No, Black Holes Don’t Suck Everything Into Them
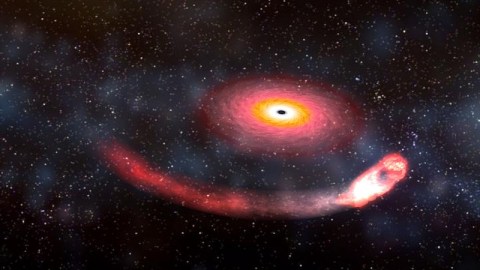
A vacuum cleaner is the wrong picture. Time to bust that myth.
There are no classes of object in our Universe more extreme than black holes. With so much mass present in such a tiny volume of space, they create a region around them where the curvature of space is so strong that nothing — not even light — can escape from its gravity once a certain boundary is crossed. That boundary is known as the event horizon, and anything from outside the event horizon that crosses inside will never get out.
This has led to a picture that most of us have in our heads about black holes that’s prevalent but incorrect: one where black holes suck all the matter from outside their event horizons into them. We think of black holes as cosmic vacuum cleaners, consuming everything that dares to approach their vicinity. Even though NASA itself has released videos illustrating this effect, it’s a complete falsehood. Black holes don’t suck, after all.
It’s easy to see how you’d think black holes would suck everything into them. Gravity is an attractive force, and black holes are the greatest collection of mass in a small volume of space you can possibly achieve. They are the densest cosmic monstrosities found in the entire Universe. When a massive object gets close to a black hole, it’s easy to intuit what you think should happen.
- An object approaches a black hole,
- the tidal forces tear it apart into streams,
- the black hole’s gravity attracts all of the stream-like matter,
- and then swallows it all, leaving no trace behind.
But this is perhaps the greatest cosmic misconception about black holes of all. While black holes do have event horizons, and while anything that crosses the event horizon can never get out, black holes aren’t the great cosmic devourers we make them out to be. Instead, they’re the messiest eaters imaginable.
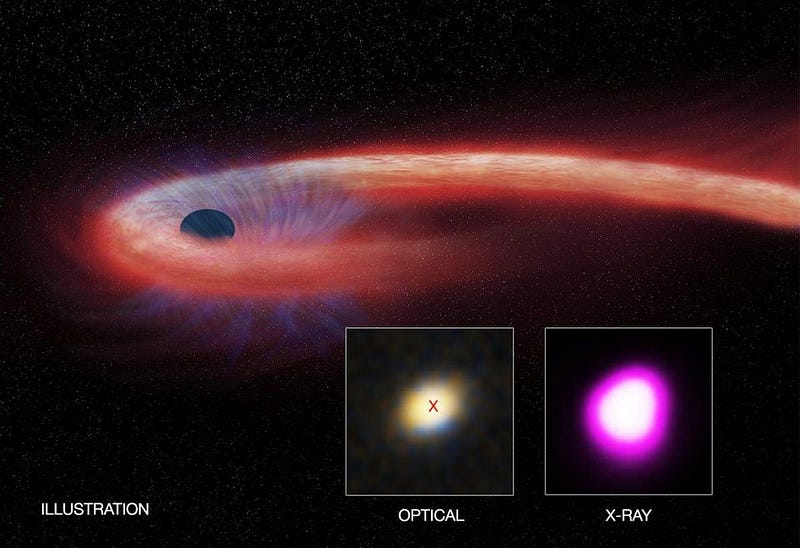
Don’t think of a vacuum cleaner when you think of black holes. Instead, it’s far more accurate — and far more fun, as I alluded to in my Brain Bar talk in Hungary — to think of black holes as giant cosmic Cookie Monsters.
If you’ve ever seen Cookie Monster get his hands on cookies, you’ll know what I’m talking about. Sure, every cookie in the nearby vicinity will find its way into the area near Cookie Monster’s mouth. The cookies get funneled inside towards it. But the overwhelming majority of the cookie matter that approaches the mouth of Cookie Monster won’t wind up getting devoured; instead, it gets spit out in all directions, having been accelerated by a variety of chaotic forces. If you’ve had a child (or been one) since the 1970s, you’ve probably seen it in action for yourself.
This might puzzle you, but let’s think about it a little more deeply, starting with planet Earth. How would you respond if you were asked the question, “does the Earth suck everything into it?”
Of course, the answer is pretty obviously “no.” Earth simply has gravity that attracts things to it, distorting the fabric of space around it and altering the paths of the objects that pass nearby. If those objects happen to strike the Earth — hitting the atmosphere, oceans, or surface of our planet — they’ll fall into (or onto) our world, but if not, they’ll escape from our gravitational pull. It’s a pretty straightforward exercise in both Newton’s and Einstein’s gravity to show that this is the case, and it agrees thoroughly with what we observe as far as spaceborne objects hitting or missing the Earth.
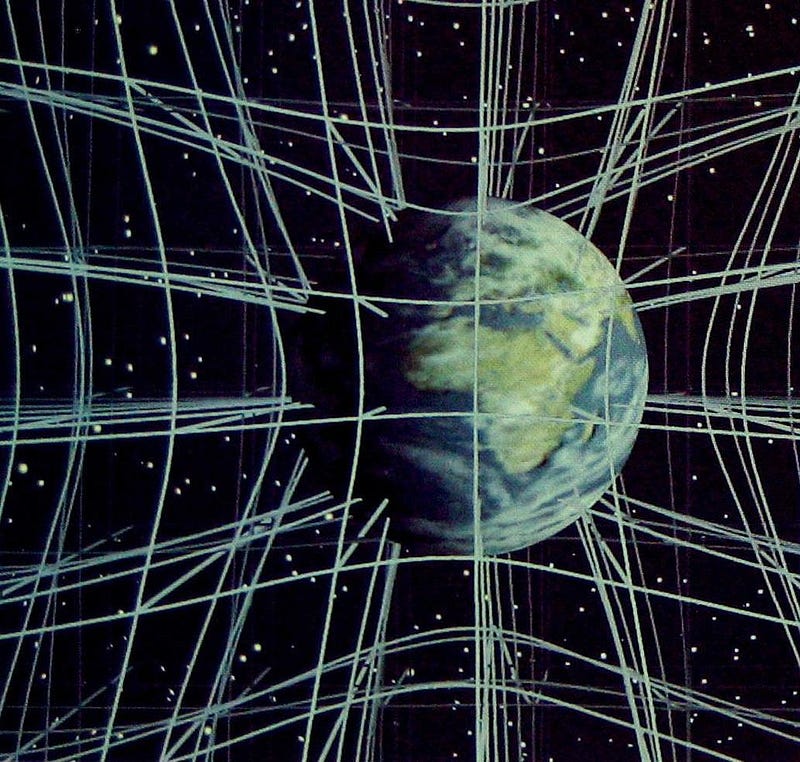
Now, let’s imagine the same exact puzzle, only this time, let’s replace the real, physical planet Earth with a black hole that’s exactly the same mass. Instead of taking up the volume of Earth, it would create an event horizon occupying a volume of space a little less than 2 cm in diameter.
Here’s the thing. If you examine the fabric of spacetime, you’ll find that outside of the volume that marked the boundary of Earth’s atmosphere when we looked at our planet as it is today, the curvature of space is identical whether you replace Earth with a black hole or not. All the objects that would miss planet Earth will still miss this black hole that’s the same mass as planet Earth. There is no additional sucking force at all. In fact, many of the objects that would have hit Earth previously will now miss the black hole. Only the rare objects that cross the event horizon — a mere 2 cm across (as opposed to ~12,700 km for the actual Earth) — will get swallowed.
This line of reasoning doesn’t just apply to Earth-mass black holes, but all black holes in the Universe. A black hole that’s the mass of the Sun will only be a few kilometers in diameter: smaller than any actual star, white dwarf, planet, or even neutron star in existence. The black hole at the center of the Milky Way, despite weighing in at 4 million Suns, will only be about 18 times the diameter of our Sun itself.
When you consider how big space actually is, and how much mass black holes actually have, you start to realize that event horizons are tiny. Yes, they have a lot of gravitational pull on the space in their vicinity, but that just causes the matter around them to accelerate rapidly. Believe it or not, that actually contributes to black holes devouring less matter than they would if only isolated, individual particles fell into it.
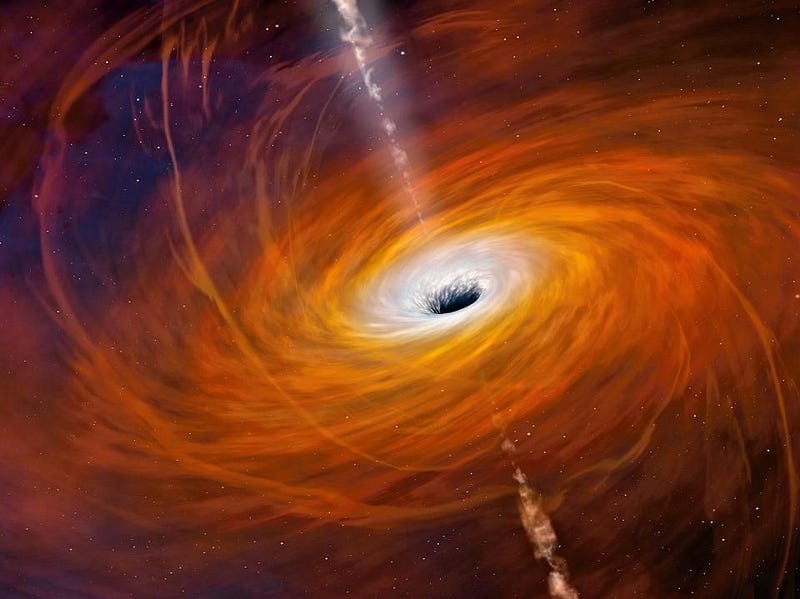
In the real Universe, you see, it isn’t isolated particles that represent the majority of mass that interacts with a black hole. Instead, the two most common snacks for a black hole are either stars or gas clouds.
A typical gas cloud in space is much larger than our Solar System is, with many spanning multiple light years in size, while a star that approaches a black hole will find itself spaghettified, or stretched into a long, thin strand aligned with the direction of the black hole. By time either of these options reach the event horizon of the black hole itself, they’re many, many times the size of the black hole’s event horizon. They’re also stretched in the direction approaching the black hole, compressed in the perpendicular direction, and heated, as particle-particle collisions can even cause the atoms inside to ionize and break up into free electrons and nuclei.
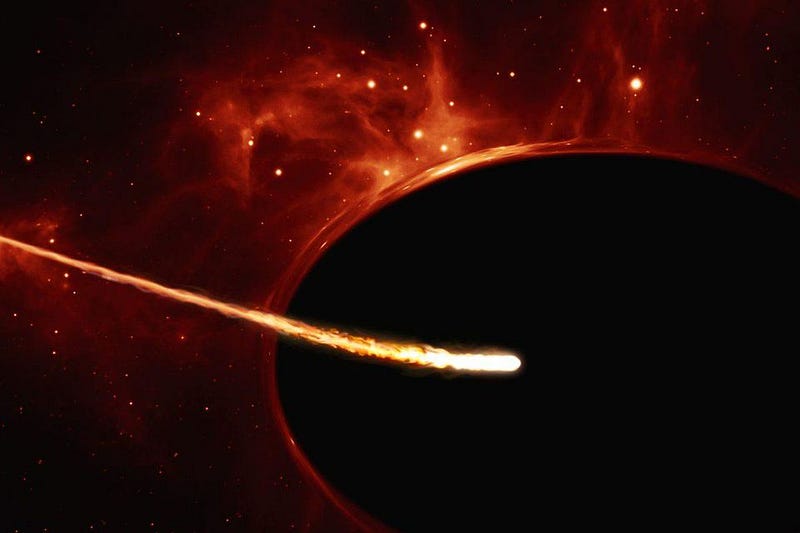
Sure, if some particle falls into the black hole’s event horizon, which some of the matter will inevitably do, it adds to the black hole’s mass, making it larger. But if a particle misses the event horizon itself and simply approaches near the black hole, it’s going to experience a tremendous acceleration instead. A charged particle in motion creates a magnetic field, and magnetic fields are spectacular at changing the direction of every other charged particle around them.
In particular, these particles will heat up, accelerate, emit light (in the form of cyclotron or synchrotron radiation), and will produce bipolar jets perpendicular to the plane of the black hole’s (or the accretion flow’s) rotation.
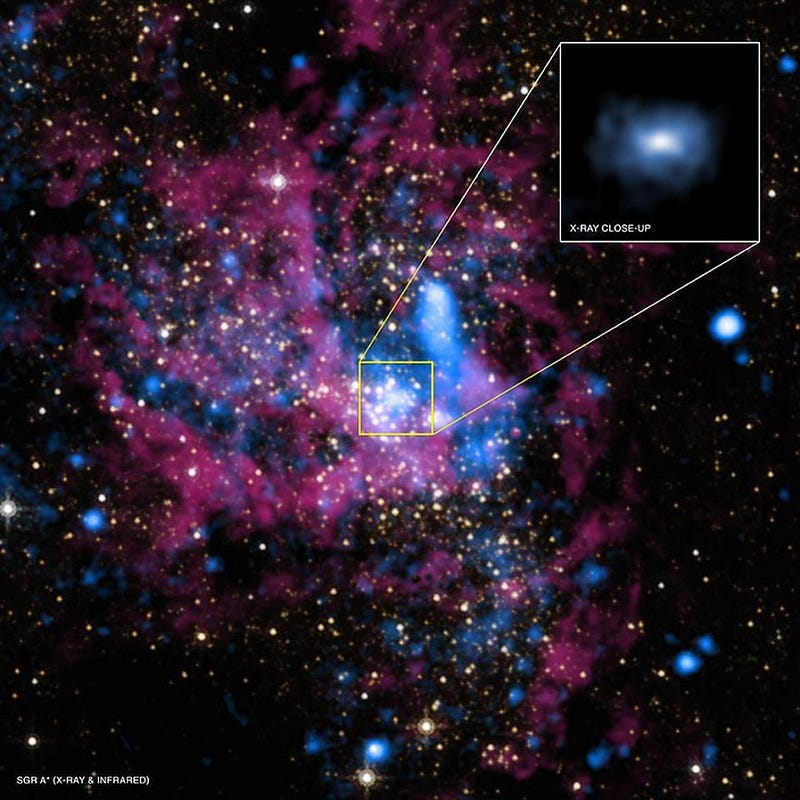
Considering that we’ve only seen our first image of a black hole’s event horizon a few months ago, you might think these arguments are completely theoretical. Not so! We actually have an incredible amount of observational evidence to support this picture.
- Black holes within our own galaxy appear to turn on-and-off in fast, incredible bursts of high energy emission: microquasars.
- The black hole at the center of the Milky Way appears to flare up on random occasions, emitting bursts of X-ray light due to passing, infalling, accelerating matter.
- Supermassive black holes at the centers of other galaxies — many of which are thousands of times the mass of our own supermassive black hole — can be active, emitting tremendous amounts of energy due to their acceleration and emission of matter and energy in exactly this predictable fashion.
We can often find evidence of this in many different wavelengths of light, even including visible signatures and jets in many instances.
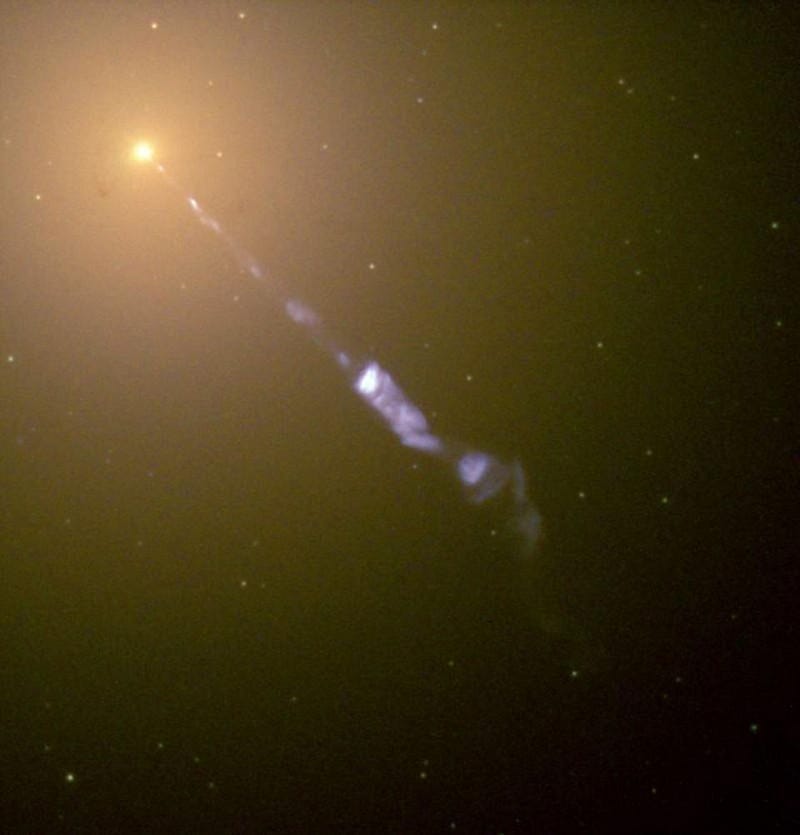
But whether it comes from asteroids, planets, stars, or hot or cold gas, most of the infalling matter doesn’t go into feeding the black holes that attracted them in the first place. Instead, just like when Cookie Monster eats a cookie, only a tiny fraction actually makes it past the boundary of the event horizon.
Due to the intense gravitational forces and the tremendous size mismatch between the relatively tiny black holes and the large clumps of matter that feed them, the vast majority of infalling matter finds itself spit back out in an intense, violent flurry. It’s estimated that, contrary to the popular picture, upwards of 90% of infalling matter will never make it inside a black hole at all. Instead, it’s spewed back out into the outer regions of the galaxy, where it can fuel the formation of new stars and return to the interstellar medium once again.
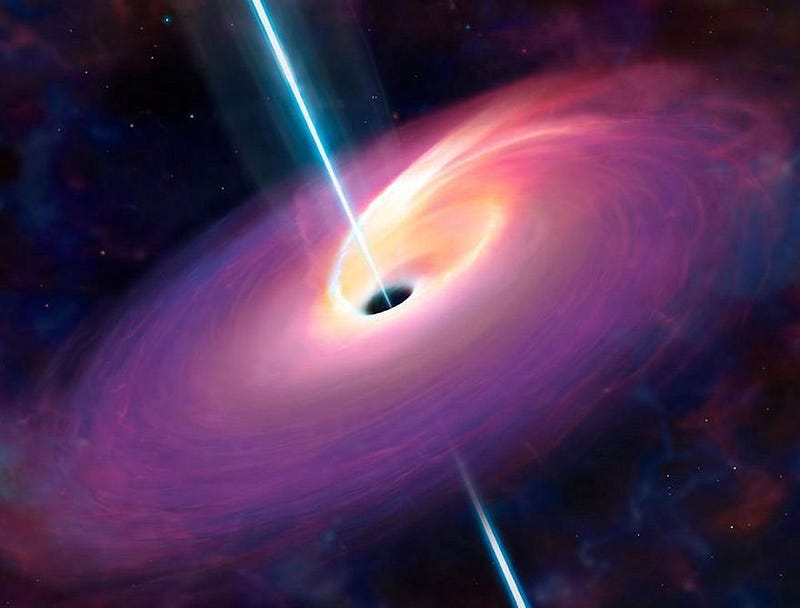
The fact of the matter is that black holes aren’t sucking anything in; there’s no force that a black hole exerts that a normal object (like a moon, planet, or star) doesn’t exert. In the end, it’s all just gravity. The biggest difference is that black holes are denser than most objects, occupying a much smaller volume of space, and capable of being far more massive than any other single object.
But matter is charged, accretion disks and flows are real, generate magnetic fields, and accelerate most of the infalling matter away from the event horizon itself. If you’ve ever had to deal with a young child who eats a quarter of their food while spilling the rest on their faces, the table and the floor, cheer up. You can always comfort yourself with this knowledge: at least they’re doing much better than a black hole.
Ethan Siegel is the author of Beyond the Galaxy and Treknology. You can pre-order his third book, currently in development: the Encyclopaedia Cosmologica.