What if Einstein never existed?
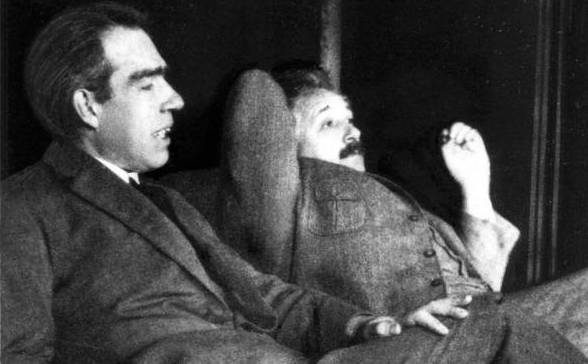
- From the speed of light to E = mc² to general relativity and more, no scientist in history has contributed more to human knowledge than Albert Einstein.
- Yet many others were working on the same sets of problems, and they may have made the same key advances even if Einstein were never present.
- If Einstein had never existed, however, would science have still advanced to its present state by today? It’s a fascinating question to explore.
If you ask the average person to name one scientist from any time or place in history, one of the most common names you’re likely to hear is Albert Einstein. The iconic physicist was responsible for a remarkable number of scientific advances during the 20th century, and perhaps single-handedly overthrew the Newtonian physics that had dominated scientific thought for more than 200 years. His most famous equation, E = mc², is so prolific that even people who don’t know what it means can recite it. He won the Nobel Prize for advances in quantum physics. And his most successful idea — the general theory of relativity, our theory of gravity — remains undefeated in all tests more than 100 years after Einstein first proposed it.
But what if Einstein had never existed? Would others have come along and made precisely the same advances? Would those advances have come quickly, or would they have taken such a long time that some of them might not yet have occurred? Would it have taken a genius of equal magnitude to bring his great achievements to fruition? Or do we severely overestimate just how rare and unique Einstein was, elevating him to an undeserved position in our minds based on the fact that he was simply in the right place at the right time with the right set of skills? It’s a fascinating question to explore. Let’s dive in.
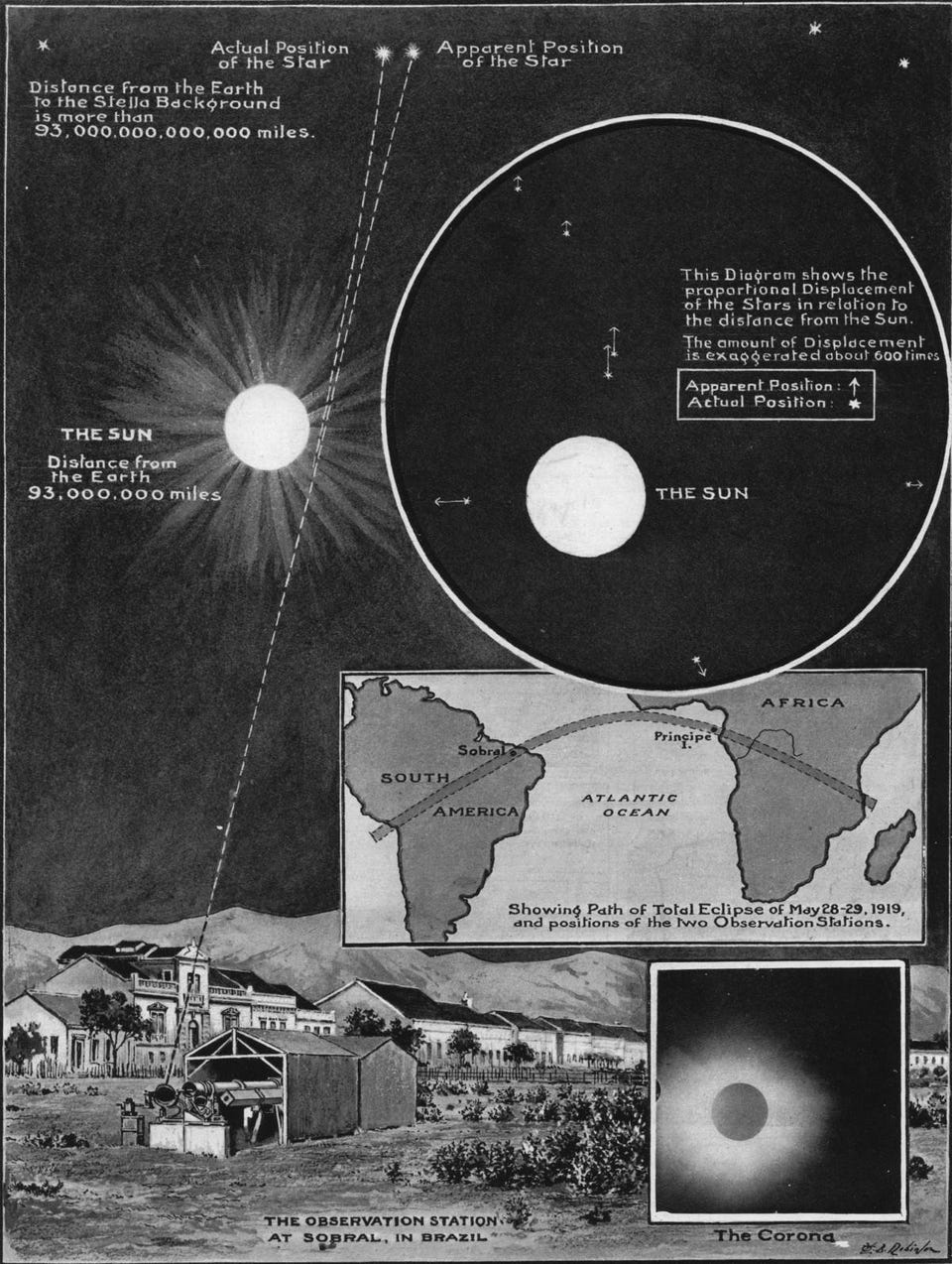
Credit: London Illustrated News, 1919
Physics before Einstein
Einstein had what’s known as his “miracle year” in 1905, when he published a series of papers that would go on to revolutionize a number of areas in physics. But just prior to that, a great number of advances had recently occurred that threw many long-held assumptions about the Universe into great doubt. For over 200 years, Isaac Newton had stood unchallenged in the realm of mechanics: both in the terrestrial and celestial realms. His law of universal gravitation applied just as well to objects in the Solar System as it did to balls rolling down a hill, or cannonballs fired from a cannon.
In the eyes of a Newtonian physicist, the Universe was deterministic. If you could write down the positions, momenta, and masses of every object in the Universe, you could calculate how each of them would evolve to arbitrary precisions at any moment in time. Additionally, space and time were absolute entities, and the gravitational force traveled at infinite speeds, with instantaneous effects. Throughout the 1800s, the science of electromagnetism was developed as well, uncovering intricate relationships between electric charges, currents, electric and magnetic fields, and even light itself. In many ways, it seemed that physics was almost solved, given the successes of Newton, Maxwell, and others.
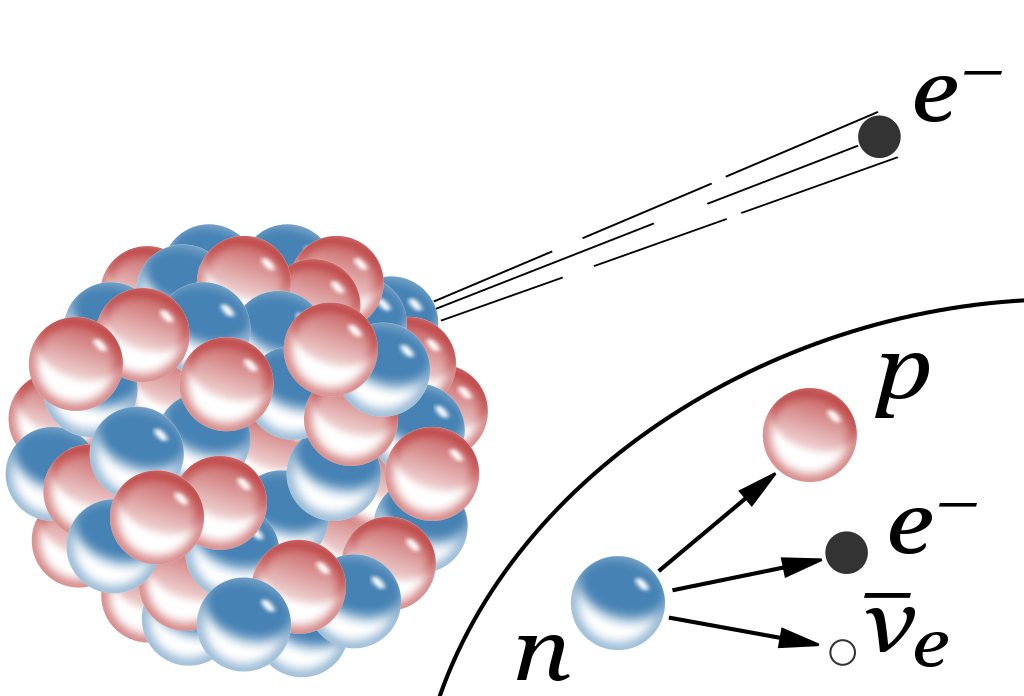
Credit: Inductiveload/Wikimedia Commons
Until, that is, it wasn’t. There were puzzles that seemed to hint at something new in many different directions. The first discoveries of radioactivity had already taken place, and it was realized that mass was actually lost when certain atoms decayed. The momenta of the decaying particles didn’t appear to match the momenta of the parent particles, indicating that either something wasn’t conserved or that something unseen was present. Atoms were determined not to be fundamental, but made of positively charged atomic nuclei and discrete, negatively charged electrons.
But there were two challenges to Newton that seemed, somehow, more important than all of the others.
The first confusing observation was the orbit of Mercury. Whereas all of the other planets obeyed Newton’s laws to the limits of our precision in measuring them, Mercury did not. Despite accounting for the precession of the equinoxes and the effects of the other planets, Mercury’s orbits failed to match predictions by a minuscule but significant amount. The extra 43 arc-seconds-per-century of precession led many to hypothesize the existence of Vulcan, a planet inner to Mercury, but none was there to be discovered.
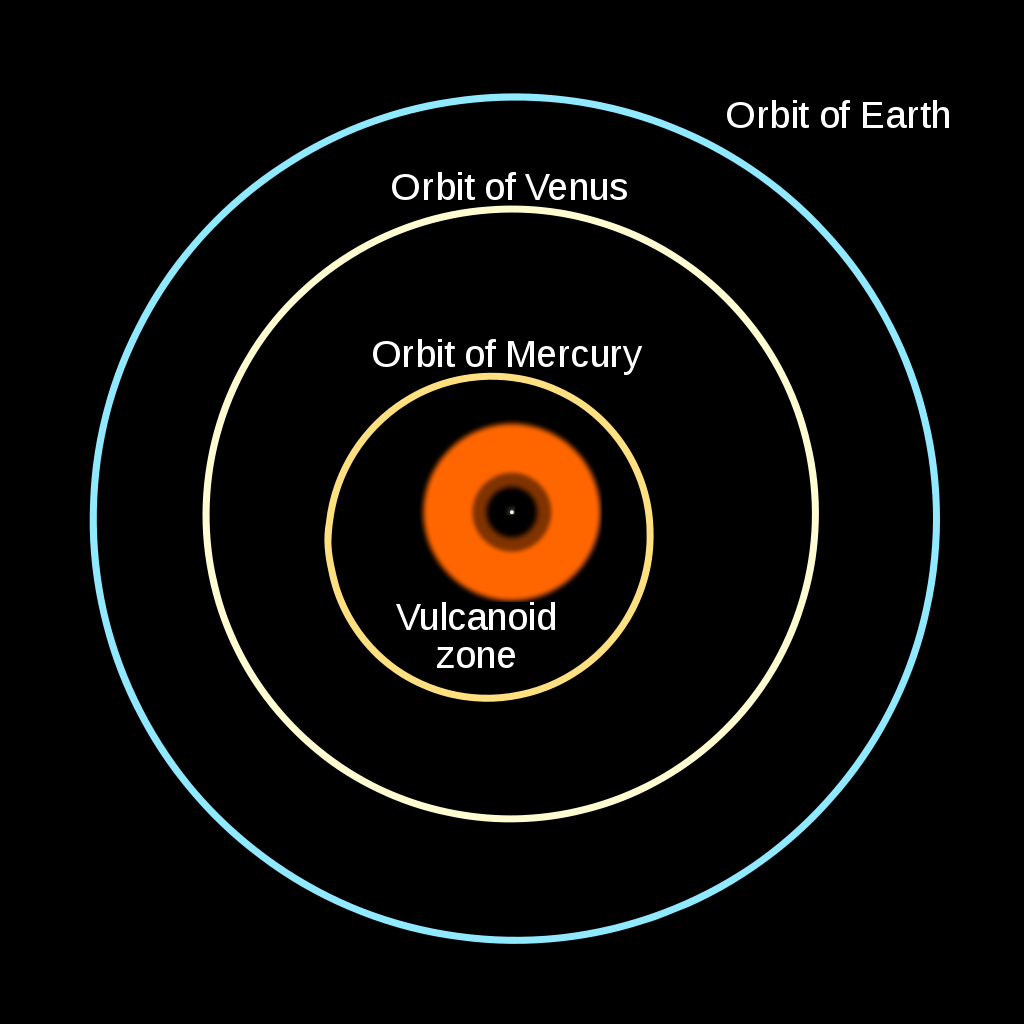
The second was perhaps even more puzzling: When objects moved close to the speed of light, they no longer obeyed Newton’s equations of motion. If you were on a train at 100 miles per hour and threw a baseball at 100 miles per hour in the forward direction, the ball would move at 200 miles per hour. Intuitively, this is what you’d expect to occur, and also what does occur when you perform the experiment for yourself.
But if you’re on a moving train and you shine a beam of light forward, backward, or any other direction, it always moves at the speed of light, regardless of how the train is moving. In fact, it’s also true regardless of how quickly the observer watching the light is moving.
Moreover, if you’re on a moving train and you throw a ball, but the train and ball are both traveling close to the speed of light, “addition” doesn’t work the way we’re used to. If the train moves at 60% the speed of light and you throw the ball forward at 60% the speed of light, it doesn’t move at 120% the speed of light, but only at ~88% the speed of light. Although we were able to describe what’s happening, we couldn’t explain it. And that’s where Einstein came onto the scene.
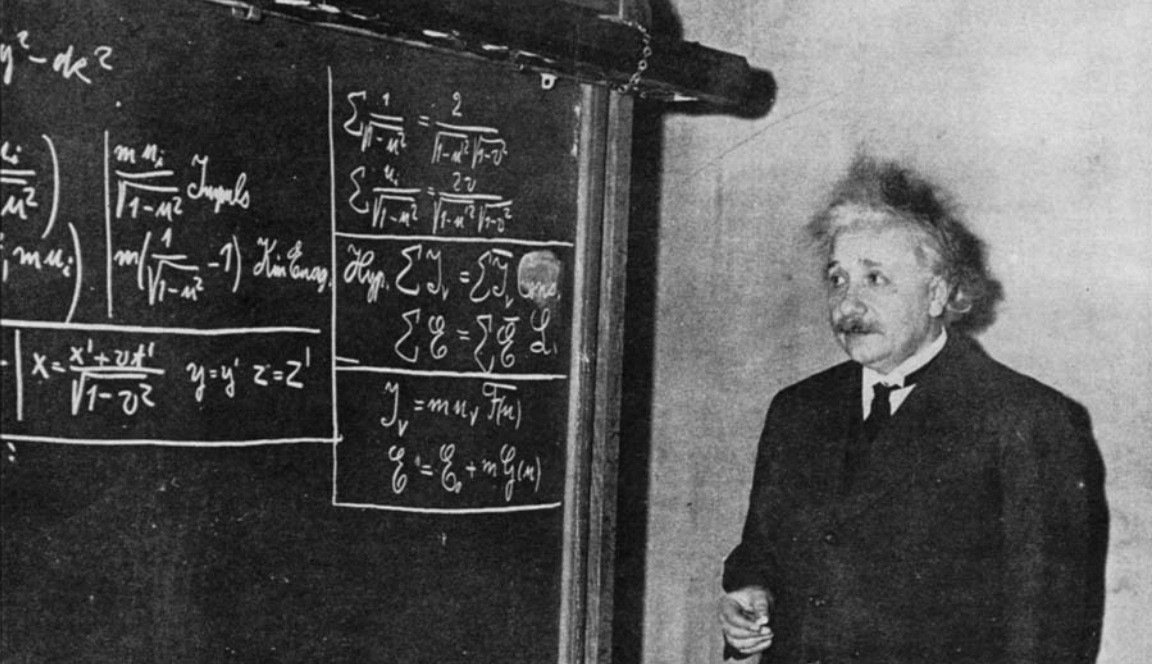
The advances of Einstein
Although it’s difficult to condense the entirety of his achievements into even a single article, perhaps his most momentous discoveries and advances are as follows.
The equation E = mc²: When atoms decay, they lose mass. Where does that mass go if it’s not conserved? Einstein had the answer: It gets converted into energy. Moreover, Einstein had the correct answer: It gets converted, specifically, into the amount of energy described by his famous equation, E = mc². It works the other way as well; we’ve since created masses in the form of matter-antimatter pairs from pure energy based on this equation. In every circumstance it’s ever been tested under, E = mc² is a success.
Special Relativity: When objects move close to the speed of light, how do they behave? They move in a variety of counterintuitive ways, but all are described by the theory of special relativity. There is a speed limit to the Universe: the speed of light in a vacuum, at which all massless entities in a vacuum move precisely. If you have mass, you can never reach, but only approach that speed. The laws of special relativity dictate how objects moving near the speed of light accelerate, add or subtract in velocity, and how time dilates and lengths contract for them.
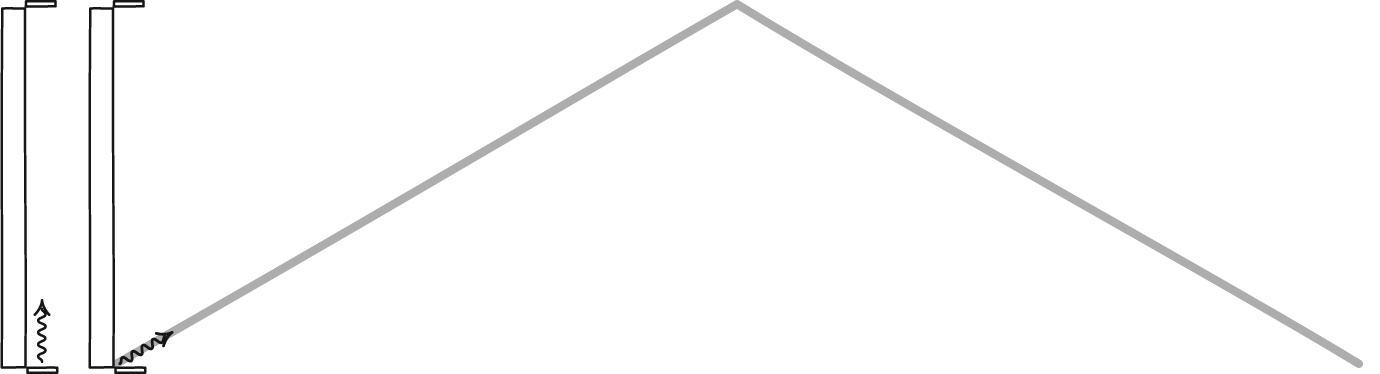
Credit: John D. Norton/University of Pittsburgh
The photoelectric effect: When you shine direct sunlight on a piece of conducting metal, it can kick the most loosely held electrons off of it. If you increase the light’s intensity, more electrons get kicked off, while if you decrease the light’s intensity, fewer electrons get kicked off. But here’s where it gets weird: Einstein discovered that it wasn’t based on the light’s total intensity, but on the intensity of light above a certain energy threshold. Ultraviolet light only would cause the ionization, not visible or infrared, regardless of the intensity. Einstein showed that light’s energy was quantized into individual photons, and that the number of “ionizing photons” determined how many electrons got kicked off; nothing else would do it.
General relativity: This was the biggest, most hard-fought revolution of all: a new theory of gravity governing the Universe. Space and time were not absolute, but made a fabric through which all objects, including all forms of matter and energy, traveled. Spacetime would curve and evolve owing to the presence and distribution of matter and energy, and that curved spacetime told matter and energy how to move. When put to the test, Einstein’s relativity succeeded where Newton failed, explaining Mercury’s orbit and predicting how starlight would deflect during a solar eclipse. Since it was first proposed, General Relativity has never been experimentally or observationally contradicted.
In addition to this, there were many other advances that Einstein himself played a major role in initiating. He discovered Brownian motion; he co-discovered the statistical rules under which boson particles operated; he contributed substantially to the foundations of quantum mechanics through the Einstein-Podolsky-Rosen paradox; and he arguably invented the idea of wormholes through the Einstein-Rosen bridge. His scientific career of contributions was truly legendary.
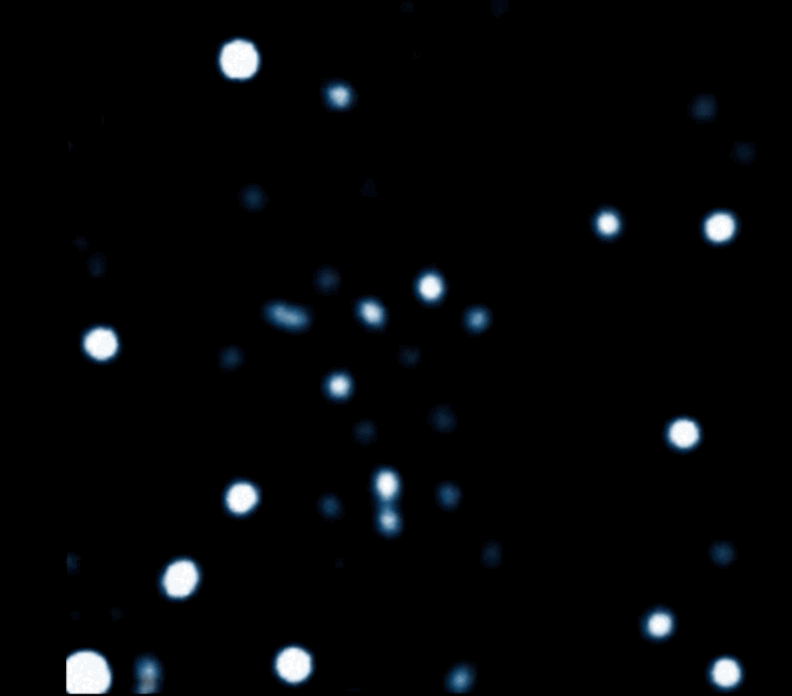
(Credit: ESO/MPE)
Would physics have advanced equally without Einstein?
And yet, there are many reasons to believe that despite the unparalleled career that Einstein had, the full suite of advances that were made by Einstein would have been made by others in very short order without him. It’s impossible to know for certain, but for all that we laud “the genius of Einstein” and hold him up as a singular example of how one incredible mind can change our conception of the Universe — as he, in fact, actually did — pretty much everything that occurred on account of Einstein would have occurred without him.
Prior to Einstein, back in the 1880s, physicist J.J. Thomson, discoverer of the electron, began thinking that the electric and magnetic fields of a moving, charged particle must carry energy with them. He attempted to quantify the amount of that energy. It was complicated, but a simplified set of assumptions allowed Oliver Heaviside to make a calculation: He determined the amount of “effective mass” that a charged particle carried was proportional to the electric field energy (E) divided by the speed of light (c) squared. Heaviside had a proportionality constant in there of 4/3 that was different from the true value of 1 in his 1889 calculation, as would Fritz Hasenöhrl in 1904 and 1905. Henri Poincaré independently derived E = mc² in 1900, but didn’t understand the implications of his derivations.
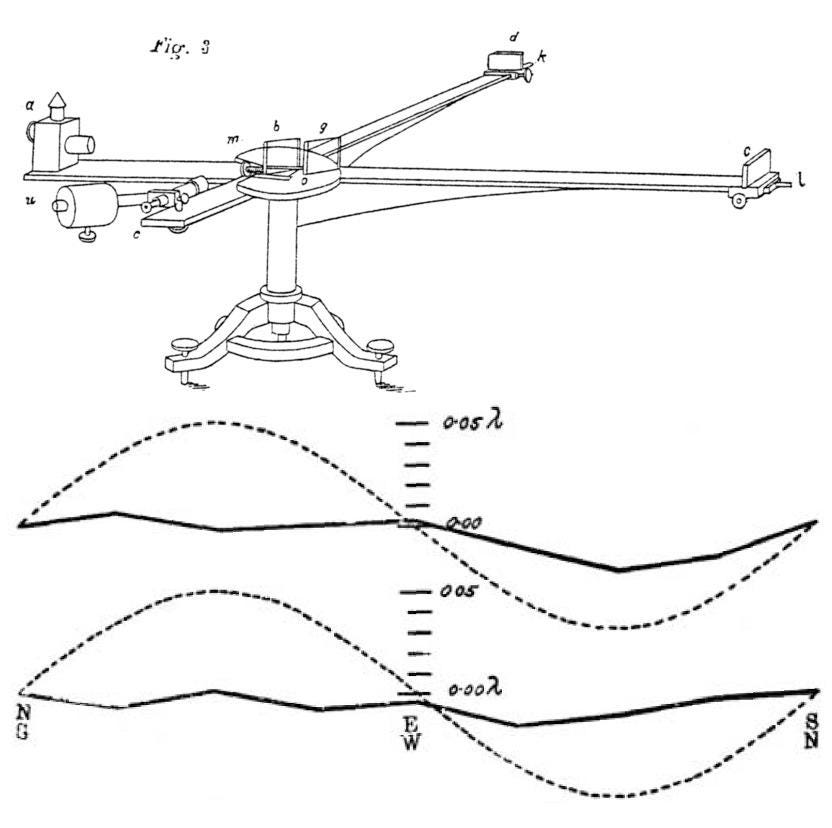
Credit: A.A. Michelson 1881 (top), A.A. Michelson and E.W. Morley 1887 (bottom)
Without Einstein, we were already perilously close to his most famous equation; it seems unrealistic to expect we wouldn’t have gotten the rest of the way there in short order had he not come along.
Similarly, we were already extremely close to special relativity. The Michelson-Morley experiment had demonstrated that light always moved at a constant speed, and it had disproven the most popular aether models. Hendrik Lorentz had already uncovered the transformation equations that determined how velocities added and how time dilated, and independently along with George FitzGerald, determined how lengths contracted in the direction of motion. In many ways, these were the building blocks that led Einstein to develop the theory of special relativity. However, it was Einstein who put it together. Again, it’s difficult to imagine that Lorentz, Poincaré, and others working at the interface of electromagnetism and the speed of light wouldn’t have taken similar leaps to arrive at this profound conclusion. Even without Einstein, we were already so close.
Max Planck’s work with light set the stage for the discovery of the photoelectric effect; it surely would have occurred with or without Einstein.
Fermi and Dirac worked out the statistics for fermions (the other type of particle, besides bosons), while it was Satyendra Bose who worked them out for the particles that bear his name; Einstein was merely the recipient of Bose’s correspondence.
Quantum mechanics, arguably, would have developed just as well in the absence of Einstein.
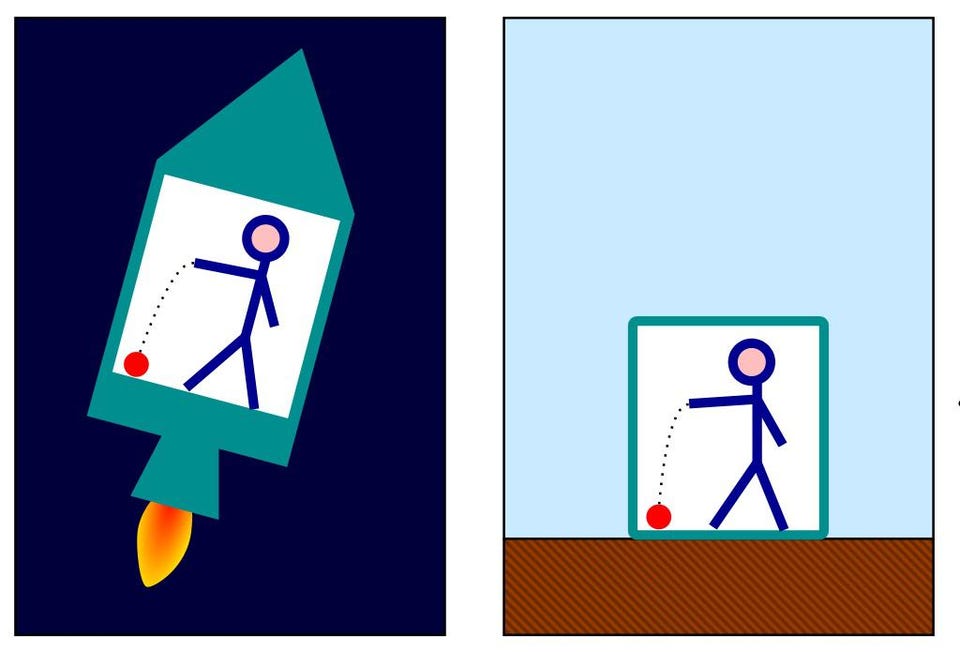
Credit: Markus Poessel/Wikimedia commons; retouched by Pbroks13
But general relativity is the big one. With special relativity already under his belt, Einstein set about to fold in gravity. While Einstein’s equivalence principle — the realization that gravitation caused an acceleration, and that all accelerations were indistinguishable to the observer — is what led him there, with Einstein himself calling it “his happiest thought” that left him unable to sleep for three days, others were thinking along the same lines.
- Poincaré applied special relativity to the orbit of Mercury, finding that he could account for about ~20% of the observed “extra” precession by folding it in.
- Hermann Minkowski, Einstein’s former professor, formulated the idea of spacetime, weaving space and time together into an inextricable “fabric.”
- Simon Newcomb and Asaph Hall modified Newton’s law of gravitation to account for Mercury’s precession, offering a hint that a new theory of gravity would solve the dilemma.
- Perhaps most compellingly, mathematician David Hilbert was also playing with non-Euclidean geometry, formulating the same “action” principle as Einstein for motion in the context of gravity, where the action principle leads to the Einstein field equations. Although Hilbert didn’t quite have the physical implications correct, we still call it the Einstein-Hilbert action today.
Of all the advances that Einstein made, this was the one that his peers were farthest behind when he put it forth. Still, while it might have taken many years or even decades, the fact that others were already so close to thinking precisely along the same lines as Einstein leads us to believe that even if Einstein had never existed, general relativity would eventually have fallen into the realm of human knowledge.

Credit: LucasVB
We typically have a narrative in how science advances: that one individual, through a sheer stroke of genius, spots the key advance or way of thinking that everyone else had missed. Without that one individual, humanity would never have gained that remarkable knowledge that was stored away.
But when we examine the situation in greater detail, we find that many individuals were often nipping at the heels of that discovery just before it was made. In fact, when we look back through history, we find that many people had similar realizations to one another at about the same time. Alexei Starobinskii put many of the pieces of inflation together before Alan Guth did; Georges Lemaître and Howard Robertson put together the expanding Universe before Hubble did; and Sin-Itiro Tomonaga worked out the calculations of quantum electrodynamics before Julian Schwinger and Richard Feynman did.
Einstein was the first to cross the finish line on a number of independent and remarkable scientific fronts. But had he never come along, many others were close behind him. Although he may have possessed every bit of dazzling genius that we often attribute to him, one thing is almost certain: Genius is not as unique and rare as we often assume it to be. With a lot of hard work and a little luck, almost any properly trained scientist can make a revolutionary breakthrough simply by stumbling upon the right realization at the right time.