The future of astronomy: thousands of radio telescopes that can see beyond the stars
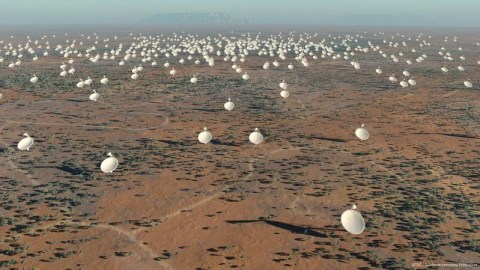
Never heard of SKA, the square kilometer array? Once it starts taking data, you’ll never forget it.
“Not all chemicals are bad. Without chemicals such as hydrogen and oxygen, for example, there would be no way to make water, a vital ingredient in beer.” –Dave Barry
By building bigger telescopes, going to space, and looking from ultraviolet to visible to infrared wavelengths, we can view stars and galaxies as far back as stars and galaxies go. But for millions of years in the Universe, there were no stars, no galaxies, nor anything that emitted visible light. Prior to that, the only light that existed was the leftover glow from the Big Bang, along with the neutral atoms created during the first few hundred thousand years. For those millions of years, there’s simply never been a way to gather information from the electromagnetic part of the spectrum. But a combination of advances in computing and the new construction of an array of thousands of large-scale radio telescopes across twelve countries opens up an incredible possibility like never before: the ability to map the neutral atoms themselves.
How can you see neutral atoms? After all, unless you’re dealing in either reflected light or with atoms that are themselves in an excited state, neutral atoms are some of the most optically boring materials that there are. Atoms are made of negatively charged electrons surrounding a positively charged nucleus, capable of occupying a variety of quantum states. But early on, for millions of years after the Big Bang, 92% of the atoms are the most boring type that exists: hydrogen, with a single proton and electron. While many different energy states exist, without any external source to excite it, hydrogen atoms are doomed to live in the lowest-energy (ground) state.
But when you first make neutral hydrogen, not all the atoms are perfectly in the ground state. You see, in addition to energy levels, the particles in atoms also have a property called spin: their intrinsic angular momentum. A particle like a proton or an electron can either be spin up (+½) or spin down (-½), and so a hydrogen atom can either have the spins aligned (both up or both down) or anti-aligned (one up and the other down). The anti-aligned combination is slightly lower in energy, but not by much. The transition from an aligned state to an anti-aligned one takes millions of years to occur, and when it does, the atom emits a photon of a very particular wavelength: 21 centimeters.
Every time you undergo a burst of star formation, you ionize hydrogen atoms, meaning that electrons will fall back onto protons eventually, forming a large number of aligned atoms. By looking for this 21-cm signal, we can:
- construct a map of nearby, recent star formation,
- detect absorbing, neutral sources of anti-aligned gas,
- build a 3D map of neutral gas throughout the Universe,
- detect how star clusters and galaxies formed and evolved over time,
- and possibly detect the absorption and emission features of hydrogen gas immediately after, during, and possibly even before the formation of the first stars.
Next year, in 2018, just as the James Webb Space Telescope prepares for launch, construction will begin on the Square Kilometer Array (SKA). SKA will wind up, at completion, being an array of some 4,000 radio telescopes, each approximately 12 meters in diameter, and capable of detecting this 21-cm line back farther than any galaxy we’ve ever seen. While the current galactic record-holder comes from when the Universe was just 400 million years old — 3% of its current age — SKA should be able to get the first 1% of the Universe that even James Webb might not see.
To go beyond the first stars, or to arrive at a cosmic destination where no ultraviolet or visible light can pass through the opaque, intergalactic medium, you need to probe what’s actually there. And in this Universe, the overwhelming majority of what’s there, at least that we can detect, is hydrogen. That’s what we know is out there, and that’s what we’re building SKA with the intention of seeing. It will collect more than ten times the data per second than any array today; it will have more than ten times the data collecting power; and it is expected to map the entire Universe from here all the way back to before the first galaxies. We will learn, in the most powerful way ever, how stars, galaxies, and the gas in the Universe grew up and evolved over time.
According to Simon Ratcliffe, SKA scientist, we know some of what we’re going to find with SKA, but it’s the unknowns that are the most exciting.
“Every time we’ve set out to measure something, we’ve discovered something entirely surprising.”
Radio astronomy has brought us pulsars, quasars, microquasars, and mysterious sources like Cygnus X-1, which turned out to be black holes. The entire Universe is out there, waiting for us to discover it. When SKA is completed, it will shed a light on the Universe beyond stars, galaxies, and even gravitational waves. It will show us the invisible Universe as it truly is. As with anything in astronomy, all we need to do is look with the right tools.
Ethan Siegel is the author of Beyond the Galaxy and Treknology. You can pre-order his third book, currently in development: the Encyclopaedia Cosmologica.