No, Melting Quarks Will Never Work As An Energy Source
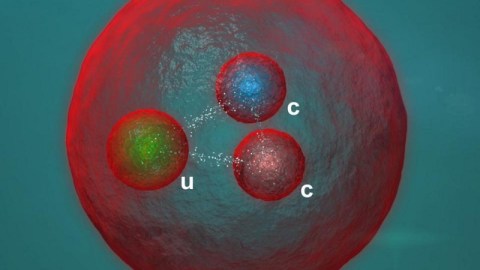
There’s more to powering the world than releasing energy.
When it comes to the ultimate dream of clean, efficient, and prolific energy sources, it’s hard to do better than the secrets held within the interior of an atom. While conventional energy sources rely on chemical-based energy and the atomic/molecular transitions of electrons, nuclear energy is far more efficient. For the same amount of mass, a single atomic nucleus, either when split (for an atom like uranium) or fused together (in the case of hydrogen) can give off up to a million times the amount of energy of a combustion reaction. Recently, “melting quarks” have been discovered to be up to ten times more energy-efficient than fusion reactions. But while fusion and fission both hold tremendous potential for revolutionizing the world’s energy, melting quarks will never work. Here’s the science of why.
The way nuclear fusion works is by taking stable, bound states of quarks (like protons, neutrons, and composite nuclei) and bringing them together under high-energy, high-density conditions. When you overcome the electrostatic force and get these charged nuclei close enough, their quantum wavefunctions begin to overlap, meaning there’s a finite probability that they’ll fuse into a heavier, more stable nucleus. When this occurs, a significant amount of energy is released: about 0.7% of the rest mass energy of the initial reactants. Via Einstein’s most famous equation, E = mc², that mass gets converted into energy, the ultimate goal of a fusion reaction.
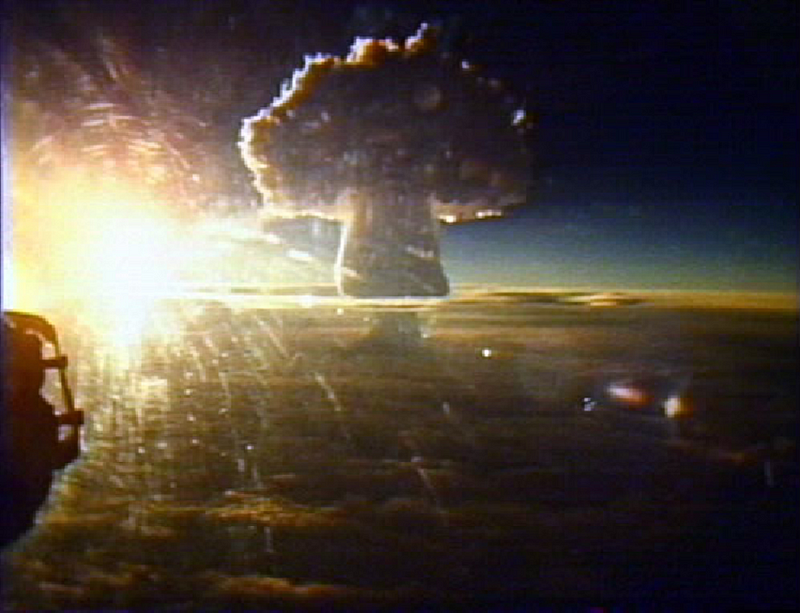
But normal nuclear bound states, even the unstable ones, are made up of up and down quarks only, including the proton, the neutron, and every element on the periodic table. There are a myriad of other possibilities, however, since there are four other types of quark known: strange, charm, bottom, and top. We’ve even made bound state analogies to the proton and neutron with strange, charm, and bottom quarks inside. If we can fuse protons, neutrons, and other bound quark states together, perhaps we can fuse these strange, charm, and bottom “baryons” together as well. (A baryon is any combination of three quarks, bound together.)
Even though they only exist for fractions of a second, we can perform detailed calculations and simulations with these particles. We can learn exactly how they’ll behave, given that we understand the laws of physics. And in a new study, scientists Marek Karliner and Jonathan L. Rosner have demonstrated that an unprecedentedly efficient “melting quark” reaction is possible.
Unlike in standard nuclear fusion, where two light nuclei will fuse together to produce a heavier one — one with a higher atomic mass number and a greater total number of quarks — a “melting quark” reaction keeps the number of quarks inside at three, total. Instead, each of the two reacting baryons contains one heavy quark, like a charm quark or a bottom quark, and forms a single doubly-heavy baryon at the end, along with a boring light baryon like a normal proton or neutron. Unlike standard fusion reactions, which emit around half-a-percent of their mass as energy, the binding energy between these doubly-charmed (or doubly-bottomed) baryons is nearly 10 times as large, resulting in a reaction where up to 4% of the total mass is converted into energy.
Your mind might immediately race to unprecedented applications. “This could revolutionize our energy needs,” you might think. “This could be the most efficient weapon of all time,” the military-minded part of you says. But the truth is that these are just pipe dreams, never to be realized with any sort of practical application in the physical Universe.
Why not, you ask?
Because these particles are too unstable, and the amount of energy required to make them is far, far greater than the amount of energy you’d get out.
In order to create a particle with a heavy quark (strange, charm, bottom, etc.) in it, you have to collide other particles together at extremely high energies: enough to make equal amounts of matter and antimatter. Assuming you then make the two baryons you need (two charmed or two bottomed baryons, for instance), you must then have them interact under the right conditions — fast and energetic, but not too fast or too energetic — to cause that fusion reaction. And then, at last, you get that ~3–4% energy gain out.
But it cost you over 100% to make these particles in the first place! They’re also incredibly unstable, meaning they’ll decay to lighter particles on incredibly short timescales: a nanosecond or less. And, finally, when they do decay, you get 100% of your energy back, in the form of new particles and their kinetic energies. In other words, you don’t get any net energy out; you simply get out what you put in, but in a lot of different, hard-to-harness ways.
Nuclear fusion is the holy grail of energy because of many factors, including:
- the abundance and stability of the reactants,
- the controllable nature of the reaction,
- the large amount of energy-per-unit-mass liberated from the fusion itself,
- and the ease of harnessing the energy that comes out.
Melting quarks might have the edge when it comes to that third point, as a nearly tenfold increase in liberated energy suggests, but its catastrophic failure on all the other points renders it a scientific curiosity. Its potential application to the energy or weapons sector relies on unrealistic conditions, necessary to overcome the other barriers.
It’s still an incredibly important find to learn — even via simulation — how these bound-quark systems bind together and interact with one another. It’s important to understand how binding energy works, how much energy is liberated, and what form it takes when various unstable particles react. These steps forward are an integral part of nuclear and particle physics. But melting quarks will never work as an energy source or a weapons source, as the increased efficiency over traditional nuclear fusion at these high, unstable energies is far surpassed by the 100% efficiency of matter-antimatter annihilation. If you can make particles where melting quarks is a possibility, you can also create antimatter: the most energy-efficient source in the Universe. But for cheap, abundant, clean energy, nuclear fusion, notmelting quarks, is the wave of the future.
Ethan Siegel is the author of Beyond the Galaxy and Treknology. You can pre-order his third book, currently in development: the Encyclopaedia Cosmologica.