Sorry Science Fans, Discovering A 70-Solar-Mass Black Hole Is Routine, Not Impossible
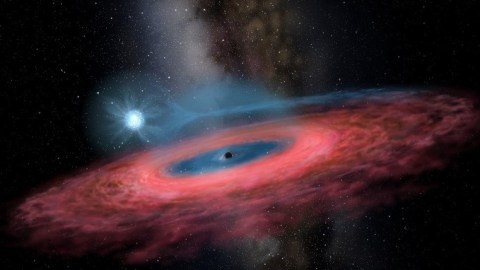
If this discovery came in a pre-LIGO era, maybe it would have been a surprise. But in 2019, there’s no mystery at all.
Did you hear that astronomers had recently discovered a stellar mass black hole that was so heavy, it shouldn’t exist? At 70 solar masses and closer to the galactic center than we are, it’s certainly an interesting system to discover, entirely worthy of its publication in Nature last week. (Full, free preprint available here.) It ranks, at the moment, as the heaviest stellar mass (as opposed to supermassive) black hole ever discovered through optical techniques.
But on the theory side, claiming that this object shouldn’t exist is not only foolish, it requires that you ignore a number of basic facts about astronomy and the Universe. We’ve already discovered a handful of comparably massed black holes through gravitational waves, and have a very good idea of how they form and why. Here’s the science of these heavy black holes that goes beyond the superficial.
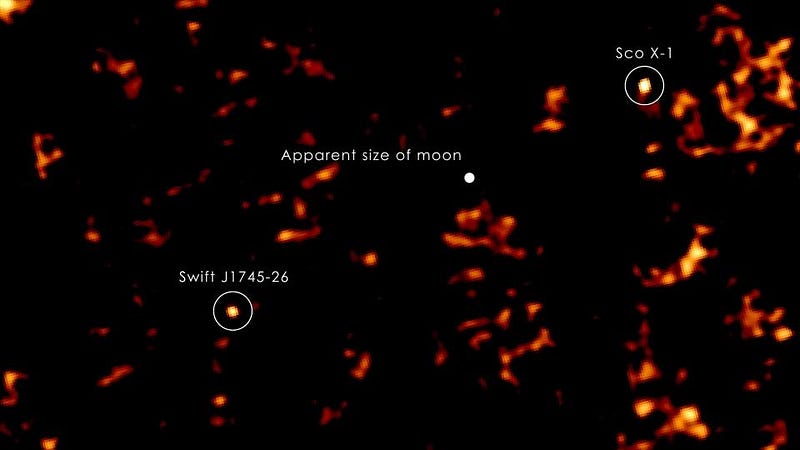
When it comes to detecting black holes in general, there are three ways to do it.
- You can find a black hole that’s actively gobbling up matter and measure the (X-ray and/or radio) radiation that it emits, inferring the black hole’s mass from the light we measure.
- You can find a light-emitting object (like a star or pulsar) orbiting a black hole, measure its orbit over time, and deduce what the black hole’s mass must be.
- Or, as of 2015, you can look for gravitational waves arising from the inspiral and merger of two dense, massive objects (like black holes) and, with enough good detectors, determine their pre-merger and post-merger masses, as well as their location in the sky.
All three methods have proven enormously useful, revealing some fascinating information about our Universe.
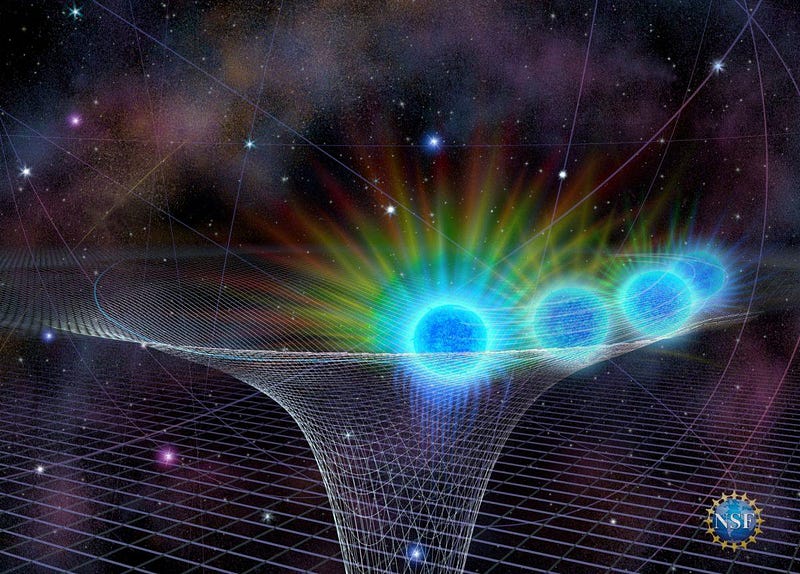
The majority of stellar mass black holes — where the black hole in question is in the same mass range where we find stars (up to about 300 solar masses) — are known to be relatively light: between about 5 and 20 solar masses. However, you cannot just make a black hole as heavy as you want. There are important astrophysical constraints on how massive a black hole will be, and not every possible outcome is physically allowed.
For example, the most common way the Universe has to make a black hole is through a supernova explosion: the death of a massive star. When stars live, the internal radiation pressure that results from nuclear fusion counteracts the gravitational force trying to collapse the star down. When a very massive star runs out of fuel in its core, that collapse is suddenly irresistible, and the core implodes to form a black hole, while a runaway fusion reaction blows the outer layers off.
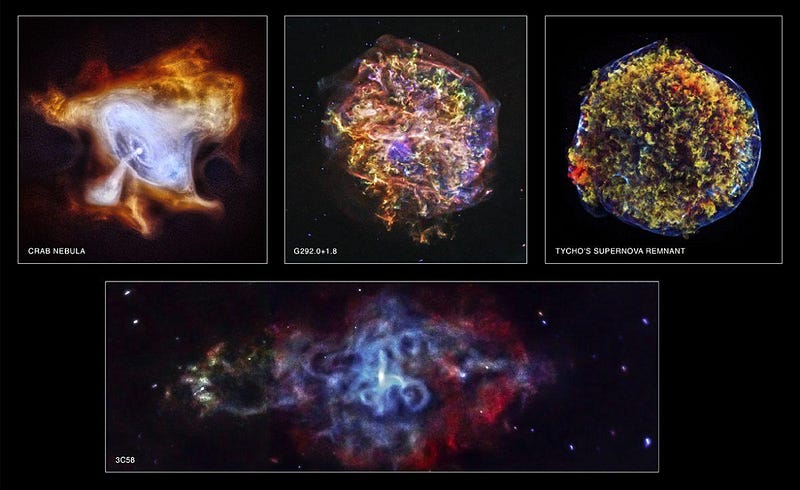
This is where things start getting interesting. The fate of your star isn’t just tied to its mass, although mass is certainly a major factor. In addition, the star’s environment matters, including:
- what elements it’s initially made out of (hydrogen and helium, plus heavier elements like oxygen, carbon, silicon, iron and more),
- whether there’s a companion star capable of either siphoning matter away from the star, giving matter up to the star, or even merging with the star itself,
- and what processes occur with specific efficiencies inside that star.
That first factor alone — what astronomers call a star’s metallicity — can play an enormous role in the ultimate outcome of a star, and the black holes that do (or don’t) result from its demise.
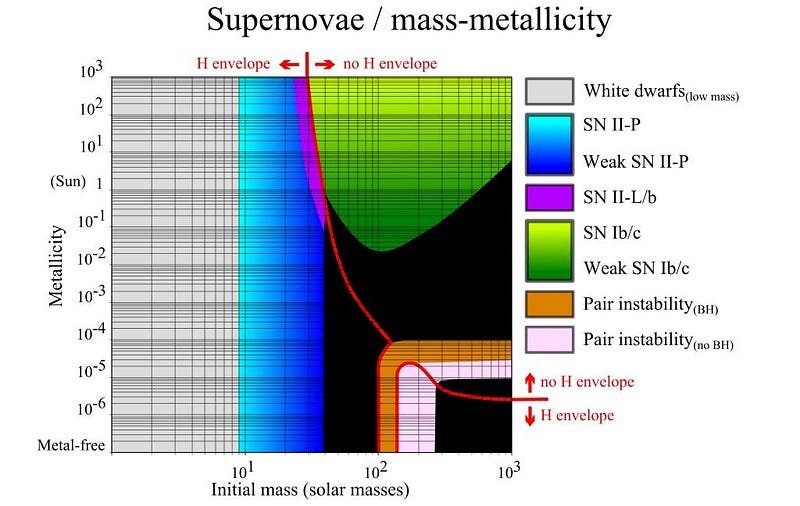
There is a very contentious claim that, beyond a certain mass, the supernovae that occur for an extremely massive star won’t result in a black hole at all. Rather, the idea goes, that either the star’s internal temperature gets so hot that you spontaneously form electron/positron pairs (the lightest matter-antimatter pair that couples to photons) out of the radiation in the star, and you get a pair instability event, that either leads to a black hole immediately or destroys the star entirely.
That’s for low-metallicity stars, in theory. For high-metallicity stars, however, the idea is that the outer portions of the star get blown off: most of the hydrogen and helium. The remaining core might go supernova, but won’t leave you a black hole that goes above about 20 solar masses. That’s the old idea that many have referred to in claiming that this 70 solar mass black hole in a high metallicity environment is impossible.
But we know that idea is untrue.
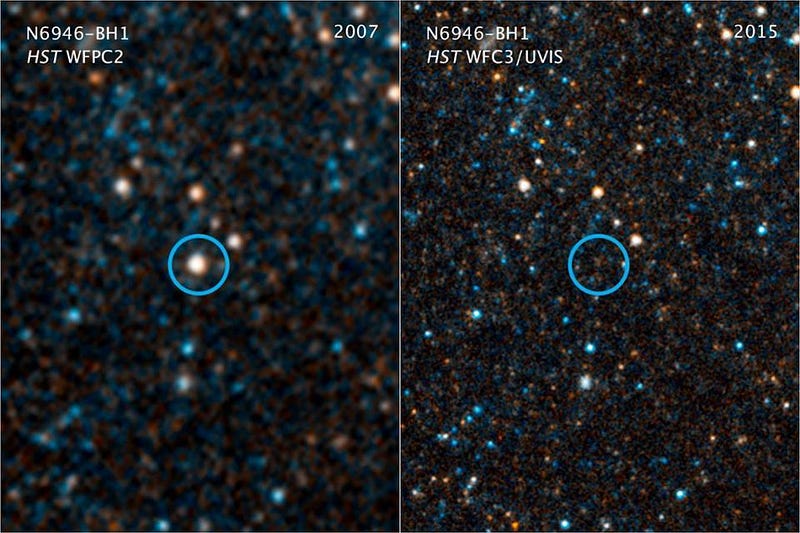
One reason that we know this is untrue is because not all massive stars end their lives in a supernova; a substantial fraction undergo what we call “direct collapse.” Stars can burn their nuclear fuel, chugging along down that path towards a supernova of burning heavier element after heavier element, where the core contracts and heats up as it escalates from burning carbon to oxygen to neon, magnesium, silicon, sulphur and beyond.
But every once in a while, an attempt to step up the ladder will create too dense of an environment too quickly, and a black hole will form, quickly swallowing up the entire star. This was first observed in 2015 by Hubble, where a previously seen star known as N6946-BH1, of around 25 solar masses, spontaneously collapsed into a black hole with no supernova at all. This is real, it happens, and it easily leads to more massive black holes than the previous upper limit.
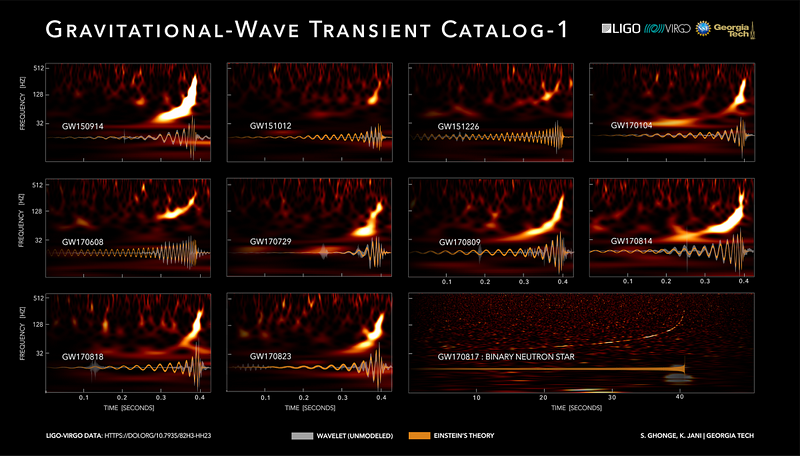
The second reason we know that black holes above 20 solar masses are not only possible but common comes from our direct observations of the Universe with gravitational waves. As black holes orbit other black holes, they radiate energy away in the form of gravitational waves, causing the two masses to inspiral and merge. During the first two science runs of LIGO and Virgo, a total of 11 events were seen, with 10 of them resulting from black hole-black hole mergers.
If we look at the 5 most massive black hole mergers, we’ll find that LIGO saw two black holes of:
- 50.6 and 34.3 solar masses merging to produce one of 80.3 solar masses,
- 39.6 and 29.4 solar masses merging to produce one of 65.6 solar masses,
- 35.6 and 30.6 solar masses merging to produce one of 63.1 solar masses,
- 35.5 and 26.8 solar masses merging to produce one of 59.8 solar masses, and
- 35.2 and 23.8 solar masses merging to produce one of 56.4 solar masses.
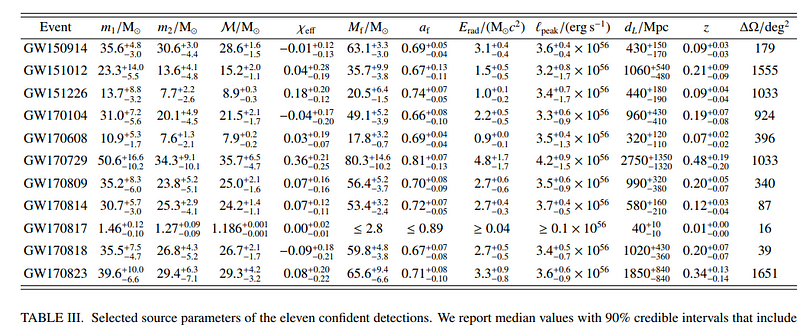
As we can clearly see, black holes above 20 solar masses are not only common, they’re commonly seen by LIGO and other gravitational wave detectors in the act of merging together, producing even larger black holes that can easily meet or exceed the 70 solar masses observed in this new study.
In the study itself, the authors note that this 70 solar mass black hole was found because it’s in a binary orbit with another massive star: a B-class star, which is short-lived and massive itself, a candidate for going supernova and creating a black hole on its own. But this is exactly where you’d expect to find a 70 solar mass black hole! There’s one simple reason for this that most astronomers rarely reference: star systems don’t just come in singlets and binaries, but that three-or-more stars are often found in the same system, and could easily lead to massive black holes that merge together while still having remaining stellar companions.
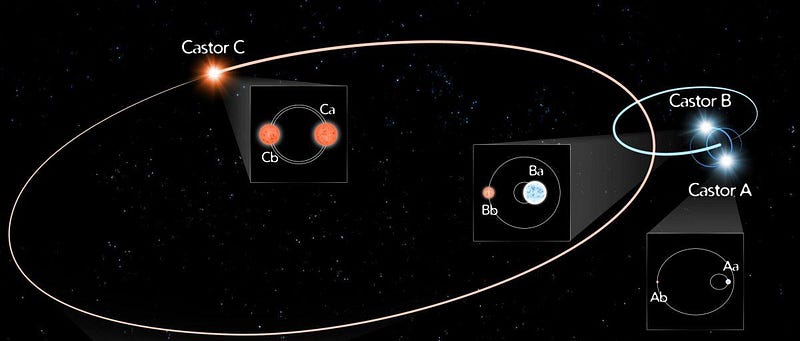
If we were to take a look at the nearest star systems to our own, we’d find that within about 25 parsecs (around 82 light-years), there are approximately 3,000 stars. But if we look at how those stars are bound together, we’d find that:
- around 50% of them are singlet systems like our Sun, with only one star,
- while 35% are binary systems, with two stars,
- approximately 10% are trinary systems, with three stars,
- about 3% are quadruple systems with four stars,
- and the remaining 2% have five or more stars,
- with notable Castor (above) being a sextuple system.
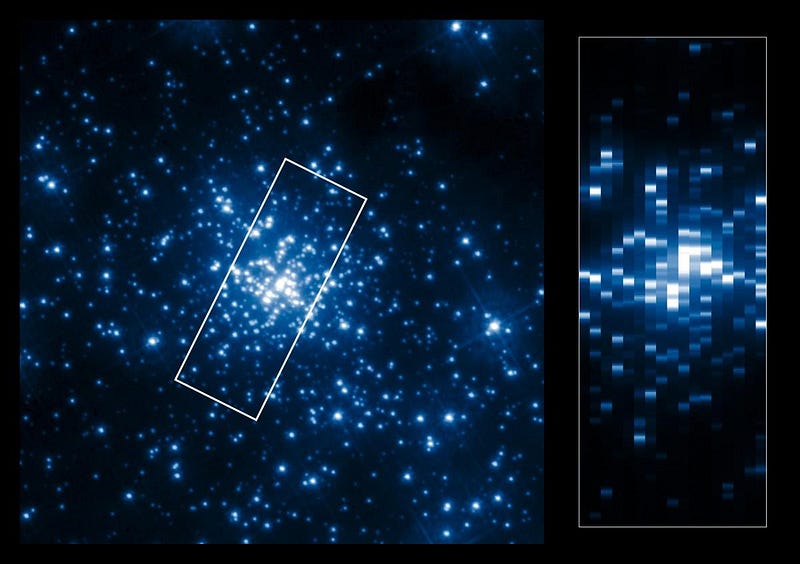
When we look at the largest, brightest star-forming regions of all, which contain the newest collections of massive stars, we find that dense clusters of comparable-mass stars are actually very common. It’s very easy to imagine a scenario where:
- a large number of star systems with three or more massive stars is created,
- at least two of them form black holes, whether by Type II (standard core-collapse) supernova, Type Ib or Ic (stripped-core) supernovae, or direct collapse,
- those multiple black holes merge together to create an even more massive one,
- while still being orbited by at least one additional star.
This isn’t fantasy or science-fiction; this is putting together four individual steps that have each been observed alone, but which humanity simply hasn’t existed for long enough to see them all happen in one sequential set of events.
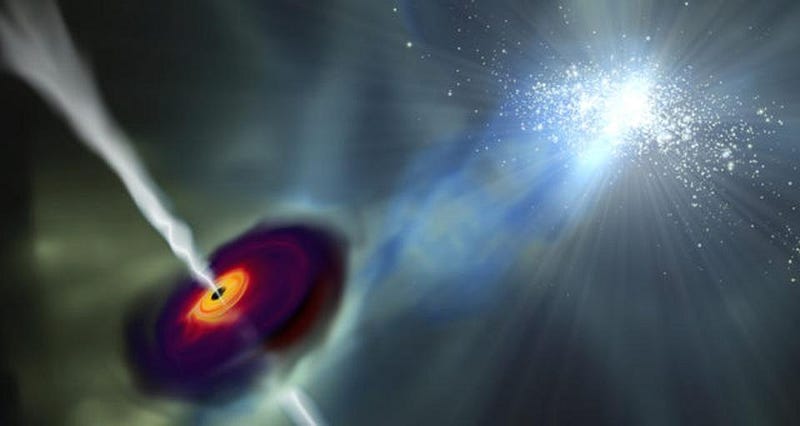
There’s nothing that a good scientist likes more than a surprise: where a theory or model makes explicit predictions that cannot explain the observations. But that’s not what we have here at all. Instead, we have one particular theory that we know is both oversimplified and overly restrictive to the point where it does not describe the Universe we have already observed, and it fails to describe a new observation as well.
The new observation itself is newsworthy, in that a stellar mass black hole this massive — reaching 70 solar masses — has never been seen in a binary system before. But the black hole itself absolutely should exist, as this makes it the fourth known black hole over 60 solar masses. Moreover, it’s consistent with what’s theoretically expected in a more realistic Universe, like the one we inhabit.
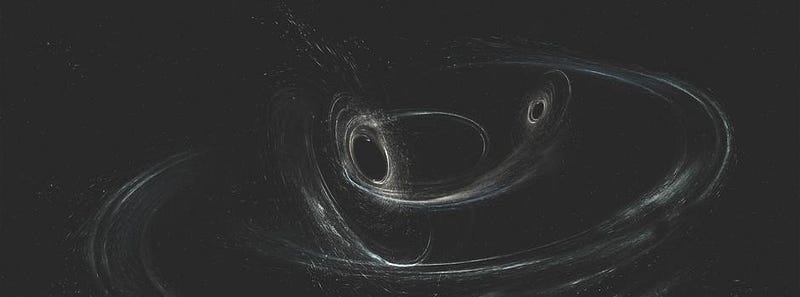
Astronomers aren’t perplexed by this object (or similar ones to it) at all, but rather are fascinated with uncovering the details of how they formed and how common they truly are. The mystery isn’t why these objects exist at all, but rather how the Universe makes them in the abundances we observe. We don’t falsely generate excitement by spreading misinformation that diminishes our knowledge and ideas prior to this discovery.
In science, the ultimate rush comes from discovering something that furthers our understanding of the Universe within the context of everything else we know. May we never be tempted to pretend anything else is the case.
Ethan Siegel is the author of Beyond the Galaxy and Treknology. You can pre-order his third book, currently in development: the Encyclopaedia Cosmologica.