The One Big Problem With All Alternatives To Dark Matter And Dark Energy
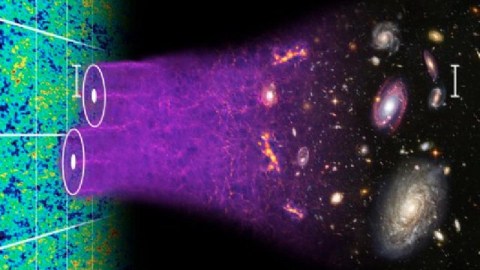
It might not feel that way, but a 95% dark Universe really is the best game in town.
No matter how much we might try and hide it, there’s an enormous problem staring us all in the face when it comes to the Universe. If we understood just three things:
- the laws that govern the Universe,
- the components that make up the Universe,
- and the conditions that the Universe started off with,
we’d be able to do the most remarkable thing of all. We could write down a system of equations that, with a powerful enough computer at our disposal, would describe how the Universe evolved over time to transform from those initial conditions into the Universe we see today.
Every single event that occurred in our cosmic history — to the limits of classical chaos and quantum indeterminism — could be known and described in great detail, from the individual interactions between quantum particles to the largest cosmic scales of all. The problem we face, when we try to do exactly that, is that despite all we know about the Universe, what we predict and what we observe don’t quite match up unless we add at least two mystery ingredients: some type of dark matter and some type of dark energy. It’s a remarkable puzzle to solve, and something every astrophysicist has to reckon with. While many love to present alternatives, they’re all even worse than the unsatisfying fix of dark matter and energy. Here’s the science of why.
There are a whole slew of measurements we can make that have helped reveal the nature of the Universe. We’ve measured the orbits of the planets and the deflection of light due to the presence of mass, which showed that Einstein’s General Relativity and not Newton’s laws of universal gravitation best describes our reality. We’ve uncovered the behavior of subatomic particles, antiparticles, and photons, revealing the quantum forces and fields that govern our Universe. If we want to simulate how the Universe evolved through time, we have to take the known, demonstrably correct laws on the scales we’ve tested them and apply them to the cosmos as a whole.
We’ve also been able to measure a whole series of properties about all the objects we can observe throughout the Universe. We’ve learned how stars shine and emit light, and can tell a lot about a star — how massive, hot, luminous, old, rich in heavy elements, etc. — just by looking at its light in the proper way. In addition, many other forms of matter, such as planets, stellar corpses, failed stars, gas, dust, plasma, and even black holes have all been identified.
We’re well on our way to performing a “cosmic census” of sorts, where we can add up all the matter and energy of the Universe and what’s composing it. In addition to matter, we’ve identified antimatter in small quantities. There are no stars or galaxies out there, within our visible Universe, made of antimatter instead of normal matter, but there are jets of antimatter streaming away from high-energy natural engines like black holes and neutron stars. There are also neutrinos speeding through the Universe, tiny in mass but enormous in number, generated during the hot Big Bang and also from nuclear processes in stars and stellar cataclysms.
The problem, of course, is that when we take all the ingredients we’ve directly measured, apply the equations that govern the Universe to the cosmos as a whole, and try to put everything together, it doesn’t add up. The laws we know and the ingredients we’ve directly discovered, when combined, can’t explain the Universe as we see it. In particular, there are a few observations that seem to be mutually exclusive if we want to examine the null hypothesis: that what we see and what we know is all there is.
You’ve heard about dark matter before, and the reason you’ve likely heard we need it is that, “there isn’t enough normal matter to account for all the effects of gravity that we see.” The most common question that astrophysicists get about it is, “well, what if there’s just more normal matter out there than the types of matter we’re good at detecting? What if ‘dark matter’ is just more normal matter that happens to be dark?”
The problem with that idea is that we know — from observations we already have — how much normal matter in total exists within the visible Universe. The Universe was hotter and denser in the past, and when things were hot and dense enough, only free protons and neutrons could exist. If they attempted to bind together into any combination of heavier nuclei, the Universe was so energetic that they would be immediately blasted apart. The lightest elements that exist:
- hydrogen (1 proton),
- deuterium (1 proton and 1 neutron),
- helium-3 (2 protons and 1 neutron),
- helium-4 (2 protons and 2 neutrons),
- and lithium-7 (3 protons and 4 neutrons)
were all created in the first 3–4 minutes of the Universe, forming only after the Universe cools sufficiently so that they’re not instantaneously destroyed.
What’s remarkable is that, because the laws of physics that govern particles (and nuclear fusion) are so well-understood, we can compute exactly — assuming the Universe was once hotter, denser, and expanded and cooled from that state — what the different ratios of these different light elements ought to be. We’ve even studied the reactions in the lab directly, and things behave precisely as our theory predicts. The only factor we vary is the photon-to-baryon ratio, which tells us how many cosmic photons (particles of light) there are for every proton or neutron (the baryons) in our Universe.
We’ve now measured it all. Satellites like COBE, WMAP, and Planck have measured how many photons there are in the Universe: 411 per cubic centimeter of space. Intervening clouds of gas that appear between us and a distant light source, like a luminous galaxy or quasar, will absorb a fraction of the light as it travels through the Universe, teaching us the abundances of these elements and isotopes directly. When we add it all up, only ~5% of the total energy in the Universe can be normal matter: no more and no less.
There are all sorts of observations, in addition to the ones mentioned here, that we have to account for. A universal law of nature is no good if it only works under certain select conditions; you have to be able to explain a wide variety of cosmic phenomena if you want your proposed cosmology to be taken seriously. You have to explain:
- the cosmic web of structure we see in our Universe and how it formed,
- the sizes, masses, and stability of individual galaxies,
- the speeds of galaxies zipping around inside clusters of galaxies,
- the temperature fluctuations imprinted in the cosmic microwave background radiation: the Big Bang’s leftover glow,
- the gravitational lensing observed around clusters of galaxies, both isolating and ones in the process of colliding,
- and how the expansion rate of the Universe changes over time in the exact fashion we’ve observed it to change.
There are many other observations we can fold into this selection, but these were chosen for a specific reason: in a Universe made only of normal matter, radiation, and neutrinos in their observed amounts, we cannot explain any of these observations. In order to explain the Universe we see, something additional is needed.
In principle, you could imagine that only one new tweak might account for everything. That maybe, if we were clever enough, we could just add one new ingredient or make one modification to our rules that would explain all of these observations together. That was the original idea behind dark matter, by the way, as it was first proposed in the 1930s by Fritz Zwicky. He was the first to measure the speeds of galaxies zipping around inside of galaxy clusters, and found there needed to be something like ~100 times as much mass as the stars could account for. He hypothesized a new ingredient — dark matter — that might account for all of it.
We know that dark matter, from observations and experiments, cannot be made of any of the known particles that exist within the Standard Model of physics. We’ve learned that dark matter could not have been hot, or fast-moving, even early on; it either needs to be quite massive or it needs to have been born without a lot of kinetic energy. We’ve learned it cannot interact through the strong or electromagnetic or weak force in any appreciable manner. And we’ve learned that, if we add this one ingredient of cold dark matter to the Universe, almost all of the observations fall into line.
With dark matter alone, we can explain many of the observations we cannot account for without it. We get a cosmic web; we get star clusters that merge into small galaxies that grow into big galaxies and eventually galaxy clusters; we get fast-moving galaxies within those clusters; we get a separation between hot gas and the effects of gravity when clusters of galaxies collide; we get galaxies that rotate just as quickly on the outside as they do on the inside; we get significant gravitational lensing, consistent with observations; we get temperature fluctuations that agree with the cosmic microwave background and that explain the likelihood of finding a galaxy a particular distance away from any other galaxy.
But we don’t quite get everything. Dark matter is the one extra “thing” we can add — and it turns out to be an ingredient rather than a modification — to solve the greatest number of these problems all at once, but it doesn’t quite give us everything. It doesn’t solve the (bigger) problem of the expansion rate, and it doesn’t explain the (smaller) puzzle of why, despite outmassing normal matter by a 5-to-1 ratio, the Universe is spatially flat. Somehow, a full 2/3 of the Universe’s total energy isn’t accounted for.
Dark energy, of course, is the second additional ingredient we can add to explain the remainder of the observations. It functions as a form of energy inherent to space itself, only becoming important when the Universe has expanded to become dilute and diffuse enough. It makes up the majority of the Universe’s energy today, after being unimportant for the first ~7+ billion years. And it causes distant galaxies to accelerate, rather than decelerate, as they move away from us in the expanding Universe.
There is no single modification that explains all of these observations together. In fact, any other single modification you can make — either by changing the laws or adding a new ingredient — will solve fewer of these problems than dark matter or dark energy will. Most of the competing ideas out there, such as:
- modifying the laws of gravity,
- having dark energy be a dynamical field or entity that evolves with time,
- or inventing some sort of decaying dark matter or early dark energy,
have one (or both) of two fatal flaws. Either they require more than the two new parameters that are added by dark matter and dark energy, or they fail to solve all of the problems that adding dark matter and dark energy solves.
In science, most people make use of Occam’s razor — the notion that given the choice between explanations, the simplest one is usually best — erroneously. It isn’t simpler to modify gravity than it is to add dark matter and dark energy, not if that modification requires two or more added parameters. It isn’t simpler to introduce a type dark energy that’s anything other than a cosmological constant; the latter is the most “vanilla” class of dark energy there is, and it works for everything. Instead, you’d have to do something like concoct an explanation that introduced only one new entity, replacing both dark matter and dark energy together.
As disconcerting as it is, dark matter and dark energy are the simplest explanation. A dark fluid idea itself necessitates multiple free parameters. The new relativistic MOND introduced earlier this year or the old tensor-vector-scalar gravity of Bekenstein not only adds at least as many parameters as dark matter and dark energy, but they still cannot explain galaxy clusters. The problem isn’t that dark matter and dark energy simply have to be right. It’s that all the other ideas are objectively worse. Whatever is truly going on with our Universe, we owe it to ourselves to continue the investigation. It’s the only way we’ll ever know how nature truly works, simple or not.
Starts With A Bang is written by Ethan Siegel, Ph.D., author of Beyond The Galaxy, and Treknology: The Science of Star Trek from Tricorders to Warp Drive.