The Big Bang by Balloon
How an experiment high above Antarctica — Spider — sheds new light on the cosmic microwave background.
Observations of the cosmic microwave background continue to capture public attention, highlighted these last few weeks in articles about Planck, BICEP, and most recently Spider — a CMB experiment that launched just this month. Like the other two experiments, Spider measured the polarization of the faint glow of the CMB. Unlike the other two experiments, it did so for 2 weeks while circling the Antarctic continent, carried above the ice by a balloon and polar winds.
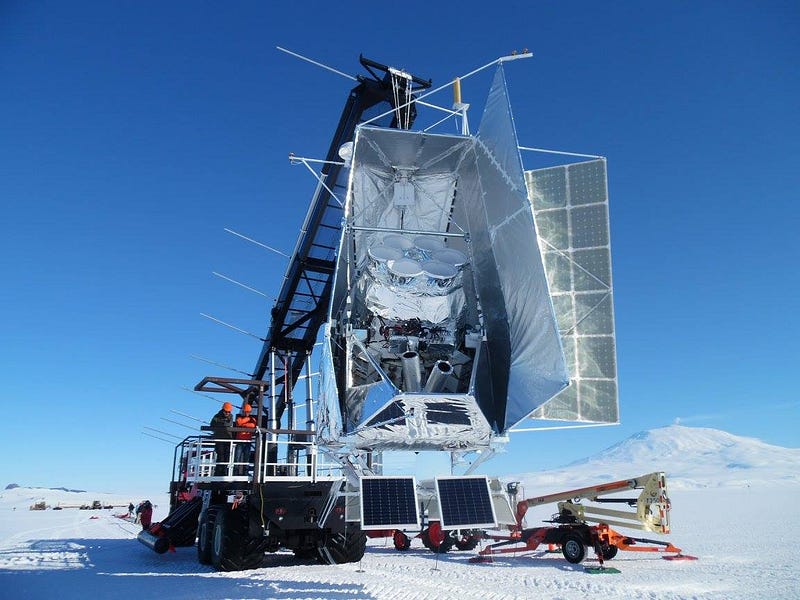
The name “Spider” has an interesting etymology. In the mid-2000s, a group of physicists working on BOOMERanG, another balloon-borne CMB experiment, got together to talk about a next-generation telescope that would measure polarization. The first, napkin-based drawings of the experiment included eight telescopes pointing out in several directions from a central cryostat which kept the telescope cooled to near absolute-zero temperatures. Another novel design idea came up — what if the telescope could drop down on a rope away from the balloon? The balloons needed to carry CMB telescopes to sub-orbital heights are often the size of football stadiums when fully inflated and in the air. By lowering down on a rope and keeping the balloon at a farther distance from the optics hoping to measure the CMB, they’d be able to keep the balloon as far away from the telescope’s field of view as possible. This gave rise to the name: eight telescopes + lowering on a rope = “Spider”. Incidentally, neither of these design features made it into the final experimental setup due to engineering challenges.
Why a balloon?
In the realm of CMB telescopes there are three categories: ground-based, balloon-borne, and satellite. Planck, the ESA satellite that collected temperature and polarization data from 2009–2013, is a satellite mission. BICEP, making news recently for it’s observations of B-mode polarization, is a ground-based telescope in Antarctica. Each of these types of designs have pros and cons. Satellites give us unmatched sensitivity, since we don’t have to worry about the faint signal from 380,000 years after the big band being distorted by the Earth’s atmosphere. Viewing the entirety of the sky, a feature which has allowed us to hone in on peculiarities at the largest scales in the CMB, is only achieved through satellite observations. Their main con is cost; Planck was estimated to cost a little under $1 billion USD. Further, there are no means of providing upgrades or repairs to the hardware after it has been sent into orbit.
Ground-based telescopes like BICEP, the Keck array, and the South Pole Telescope (SPT) do have to worry about atmospheric absorption and distortion of the incoming microwave light that they hope to measure, but they are much more cost effective. As new technology is developed, the previous telescope can be updated with more sensitive optics and electronics. SPT is currently undergoing its third upgrade to SPT-3G, where over the same viewing angle as the previous iteration they will have ten times greater sensitivity with their CMB temperature measurements. But, since they’re locked into the ground below, their field of view is only around 5% of the whole sky. That’s totally fine for some CMB science-goals like galaxy cluster finding, but not so much if you are interested in how the CMB behaves at large angular separations on the sky.
Balloon-borne telescopes offer an intermediate step between these two types. The balloons are considered suborbital when they collect data; for Spider, this was at 36 kilometers (22 miles) above the ground. (For comparison, the elevation at the South Pole, while significant, is only 2.7 kilometers, or about 1.7 miles.) At that altitude, balloon-borne telescopes are able to view the sky without worrying about significant interference from the atmosphere. This gives them an additional benefit — they make excellent testing grounds for technology that ends up in orbital, satellite telescopes. And, because the development and construction for a balloon payload is a much faster process than the approval protocol satellites must go through, they are able to use the latest technology in their design.
Compared to ground-based telescopes, balloon telescopes are able to see a wider patch of the sky, observing anywhere from a few percent to fifty percent depending on the particular experiment. The development costs of balloon experiments are in the millions of dollars, hundreds of millions of dollars cheaper than their satellite counterparts. Eventually though, the helium-filled balloon deflates and the telescope comes crashing back down to the ice; these telescopes are single use and their data collection time is mere weeks, compared to years for satellites.
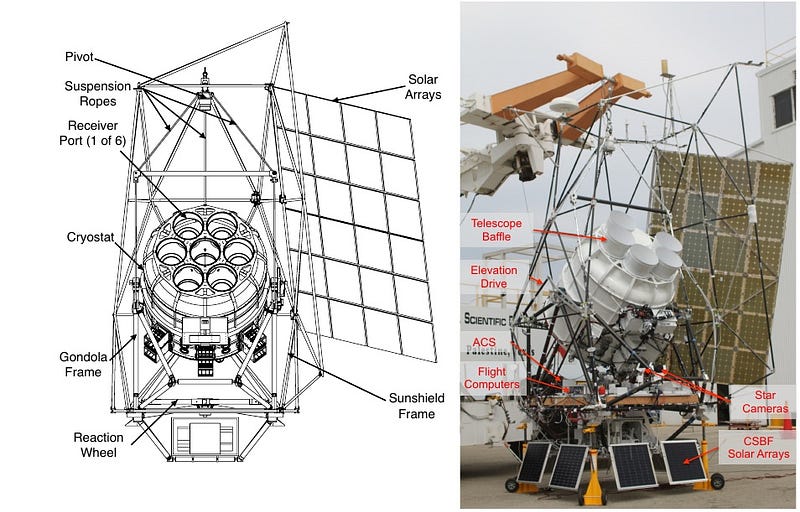
There are a few engineering challenges that are specific to balloon telescopes, however, such as how to know where your telescope is looking and making sure you are pointing at all available patches of sky. It turns out these massive telescopes hanging 20 miles in the air by a string are steerable. The telescope can move left and right very precisely by using the same physics that’s demonstrated in every 8th grade science classroom — conservation of angular momentum. A large, heavy reaction wheel attached to the bottom of the payload spins in one direction, and to conserve angular momentum the telescope turns in the opposite direction. This is exactly the same physics that makes a person sitting in a desk chair spin when they hold a rotating bike wheel.
In Spider’s case, the telescope is so heavy that it also needs help steering from a pivot at the top of the experiment, too. While in flight Spider used the Sun and magnetometers to tell where they were looking and which way they needed to turn (this was actually their backup plan; originally Spider would have used GPS to know where it was pointing, but that piece of equipment stopped working immediately after launch).
Despite challenges before and after launch, Spider’s flight was a success. The data and science results won’t be available for some time. First, the stream of in-flight data that has been collected must be converted into maps of the CMB fluctuations. To make sure their maps correspond to the right directions on the sky, they use known star locations as a reference that are tracked using cameras that have arcsecond (1/3600th of a degree) precision. Then, analysis of the maps can begin, and sometime after Spider will be able to tell us their version of the CMB polarization story.
Life in Antarctica
I’m lucky enough to have a group of grad students, postdocs, and faculty working on CMB experiments, including Spider, just down the stairs from my office. As a theorist working on CMB projects myself, with the ultimate goal of providing predictions for future experimental observations, being able to knock on someone’s door and ask what exactly is a reasonable expectation for upcoming data has been an invaluable resource. But as soon as anyone comes back from the ice, the first thing I ask is “what’s it like to live in Antarctica?” My colleagues are as patient with this as they are with my science questions, and indulge me with a few stories and insights that I’m sure they’ve told countless times before.
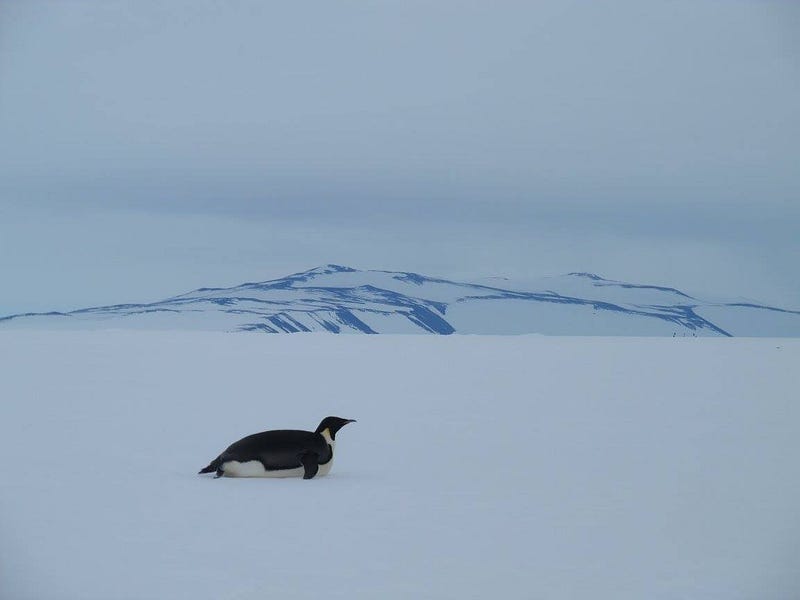
The scientists themselves (about 15 from Spider at any given time) live at McMurdo Station, a large base run by a branch of the NSF that oversees Antarctic science for the United States. To get to the balloon station 6 miles (10 km) away, a bus picked everyone up and made the 45 minute trek. Actual workdays ranged from an average 9–5 to 12 hour “night” shifts that actually happened in daylight — this time of year there is 24 hour sunshine at the south pole. During Spider’s 2 weeks of flight, some of the scientists spent time on “gondola siderial shift,” meaning the hours they worked were completely dictated by the longitudinal location of the telescope.

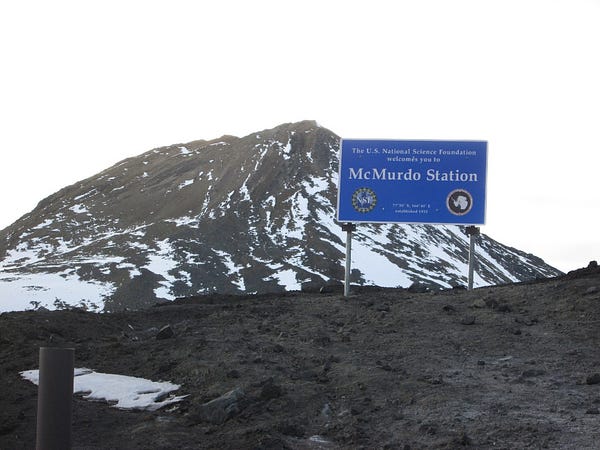
Life at McMurdo is often recounted to me as being somewhat ‘normal.’ As one person put it, “Living in McMurdo is kind of like being in college again, except that everyone actually wakes up for breakfast.” People are housed with roommates in dorms and have shared bathrooms, and food for every meal is served buffet style. Outside of your dorm, you can: get a beer at one of two bars (one of them being distinguished as “the dive bar”); go on hikes to points with excellent views; visit some historic sites from early Antarctic expeditions; watch movies in a dedicated movie room; hit up the gym; or get a cup of coffee at the coffee shop. For people at the station over holidays, there are always things like an official Thanksgiving dinner. And from what I understand, one of the most typical things to do on your way to the base during the layover at Christchurch, NZ, is to stop by a particular liquor shop to pick up some Scotch.
Although, the terrain was described as anything but normal. The same colleague had this to say:
I have the same 40 minute to and from lab every day, but it looks different every single time. The light changes, the snow drifts, the wind transforms the environment, and the pace of change is not so glacial. In Antarctica more than any other place I’ve been it feels as though you really can’t see the same view twice. It’s a new and different experience every time, and it makes me want to see more.
It makes me wonder, do they ever let theorists go to the ice?
This post was written by Amanda Yoho, graduate student in cosmology at Case Western Reserve University, specializing in primordial fluctuations and their signatures in the Cosmic Microwave Background. She extends her thanks to Sean Bryan, Natalie Gandilo, Jamil Shariff, and past/current members of Ruhl lab.
Leave your comments at the Starts With A Bang forum on Scienceblogs!