Sparks fly when you microwave grapes: here’s the science of why
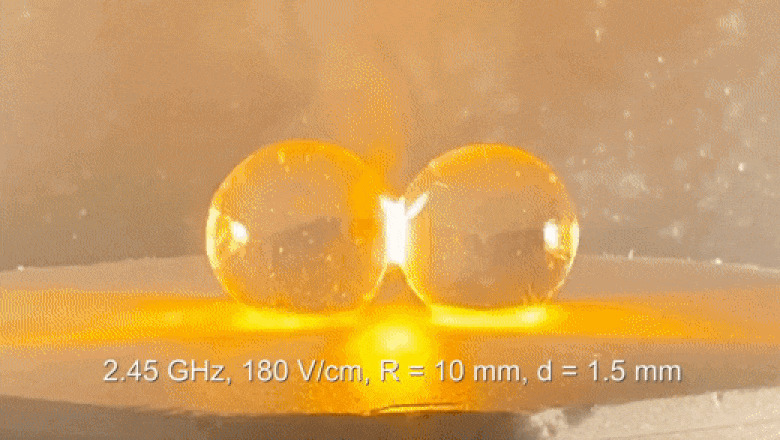
- When you put two grape hemispheres close together in a microwave oven, they put on a spectacular light show.
- The microwaves create a plasma, but the complex physics of why this occurs has been a point of contention among theorists.
- At last, a high-precision experiment has pinned down why, and it's simply classical electromagnetism at work, not a complicated resonance.
For more than 20 years, microwaving grapes has been a popular trick for creating a plasma — and a spectacular, if messy, show — right in your own home. The trick, as reported all over the internet, is to:
- take a grape
- cut it very neatly in half
- except to leave a thin bridge of “grape skin” connecting the hemispheres
- place it in the microwave (without the rotating tray)
And then sit back and watch the sparks fly!
It was assumed, by many, that the sparks were caused simply by electrical conduction: The microwaves interacted with the grapes, created a difference in the electric potential between the two hemispheres, and when the potential became great enough, current flowed. When that current flowed across the grape skin, it heated it up due to the skin’s electrical resistance, and as a result, electrons were kicked off of their atomic nuclei, creating the plasma effect that’s so prominently visible. There’s only one problem with this explanation: everything. Here’s the science of what actually causes grapes to spark in a microwave, and how we figured it out.
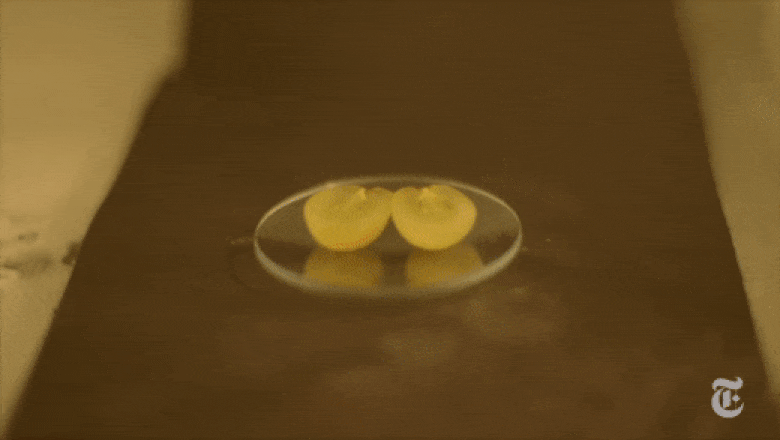
The first thing we’d want to do, whenever we formulate any hypothesis, is to test the premise it rests on. In other words, when we have an idea about how things work, we don’t just put that idea to the test; we go back to the starting point — our assumptions that led us to form our hypothesis in the first place — and make sure that they’re actually a valid place to start.
In this case, the assumption is that the grape needs to be split so that the two hemispheres are almost completely severed, but not quite. There needs to be a thin film, one that’s solid but lacks the electrical conductivity of the aqueous interior of a grape that connects the two hemispheres.
The simplest test we could perform to see if that’s even the case is to take two completely separate grapes and to repeat the experiment. Instead of a single grape cleaved neatly and almost perfectly in half, we’d take two distinct grapes and place them close together: so close that they’re almost, but not quite, touching. If electrical conduction were the mechanism at play, there would be no sparks, no plasma, and no exchange of electric charge.
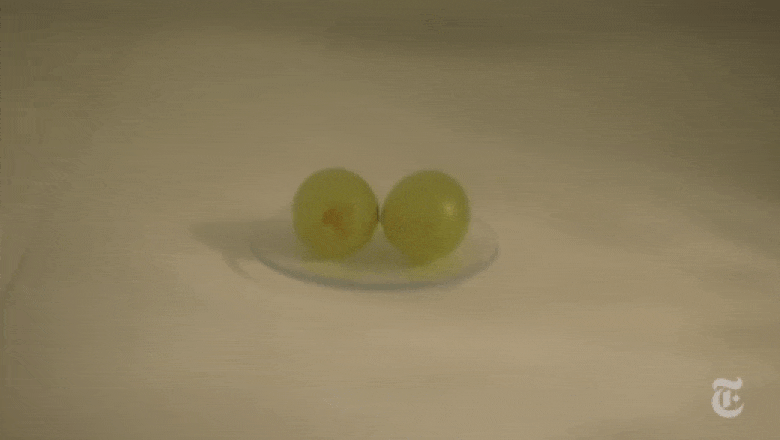
Clearly, when we perform this experiment, we can see the flaw in our assumption that electrical conduction is the mechanism behind the sparking between two grapes. We can also see that grape skin is not an essential part of this process, that a physical connection between the two “sides” of the experiment are not necessary, and that some other mechanism must play a role in order to explain what we observe.
In 2019, a team of three scientists — Hamza Khattak, Pablo Bianucci, and Aaron Slepkov — put forth a paper that asserted resonance was to blame. The grapes themselves behave as resonant cavities, and even though the microwaves themselves have a wavelength that’s about 10 times the physical size of a grape, the electromagnetic fields generated by those microwaves become concentrated within the grapes themselves. The authors then surmised that this resonance winds up creating “hotspots” on the grapes themselves, in particular at the junction between two grapes.
By combining thermal imaging with computer simulations, they believed they had finally explained this longstanding household puzzle.
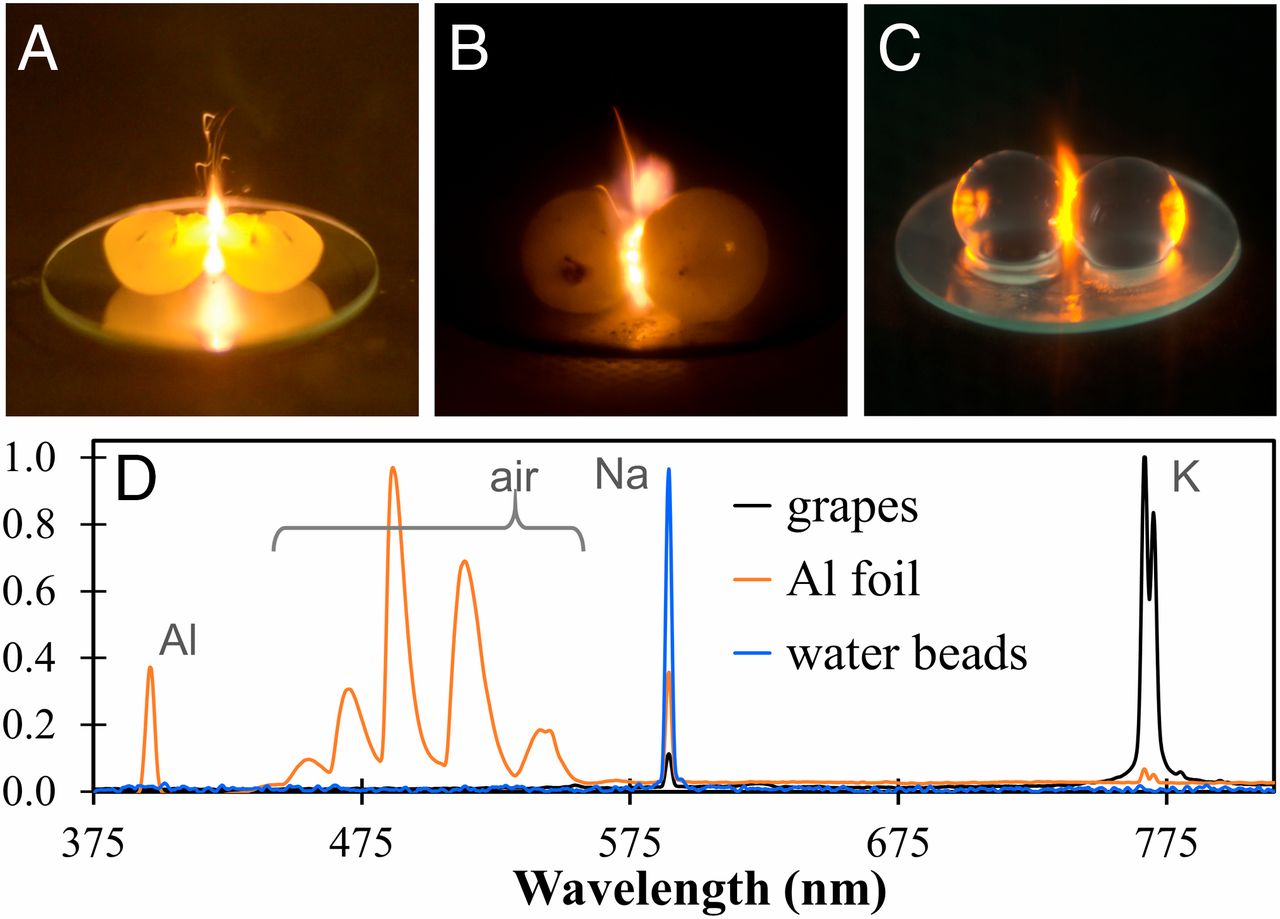
The key to their conclusions came from the thermal imaging studies. Whether using two grapes or a pair of grape-sized hydrogels, they turned a heat-measuring infrared camera onto these objects while they were being microwaved. If the microwaves were heating the internal material evenly, you’d expect the temperature to rise equally across the grapes and/or hydrogels. Only if there were some sort of uneven heating occurring — where the objects developed one or more “hotspots” on them — would you resort to a more complicated explanation.
But that latter situation, where hotspots developed, was precisely what the researchers observed. In particular, they saw that the hotspots didn’t just develop anywhere, but at the junction between the two objects. Whether they used two hemispheres connected by a thin bridge, two skin-off grapes, or two hydrogel spheres, the same phenomenon ensued: the heating occurs primarily in the location where these two objects interface with one another.
What was really exciting and unexpected, however, was what occurred where the two surfaces touched: it compressed the wavelength of the microwaves by a factor of ~80 or so, an unprecedented enhancement.
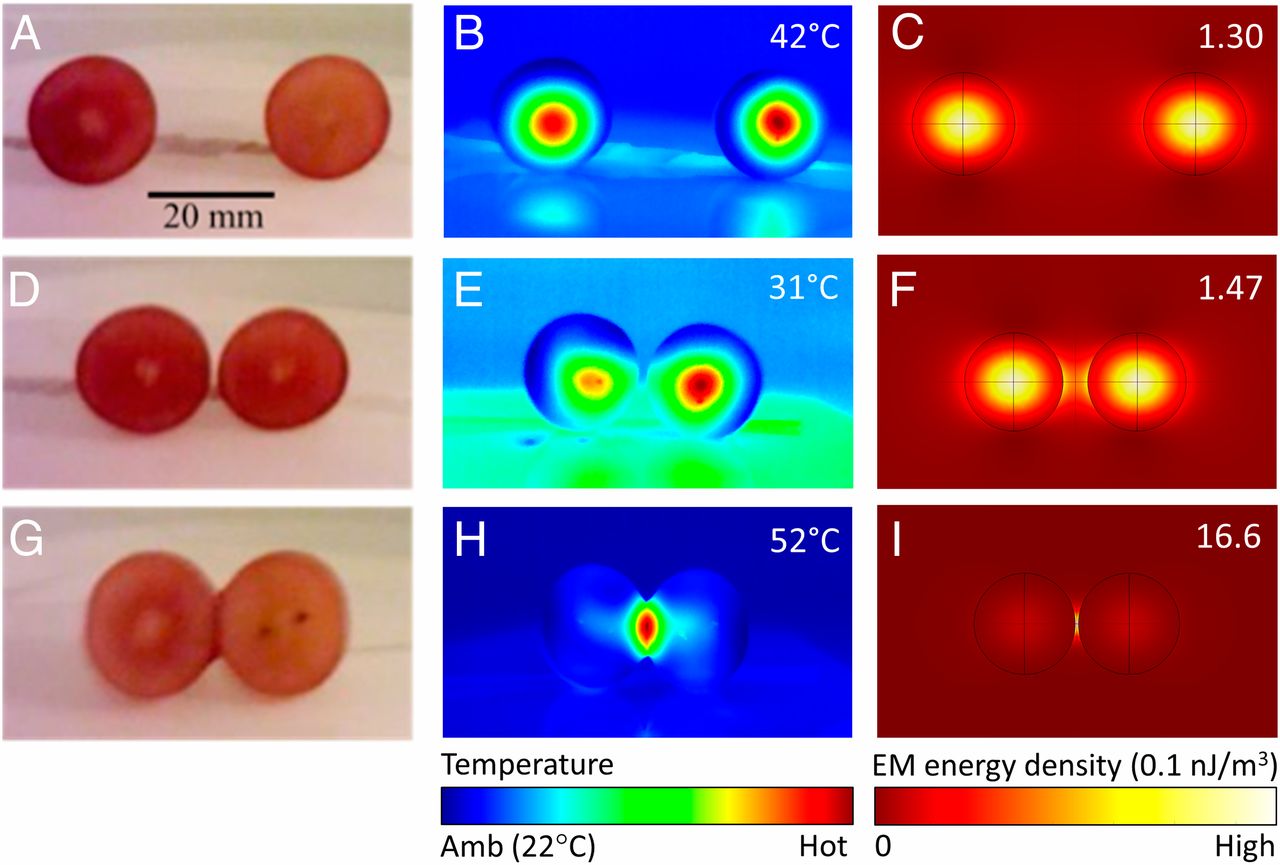
By putting thermal paper in the thin air gap between those two grapes, they were able to see what sort of “etching” was being deposited on this paper. In theory, the resolution of that etching should be limited by what we call the diffraction limit of electromagnetic waves: half the size of the full wavelength. For the microwaves found in your microwave oven, that would correspond to about 6.4 centimeters (2.5 inches) in length: significantly larger than even the grape itself.
Sure, light changes its wavelength when you pass it through a medium, and a medium like water, a hydrogel, or the interior of a grape will also possess different dielectric properties than air or a vacuum. But somehow, the etchings were only ~1.5 millimeters (0.06 inches) in size. Because of that observation, the authors concluded that the microwaves were being compressed by a factor of more than ~40 at the interface between the two objects.
If true, it would have profound implications for photonics: enabling researchers to use light to achieve resolutions that exceed the diffraction limit, something that’s long been thought impossible.
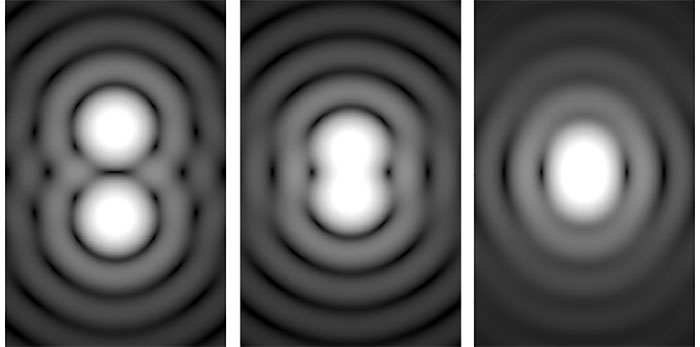
But is that correct? It’s one thing to propose a theory that successfully explains what you see in one circumstance. Although when that explanation then results in a prediction that’s thought to be impossible, you can’t simply accept it at face value. It’s absolutely vital to perform that critical test yourself and see if what’s predicted is what occurs.
Alternatively, however, you can put the underlying assumptions to the test, which is precisely what the research team of M. S. Lin and their collaborators did in October of 2021 in the Open Access journal Physics of Plasmas.
Instead of a buildup of hotspots owing to resonance, the team hypothesized an alternative mechanism: a buildup of the electric field in the small gap between the two liquid spheres, such as grapes or hydrogels. They visualize the two spheres as electric dipoles, where equal and opposite electric charges build up on the two sides of the spheres. This polarization results in a large electric potential in the gap between the spheres, and when it gets large enough, a spark simply jumps the gap: a purely electrical phenomenon. In fact, if you’ve ever turned the crank on a Wimshurst machine, exactly the same phenomenon causes the sparks there: exceeding the breakdown voltage of the air separating the two spheres.
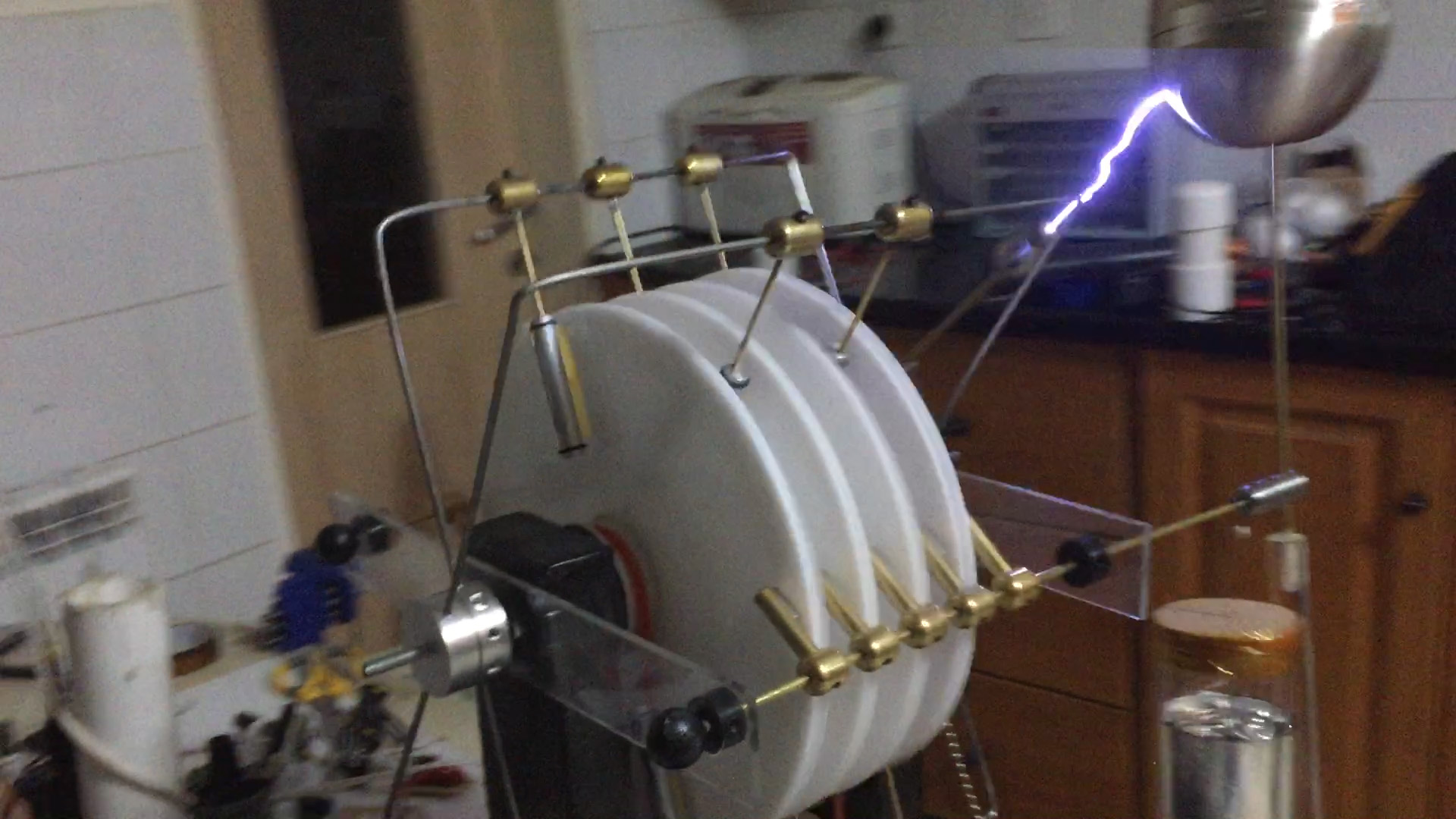
This is interesting, because a buildup of electric charge and an exchange of electrical energy through a discharge can also cause rapid and localized heating. In other words, the explanation proposed by the earlier study, of an electromagnetic hotspot, isn’t the only game in town. Instead, an electrical hotspot could just as easily be the culprit. In this newer explanation, there’s the additional benefit that no defiance of the diffraction limit needs to be hypothesized. If the sparking is electrical in nature rather than electromagnetic — meaning that it’s based on the transfer of electrons rather than the resonant buildup of light — then the entire experiment has nothing to do with the diffraction limit at all.
The key, of course, is to figure out what critical test to perform to determine which of these two explanations best accounts for the phenomenon we’re investigating. Fortunately, there’s a very simple test we can perform. If there are electromagnetic hotspots forming on the surfaces of the two spheres, it will generate increased radiation pressure between them, causing them to repel. However, if these are electrical hotspots produced by the buildup of opposite charges on either sphere across the gap, there will be an attractive electrical force instead.
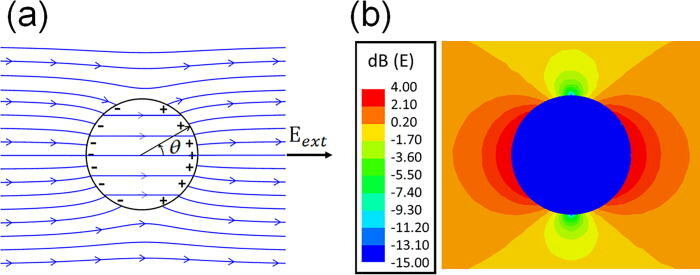
It seems pretty simple, then, right? All we have to do, if we want to rule one of these two possible explanations out, is to have those two spheres begin a very small distance apart and then apply the microwaves.
- If the electrical hotspot explanation is correct, then that means an electric field is causing both spheres to polarize. If the spheres are lined up along the direction of the electric field, there will be a large voltage generated between them, followed by the two spheres moving closer together, followed by sparks and a plasma breakdown. If the spheres are lined up perpendicular to the electric field, however, there should be no net effect.
- If the electromagnetic hotspot explanation is correct, then that means there will be changing electromagnetic fields inside and outside the water droplet, and the two droplets should develop hotspots, repel, and spark regardless of how they’re oriented within the microwave.
This is what we ideally want: a way to tell the two scenarios apart. All we need to do, if we want to invalidate (at least) one of them, is to do the experiments ourselves.
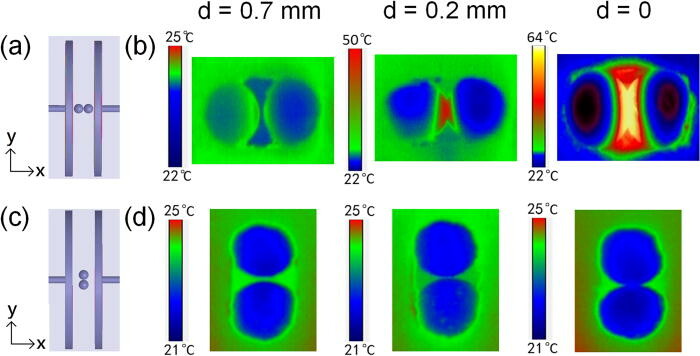
The first experiment that was performed was a simple proof-of-concept of the electrical hotspot idea. Instead of using a microwave cavity, the researchers started with a parallel plate capacitor: an electrical setup where one side is loaded with positive charges and the opposite side is loaded with an equal amount of negative charges. They lined up the two spheres inside the capacitor in two different configurations, one where the spheres were parallel to the field and one where they were perpendicular.
Just as you’d anticipate, the spheres lined up in the direction of the electric field polarized, attracted, and swiftly heated up, while the ones lined up perpendicular to the electric field neither moved nor heated up at all. The next step was the most critical: to subject the two spheres to microwave radiation and to measure, with high-speed photography and to great precision, whether their initial motion would be toward or away from one another. If it’s attractive, that supports the electrical hotspot idea, while if it’s repulsive, it would instead support the electromagnetic hotspot idea.
As the above video clearly demonstrates, these two grape-sized spheres, driven by microwave radiation and an electric potential, initially separated by just 1.5 millimeters (about 0.06 inches), become attracted to one another, and move so that they practically touch. Upon (or just prior to) contact, energy is released, which eventually leads to the formation of a plasma, ionization, and a visually stunning display.
However, as spectacular as the release of energy and the ensuing plasma display is, that’s not the scientifically interesting part; the key point here is that the two spheres attracted one another. In fact, the researchers were further able to rule out the electromagnetic hotspot explanation by changing the frequency of the microwaves over a factor of ~100 or so: if it was a resonance, as the earlier study had speculated, sparks would only appear for one particular set of wavelengths. But what was experimentally seen were sparks present over all frequency ranges.
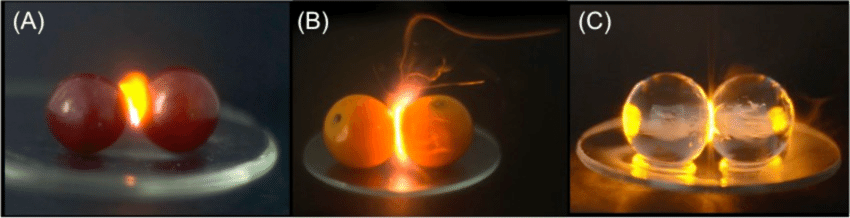
Even though electromagnetic resonances may be present, they are not the driving factor behind the creation of sparks and plasmas. An electrical discharge from air arcing is what’s responsible. Furthermore, by testing this at both low frequencies (27 MHz) and high frequencies (2450 MHz), and seeing approximately equal attractive motions, the researchers were able to demonstrate that the electromagnetic hotspot idea, which should be maximized in the latter case, could not generate even the slightest observable repulsive force.
It’s still great fun, even if a bit unsafe, to microwave two grapes a very small distance apart, and watch the sparks fly. You are, in fact, generating a plasma in your microwave, as electrons are being ionized from the atoms and molecules present at the interface of these two spheres.
But why is that happening? What’s causing this fantastic reaction?
An earlier idea, that electromagnetic hotspots are forming within these spheres as they act like resonant cavities, has now been experimentally disfavored. Instead, it’s simply an electrical discharge occurring between two heavily charged-up surfaces due to their polarization. As is so often the case, scientific investigation uncovers different aspects of a particular problem one at a time. Through the process of responsible inquiry, we slowly assemble a better picture of the reality we all inhabit.