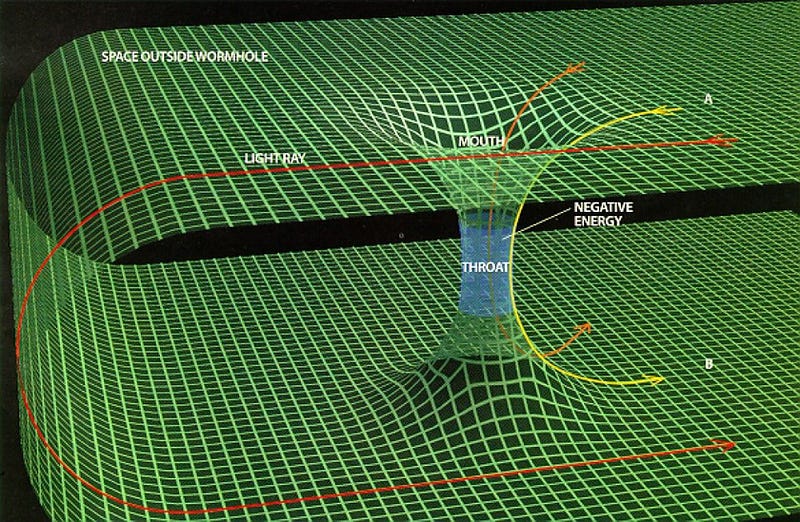
Stunningly, Morris and Thorne discovered a general relativistic wormhole solution with many favorable properties. It allowed passage between two different parts of space through a kind of stable “throat” that would not collapse when travelers passed though.
Rather than being stretched out or crushed, voyagers would safely travel from one end to the other in a reasonable amount of time — say, less than a year. Radiation would be kept to a minimum. Sagan was thrilled, and incorporated Morris and Thorne’s scheme into his plot. A movie version of Contact, starring Jodie Foster, proved a smashing success.
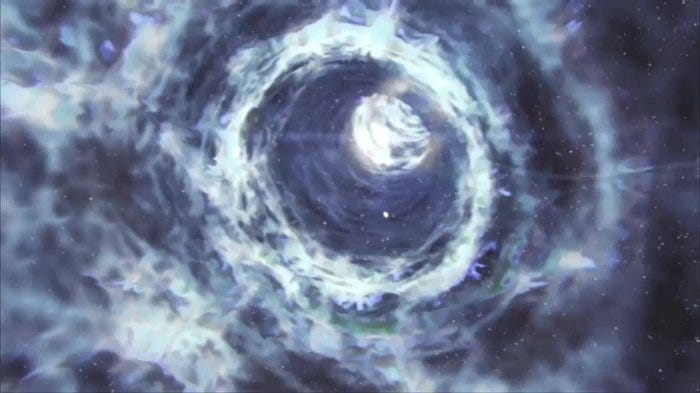
Moreover, Morris and Thorne’s paper, “Wormholes in spacetime and their use for interstellar travel: A tool for teaching general relativity,” and subsequent work brought the idea of traversable wormholes into mainstream physics journals. What a triumph!
The development of traversable wormhole solutions, as hypothetical as they were, unleashed a flood of pent-up aspirations for interstellar travel. How awesome it would be to imagine a subway-like network connecting far-flung parts of the cosmos. If Earth were in peril, a wormhole could theoretically offer an escape route. Alien civilizations hundreds or thousands of light years away might suddenly come within reach. In addition to Contact, traversable wormholes have inspired numerous science fiction stories, television shows and films, most recently Interstellar (for which Thorne is executive producer and main scientific consultant).
However, as Morris and Thorne had revealed, and further studies have confirmed, to construct traversable wormholes requires an ingredient nature seems to lack — at least in large quantities — something called “exotic matter.” Exotic matter possesses a negative mass, and thus functions as a kind of antigravity stabilizing mechanism to help prop the wormhole’s throat open.
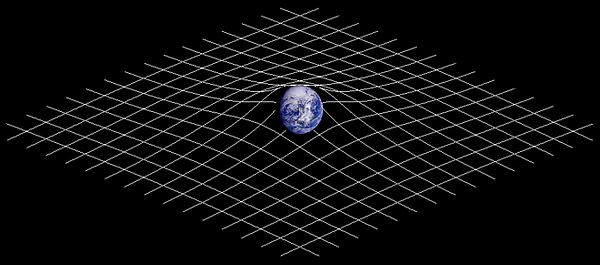
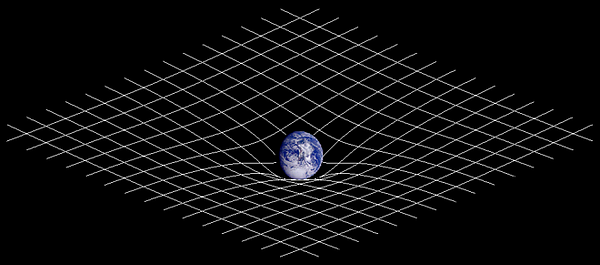
At first glance, negative mass seems impossible. No matter how much someone diets, he’d never tip the scales at minus 50 kilos. Yet, following Einstein’s famous relationship, mass is related to energy. Moreover, as Morris and Thorne realized, the Casimir effect of quantum physics permits a kind of negative energy. So perhaps negative energy could be transformed into negative mass.
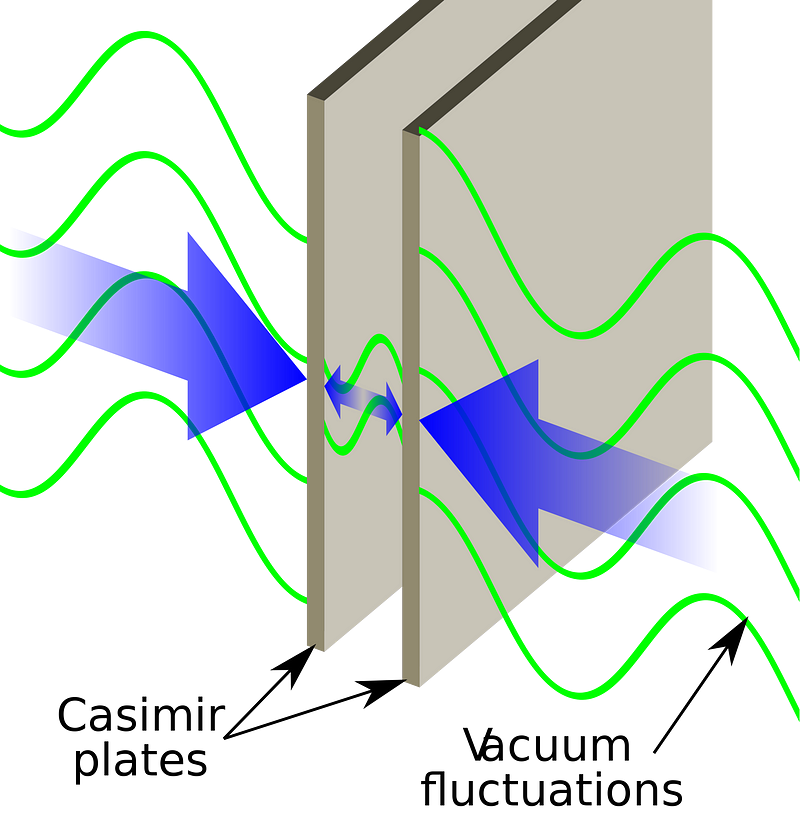
The Casimir effect has to do with the fact that the quantum vacuum is brimming with energetic fluctuations. Dutch physicist Hendrik Casimir realized that if you take two metal plates, with pure vacuum in between them, and bring them closer, the vacuum is “squeezed” and there are fewer allowed modes of fluctuation. The result is that the vacuum energy between the plates drops below that of the surroundings. If the surrounding energy is zero, the quantum vacuum between the plates acquires a negative energy. The energy difference between the exterior and interior results in a kind of negative pressure, bringing the plates together.
Morris and Thorne suggested that regions of the quantum vacuum with negative energy could conceivably be “mined” to produce exotic matter. Consequently, perhaps exotic matter is not so far-fetched.
Another possibility that has emerged since the discovery of cosmic acceleration in 1998 is that the dark energy driving that antigravity repulsion might somehow be connected to exotic matter, given that each might be related to the Casimir effect and the notion of negative pressure. The jury is still out on what dark energy actually consists of, however, so don’t rely on that prospect.
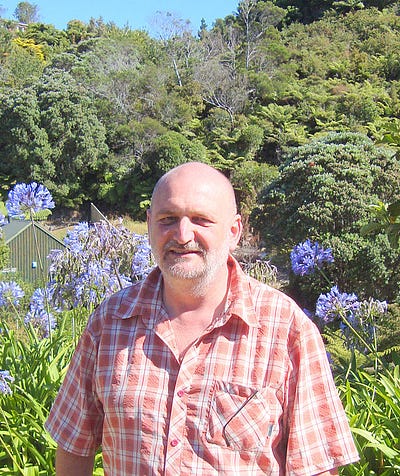
Could researchers generate enough exotic matter from the quantum vacuum to fashion a traversable wormhole? That sounds like a formidable task. Fortunately, New Zealand theorist Matt Visser has developed alternative wormhole scenarios that only require a smattering of exotic matter.
Even if exotic matter is identified and put to use, there is another obstacle to traversable wormhole construction — the enormous amount of ordinary matter required. Researchers estimate that one would need a glob of mass comparable to millions of suns. Clearly, wormhole construction is not in the cards for the foreseeable future.
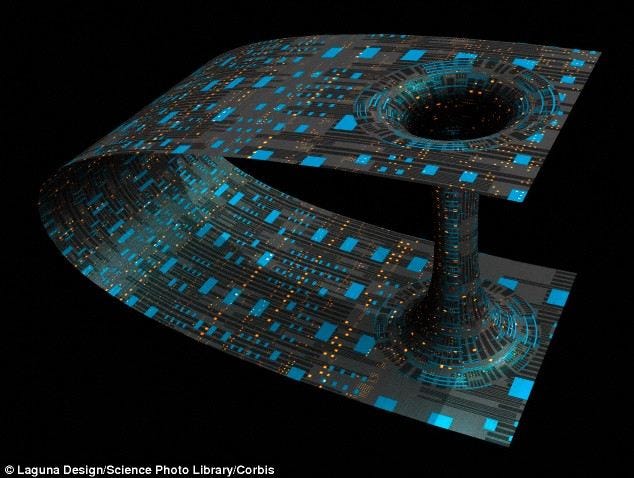
That said, it is possible that traversable wormholes exist naturally. If so, perhaps we are lucky enough that one exists within a reasonable jaunt (yet far enough away that its massive gravitational pull doesn’t affect the solar system). Could we find one and use it to explore the galaxy? Perhaps someday such a science fiction fantasy will turn into reality and far-flung civilizations will finally be within our reach.
This post was written by Paul Halpern, Professor of Physics at the University of the Sciences in Philadelphia, PA, science writer and author. Follow Paul on Twitter at @phalpern.
If you enjoyed this, leave your comments on the Starts With A Bang forum here!