The Future Of Astronomy: The Giant (25 Meter!) Magellan Telescope
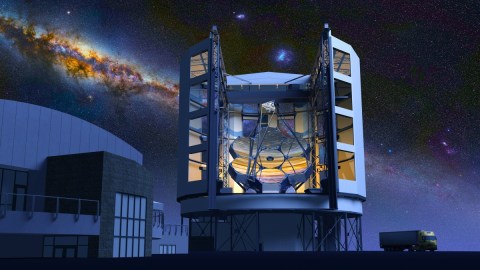
The first of the next generation of telescopes is already under construction. Here’s the audacious new science we’re in for!
“We find them smaller and fainter, in constantly increasing numbers, and we know that we are reaching into space, farther and farther, until, with the faintest nebulae that can be detected with the greatest telescopes, we arrive at the frontier of the known universe.” –Edwin Hubble
Throughout history, there have been four things that have determined just how much information we can glean about the Universe through astronomy:
- The size of your telescope, which determines both how much light you can gather in a given amount of time and also your resolution.
- The quality of your optical systems and cameras/CCDs, which allow you to maximize the amount of light that becomes usable data.
- The “seeing” through the telescope, which can be distorted by the atmosphere but minimized by high altitudes, still air, cloudless nights and adaptive optics technology.
- And your techniques of data analysis, which can ideally make the most of every single photon of light that comes through.
There have been tremendous advances in ground-based astronomy over the past 25 years, but they’ve occurred almost exclusively through improvements in criteria 2 through 4. The largest telescope in the world in 1990 was the Keck 10-meter telescope, and while there are a number of 8-to-10 meter class telescopes today, 10 meters is still the largest class of telescopes in existence.
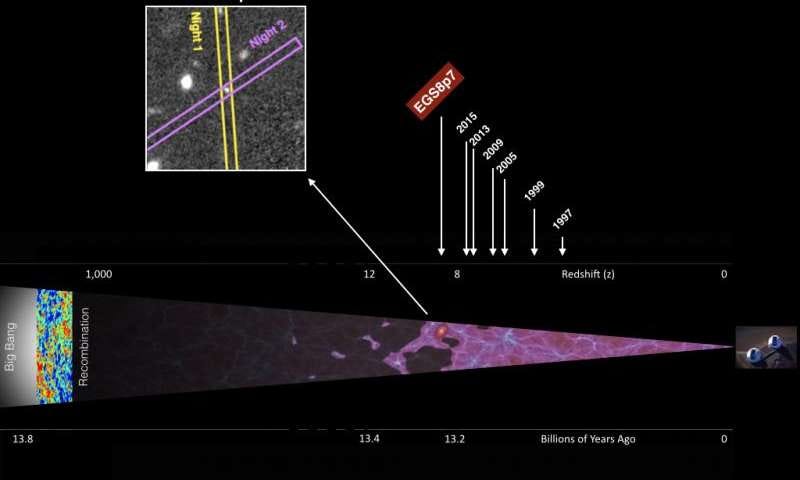
Moreover, we’ve really reached the limits of what improvements in those areas can achieve without going to larger apertures. This isn’t intended to minimize the gains in these other areas; they’ve been tremendous. But it’s important to realize how far we’ve come. The charge-coupled devices (CCDs) that are mounted to telescopes can focus on either wide-field or very narrow areas of the sky, gathering all the photons in a particular band over the entire field-of-view or performing spectroscopy — breaking up the light into its individual wavelengths — for up to hundreds of objects at once. We can cram more megapixels into a given surface area. Quite simply, we’re at the point where practically every photon that comes in through a telescope’s mirror of the right wavelength can be utilized, and where we can observe for longer and longer periods of time to go deeper and deeper into the Universe if we have to.
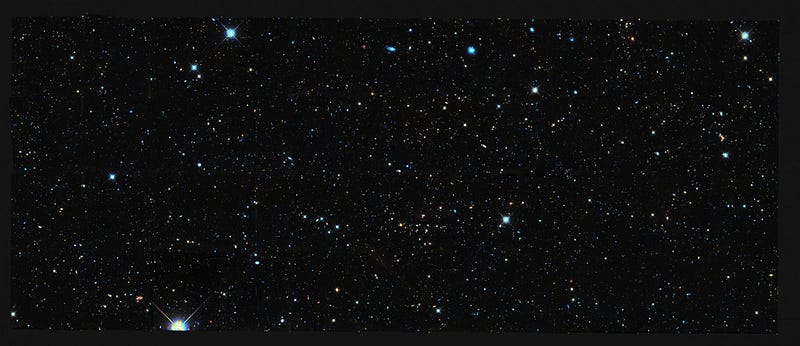
In addition, we’ve come a long way towards overcoming the atmosphere, without the need to launch a telescope into space. By building our observatories at very high altitudes in locations where the air is still — such as atop Mauna Kea or in the Chilean Andes — we can immediately take a large fraction of atmospheric turbulence out of the equation. The addition of adaptive optics, where a known signal (like a bright star, or an artificial star created by a laser that reflects off of the atmosphere’s sodium layer, 60 kilometers up) exists but appears blurry, can allow us to create the right “mirror shape” to de-blur that image, and hence all the other light that comes along with it. This way, we can further eliminate the turbulent effects of the atmosphere.
And finally, computational power and data analysis technique have improved tremendously, where more useful information can be recorded and extracted from the same data that we can take. These are tremendous advances, but just like a generation ago, we’re still using the same size telescopes. If we want to go deeper into the Universe, to higher resolution, and to greater sensitivities, we have to go to larger apertures: we need a bigger telescope. There are currently three major projects that are competing to be first: the Thirty-Meter Telescope atop Mauna Kea, the (39 meter) European Extremely Large Telescope in Chile, and the (25 meter) Giant Magellan Telescope (GMT), also in Chile. These represent the next giant leap forward in ground based astronomy, and the Giant Magellan Telescope is probably going to be first, having broken ground at the end of last year and with early operations planned to begin in just 2021, and becoming fully operational by 2025.
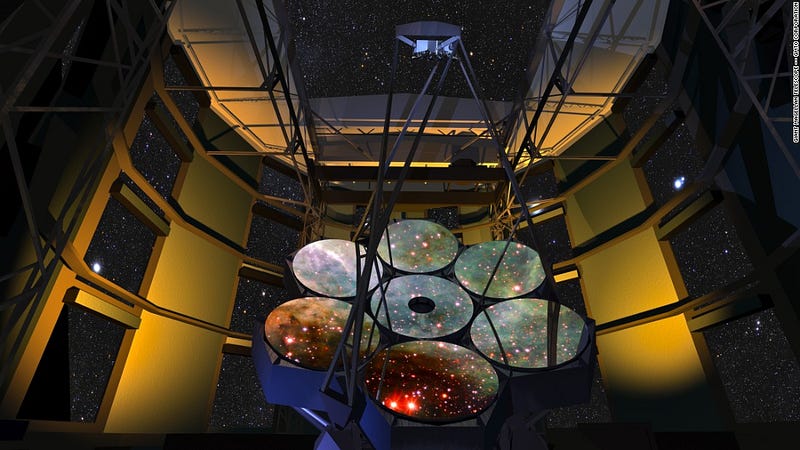
It’s not really technically possible to make a single mirror that large, as the materials themselves will deform at those weights. Some approaches are to use a segmented “honeycomb” shape of mirrors, like the E-ELT plans, with 798 mirrors, but that produces a distinct disadvantage: you get a large number of image artifacts that are difficult to remove where the sharp lines are. Instead, the Giant Magellan Telescope uses just seven mirrors (four are already complete), each a monstrous 8.4 meters (or 28 feet!) in diameter, all mounted together. The circular nature of these mirrors leaves gaps between them, meaning you miss out on a little bit of your light-gathering potential, but the resultant images are much cleaner, easier to work with, and free of those nasty artifacts.
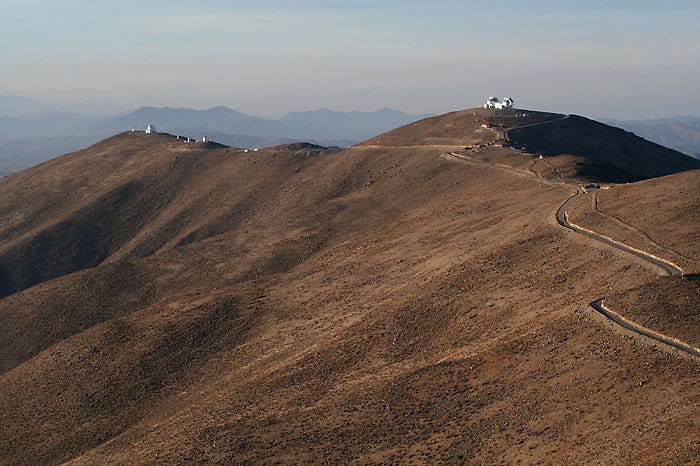
It’s also being built on a great site: the Las Campanas Observatory, which currently houses the twin 6.5-meter Magellan telescopes. At an altitude of nearly 2,400 meters (~8,000 feet), with clear skies and devoid of light pollution, it’s one of the best places for astronomical observing on Earth. Equipped with the same cutting edge cameras/CCD, spectrograph, adaptive optics, tracking and computerized technology that the world’s best telescopes have today — only scaled up for a 25 meter telescope — the GMT is going to revolutionize astronomy in a number of tremendous ways.
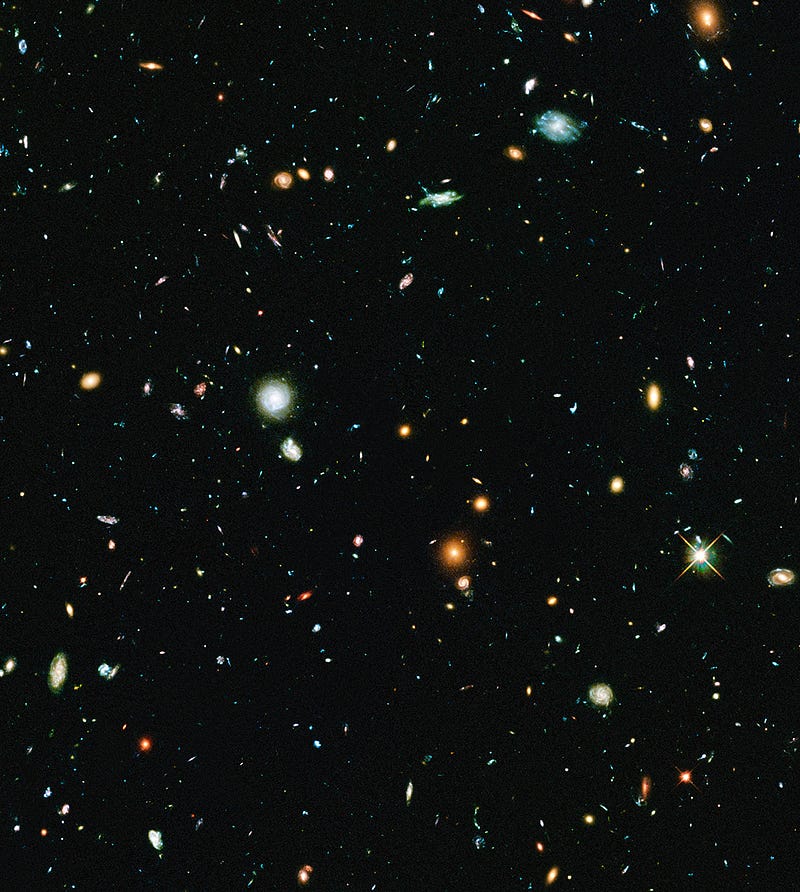
1.) The first galaxies: in order to go deeper into the Universe, you need to not only compensate for the fact that objects that are twice as far away deliver only one quarter of the light to your eyes, but that the expanding Universe causes that light to redshift, or to get stretched to longer wavelengths. Our atmosphere might only let a few select “windows” of light through, but this actually helps us out in some ways: the ultraviolet radiation that gets blocked by our atmosphere from nearby stars like the Sun can get redshifted all the way into the visible (and even near-infrared) portion of the spectrum at great enough distances. Finding these galaxies is easiest from space, but confirming them requires follow-up spectroscopy, which is best done from the ground. Ideally, the combination of the James Webb Space Telescope (last week’s “future of astronomy” article) and the GMT — which can measure the redshift and spectral features of these objects directly and unambiguously — will push the limits of the most distant known galaxies in the Universe out farther than ever, and give us an unprecedented view of how galaxies form and evolve.
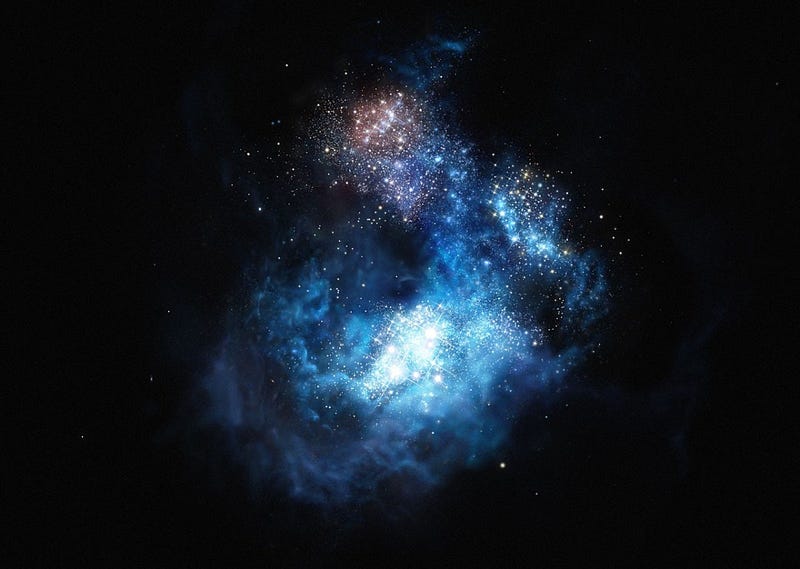
2.) The first stars: even more exciting is the chance to directly observe and ascertain the properties of the first stars ever to form in the Universe. After the Big Bang, when the Universe forms neutral atoms for the first time, there are no heavy elements at all. There’s hydrogen, deuterium, helium-3 and helium-4, and a little bit of lithium-7. That’s it. Absolutely nothing else. And so the first stars that formed in the Universe must have been made out of these materials alone, with none of the heavier elements found in 100% of our Milky Way’s stars. To find these pristine stars — these Population III stars — we have to go to incredibly high redshifts. Whereas today, we’ve barely uncovered one such candidate for these stars, the GMT should be able to discover hundreds of such candidates. In addition, it won’t just discover more, but:
- it should be able to determine the relative elemental abundances within,
- could measure the hydrogen, helium, and possibly even deuterium and lithium concentrations,
- could measure the absorption spectra of the gas clouds between us and them,
- and can discover them before the Universe has been reionized, back when there’s still neutral gas there.
This applies to the first galaxies as well, but is even more exciting for the first stars, enabling us to see pristine samples of the Universe and understand just how big these earliest stars can get.
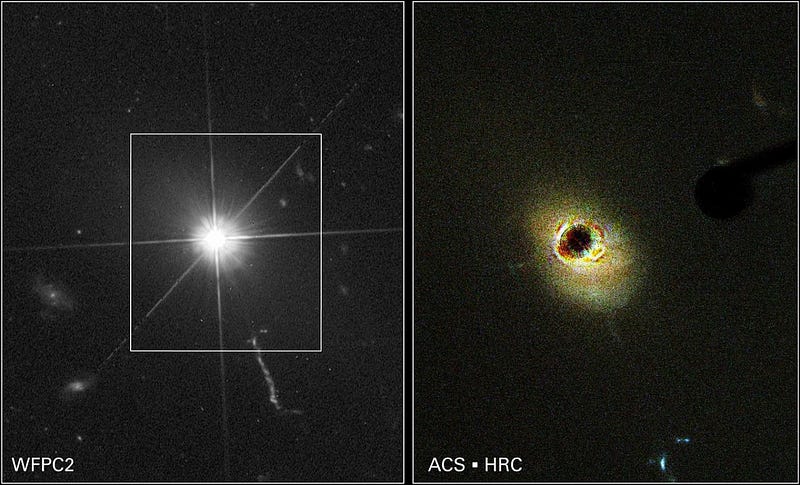
3.) The earliest supermassive black holes: we’ve serendipitously found a large number of these already, in the form of quasars. The largest number of these have been found by large-volume and all-sky surveys like SDSS and 2dF before it, but in order to truly measure these objects well, we need to obtain their spectra, something GMT will be perfect for. The difference between spectroscopy and photometry is a little bit like the difference between a black-and-white TV and a color TV: they can both show you a picture, but with spectroscopy, the level of detail and the amount of information you get increases more than a thousand-fold, as we can learn what’s inside (and how much) via spectroscopy, while without it we can only make assumptions. GMT will not only give us follow-up spectroscopy on what the future EUCLID and WFIRST missions will find — the most distant quasars over huge regions of the sky — but will enable us to find more distant quasars (and hence younger, smaller and earlier supermassive black holes) than anything else in (and out of) this world.
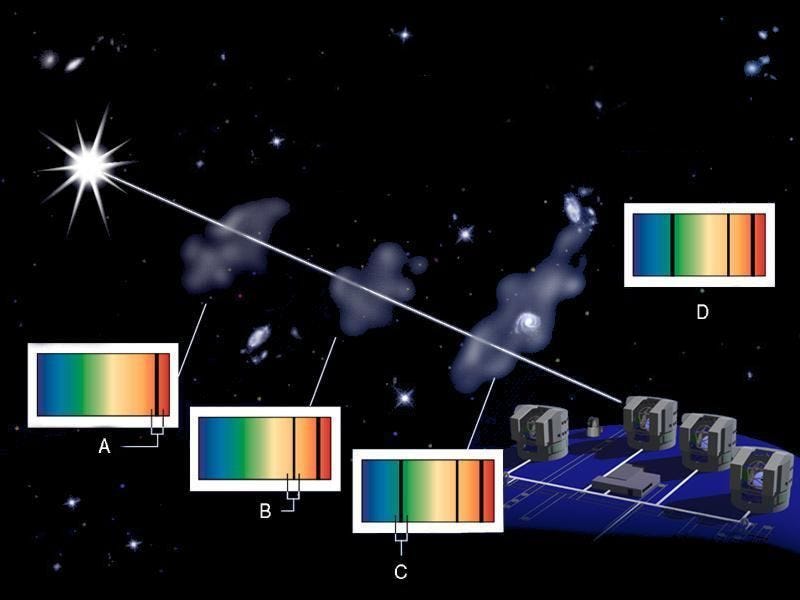
4.) The Lyman-alpha forest: when we look at the most distant quasars and galaxies, we not only see that distant light, but we see every intervening gas cloud there is between that object and ourselves, along the line-of-sight. By measuring the absorption features along the way, we can see how the structure and composition of the Universe evolves, which tells us all sorts of things about components of the Universe that would otherwise be invisible, like neutrinos and dark matter.
Of course, there’s all the “normal” astronomy we can do with it as well, including planet-finding, understanding stellar and galaxy evolution, measuring supernovae and their remnants, planetary nebulae and star forming regions, clusters, interstellar and intergalactic gas and so much more. Perhaps most exciting will be the advances that we don’t know are coming. No one could’ve predicted that Edwin Hubble would discover the expanding Universe when the 100-inch Hooker telescope was first commissioned; no one could’ve predicted how the Hubble Deep Field would open up the Universe when that image was first taken. What will GMT find in the ultra-distant Universe?
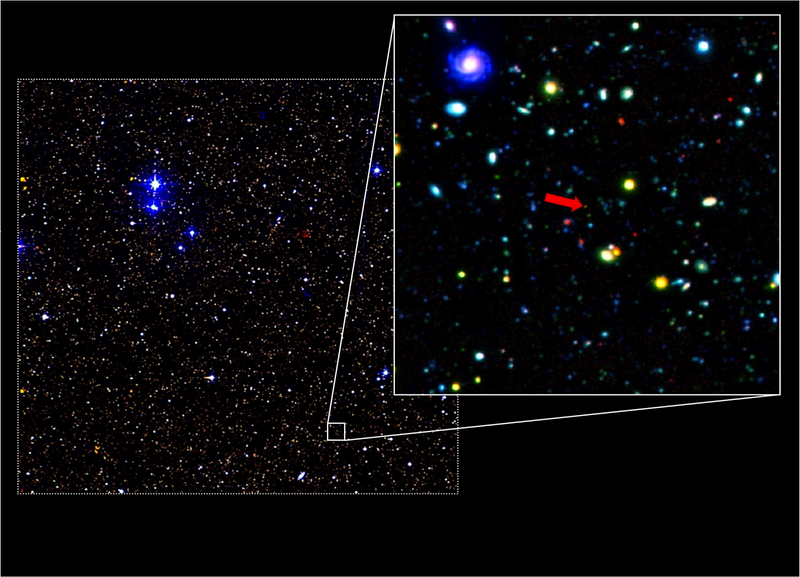
This is why we look, and this is what science at the frontiers is. The Giant Magellan Telescope will do all the things from the ground that space-based telescopes can’t do as well, and will do them better than any other telescope in existence. Unlike the other large ground-based telescopes planned, it’s completely privately funded, there are no political controversies over it, and construction on it has already begun. The future of any scientific endeavor — and perhaps astronomy in particular — requires you to be ambitious, and to invest in looking for the unknown. We’ll never learn what lies beyond our current frontiers of knowledge unless we search, and the GMT is one major step towards looking where no one has ever looked before.
Leave your comments on our forum, and check out our first book: Beyond The Galaxy, available now, as well as our reward-rich Patreon campaign!