New method could reveal novel, habitable exoplanets
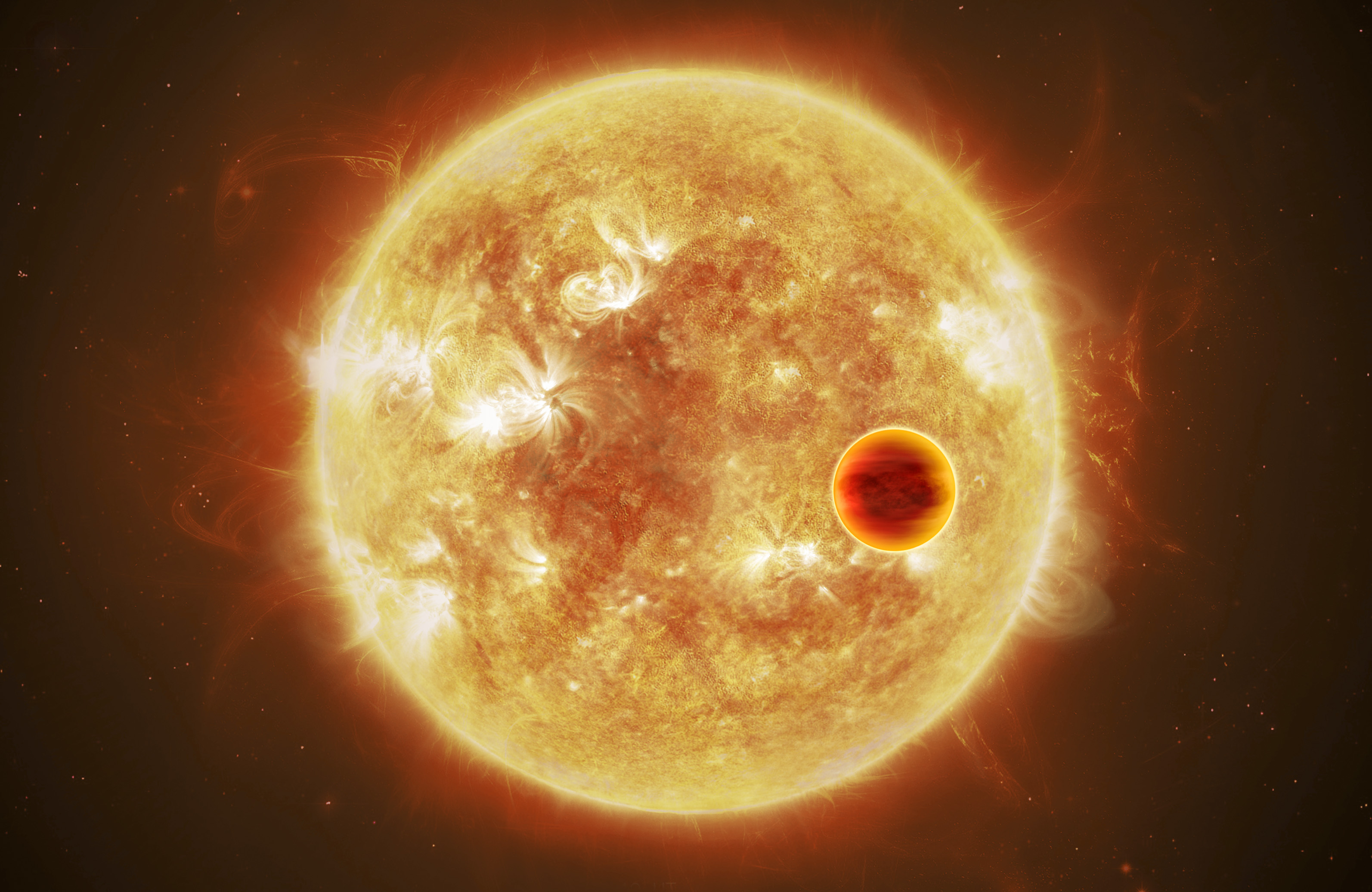
- To date, over 5000 exoplanets have been discovered: mostly around singlet stars, and mostly via the transit method, where a planet passes in front of its parent star.
- However, 50% of stars are found in multi-star systems, and the most common false positive “exoplanet candidate” turns out to be an eclipsing binary star system.
- Many of these systems might well be home to “Lagrange point exoplanets,” a type of exoplanet that’s never been seen.
- Simply looking for them could reveal a whole new population of potentially inhabitable worlds.
Out there in the Universe, wherever stars form from molecular clouds that are rich enough in heavy elements, it’s possible for rocky planets with the right ingredients for life to arise upon them to form. The quest for life beyond Earth — whether within our Solar System or on a world elsewhere within the Milky Way — is one of the proverbial holy grails of 21st century science. Just a little over 30 years ago, we knew only of the worlds within our Solar System as far as planets went; today, thanks largely to the transit methods and space-based observatories such as Kepler and TESS, the number of known exoplanets now exceeds 5000, and continues to grow.
But one has to wonder what we’re missing. Our searches for exoplanets have revealed large numbers of them with a wide variety of masses and sizes, but almost all of them have been found around singlet stars: stars that don’t have a binary companion or otherwise exist as a member of a multi-star system. There are perhaps about a dozen known circumbinary planets: where an exoplanet orbits two tightly-orbiting stars at a much greater distance than the distance separating the two central stars, but finding only ~0.2% of planets in systems that represent ~50% of all stars tells us something is missing.
Here’s one novel, spectacular idea that might close the gap, revealing planets within multi-star systems as never before.
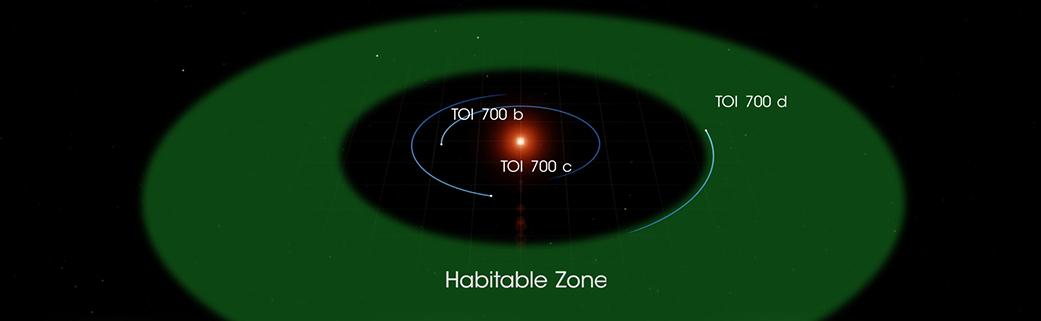
It’s important to recognize two things before we get started.
- Planets can only form and remain in locations that are sufficiently gravitationally stable. If a combination of gravitational forces would either eject or tear apart a planet in that location on timescales shorter than the existence of the stellar system in question, we cannot reasonably expect to find planets there.
- Fully half of all stars are members of multi-star systems; only 50% of stars exist in systems like our own Solar System: with one star and no others. Yet ~99.8% of the discovered planets have been found around singlet stars, indicating a tremendous bias in what our current searches are sensitive to.
There are a series of methods that we use to find exoplanets, or planets around stars other than our own. There’s direct imaging: useful for large planets that are sufficiently well-separated from their parent stars. There’s the stellar wobble (or radial velocity) method, where the gravitational tug on a star from its orbiting planet perturbs the star’s motion along our line-of-sight in a regular fashion, useful for massive-enough planets in close-enough orbits around their parent stars. But the most successful planet-finding method of all is the transit method, which reveals the presence of planets as they pass in front of — and block a fraction of the light emitted by — their parent stars.
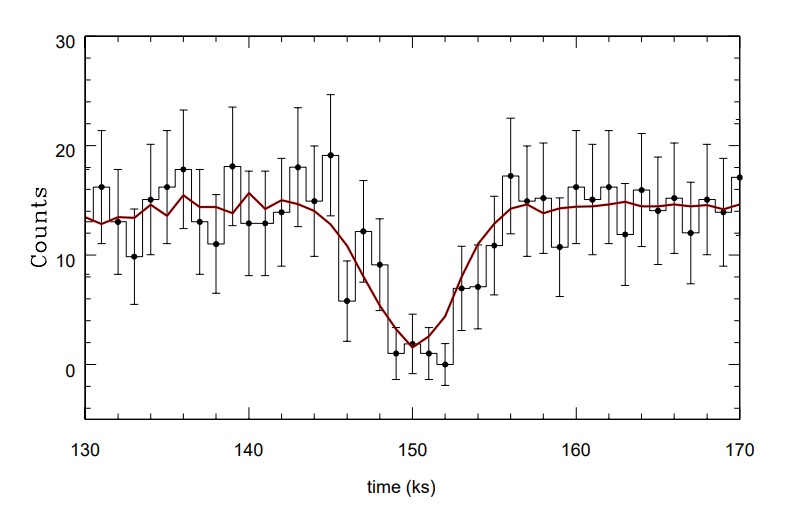
The way this works, observationally, is that we:
- watch a star for long periods of time,
- observe its flux,
- and look for “dips” in the observed flux over time.
There are, of course, many possible causes for this. The desired cause — the existence of an exoplanet that transits across the face of its parent star — will come along with a particular manifestation of how the flux dips. If it dips regularly, by the same magnitude, with a constant period, by a small amount that could feasibly correspond to the size of a planet, that makes an excellent planetary candidate. If follow-up measurements of the star, which requires some way of measuring the spectrum of the star, show that its spectral signatures periodically shift from red-to-blue and back again in alignment with the period of the flux dips already observed, that’s the gold-standard way for confirming a transiting exoplanet.
But although repeating flux dips are excellent ways to reveal exoplanets, simply seeing a set of them isn’t enough to declare that we have a confirmed exoplanet. That only reveals an exoplanet candidate; some sort of independent confirmation is required to promote a candidate to a confirmed exoplanet. And, as you might expect, some candidates don’t quite make the cut.
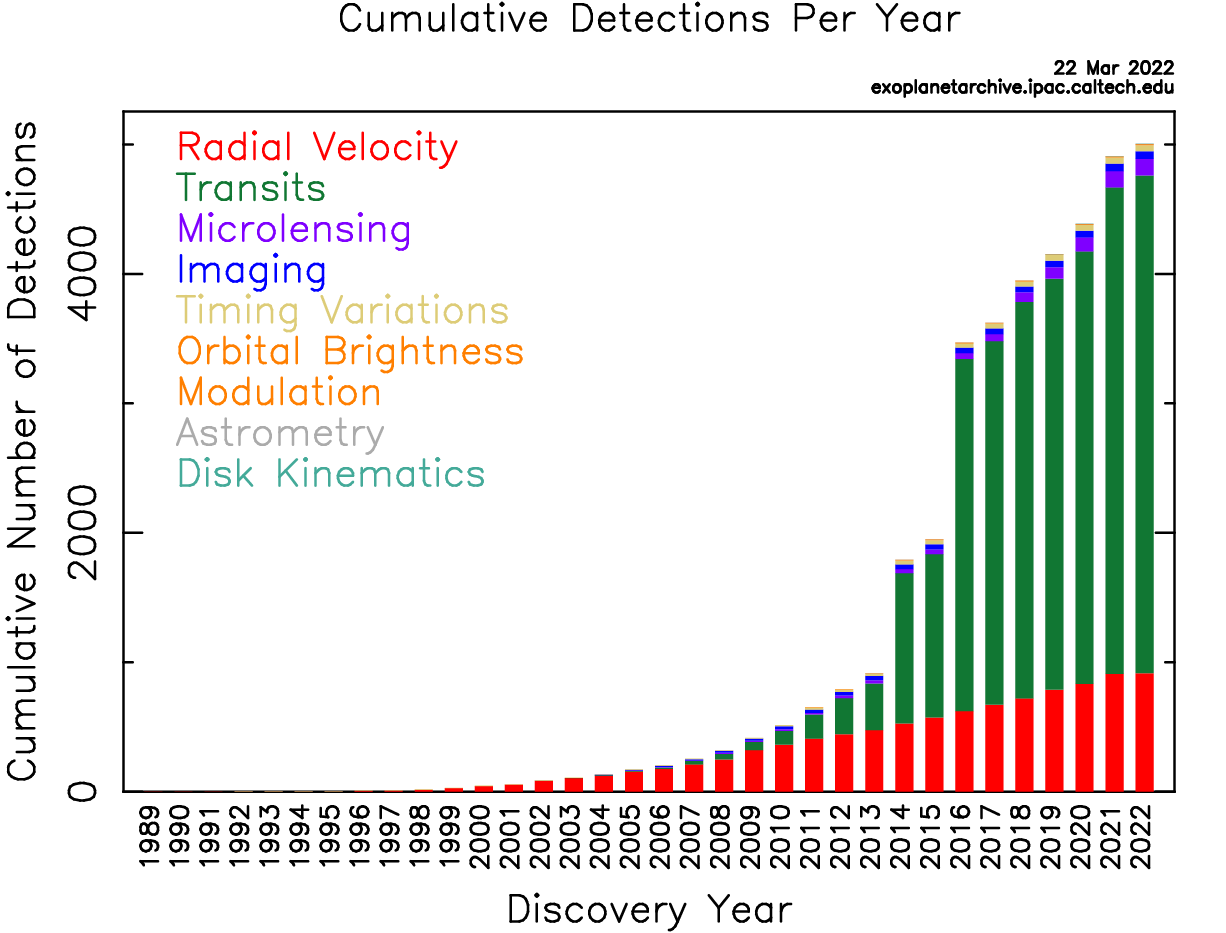
One confounding factor is intrinsic variability. We normally think of stars the same way we think of our Sun: its brightness remains relatively constant to an astounding precision. Because of sunspots, variations in the plasma temperature and density, as well as flares and mass ejections, the Sun can vary in brightness from its mean value by up to ~0.14%. Other stars have larger intrinsic variabilities, as their atmospheres can oscillate, they can flare more frequently and regularly than the Sun, and they can belch out dust, obscuring the star. These can lead to false positives: exoplanet candidates that have nothing to do with planets at all, but simply reflect the variable properties of the star we’re observing.
A second confounding factor, however, is the potential presence of a binary companion: an example of an extrinsic variable. When we look at a star from very far away, there’s an excellent chance that there are more than one stellar members that are a part of that system, but that the extreme distances mean that the multiple members are not resolvable. If the two stars dance in a “face-on” orientation to us, so that our perception of the multiple independent stellar disks never overlap, the flux will remain constant. But if the two stars move in an “edge-on” orientation relative to us, and their disks do overlap, they’ll exhibit regular dips in their flux as the two stars aren’t always fully visible at the same time.
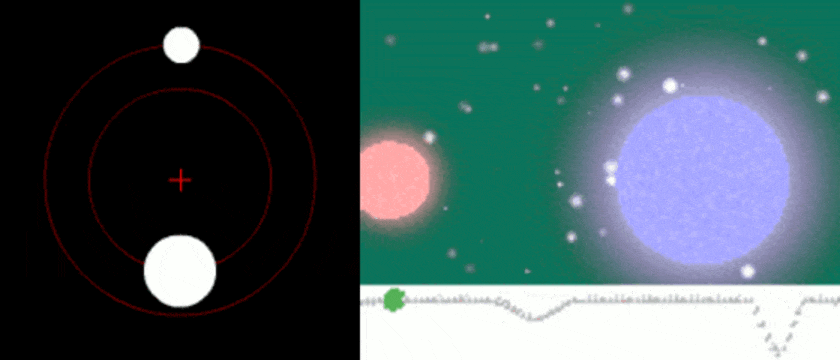
This type of configuration is known as an eclipsing binary, and is the single most common confounding source in modern exoplanet-hunting. From NASA’s Kepler mission — remember, our most successful planet-finding mission of all time — approximately half of all of Kepler’s exoplanet candidates turned out not to be planets at all, but rather represented one of the confounding factors discussed above. Almost all of the exoplanet candidates that didn’t turn out to be planets turned out to be eclipsing binaries instead: binary stars with significant overlap, relative to our line-of-sight, in their orbital dance.
This shouldn’t come as a very big surprise. If we’re looking for the signal of a transiting planet in front of a star, then it’s easy to see how a similar geometry, except with a larger, more massive, and more luminous object than a planet, could result in a “false positive” for the type of signal we’re seeking. In fact, although a 50% false positive rate might seem like an unacceptably high number, the Kepler mission represented a tremendous improvement over previous exoplanet studies. Prior to the Kepler mission, approximately 90% of all exoplanet candidates turned out not to be confirmed; having only 50% turn out to be eclipsing binaries is pretty good!
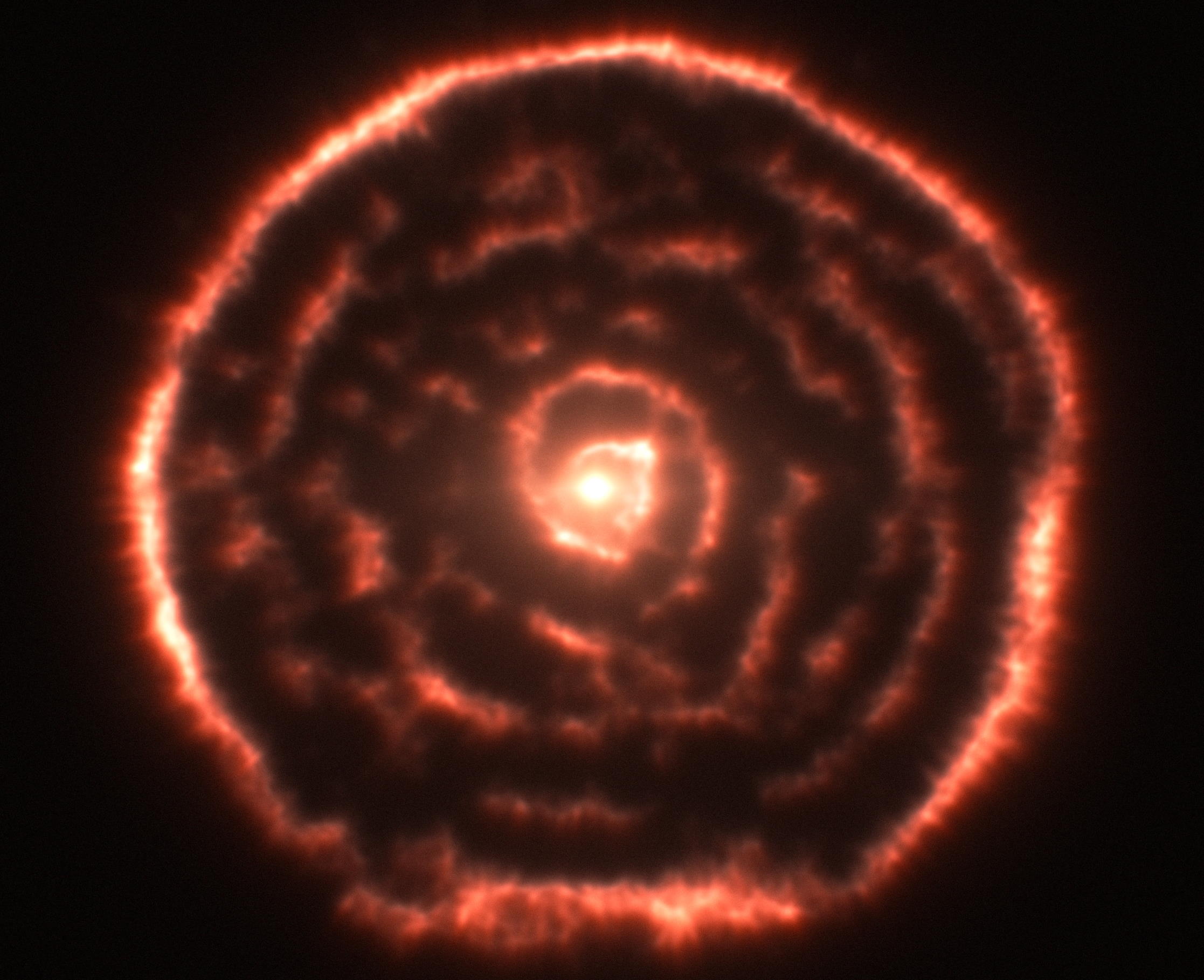
When it comes to eclipsing binaries, there’s a tremendously wide range of periods that have been observed. Some binaries eclipse one another in mere hours: periods as short as ~4-5 hours are not uncommon. On the other end, some binaries take very long periods of time: up to ~30 years or so. Very long baseline observations are needed to establish these longer-period binaries, but they do exist in non-negligible numbers.
- Some binary systems involve nearly perfectly circular orbits; others involve very eccentric, elliptical orbits.
- Some binary systems occur between stars of similar or even identical masses; others involve stars of very different masses from one another.
- And some binary systems exist with stars at similar stages of stellar evolution, such as both members being on the main sequence (in the hydrogen-burning fusion phase); others consist of a giant star orbiting a main sequence star, a variable orbiting a non-variable star, or even of a star orbiting a stellar remnant.
In general, there are three main classifications of eclipsing binary systems, but very few of them have ever been observed to possess planets.
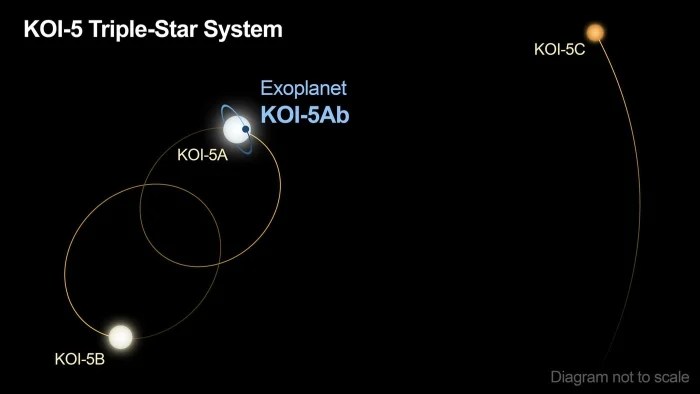
This isn’t because binary star systems (or multi-star systems with three or more stars) aren’t expected to have planets; it’s because this is not what our searches have been optimized for. But there’s a class of planets that ought to exist around at least some of these binary systems that might be:
- extraordinarily easy to find,
- could be extremely common,
- and many of which may even be inhabitable (or inhabited!) planets.
You see, when any two significant masses orbit one another, they orbit their mutual center of mass: a point known as the barycenter. For the lighter of the two masses, there are also five additional points that, if you place a mass down exactly in those five locations, the combined gravitational forces of those two masses will cause that mass to co-orbit with the same orbital period as the lighter mass without changing its relative position. Those five points — known as the Lagrange points — are of incredible interest in astrophysics.
Although the L1, L2, and L3 points are all gravitationally unstable, with objects in or around those positions requiring repeated course-corrections to remain there, L4 and L5 are gravitationally stable, and objects in or around those locations can remain there indefinitely under the right conditions.
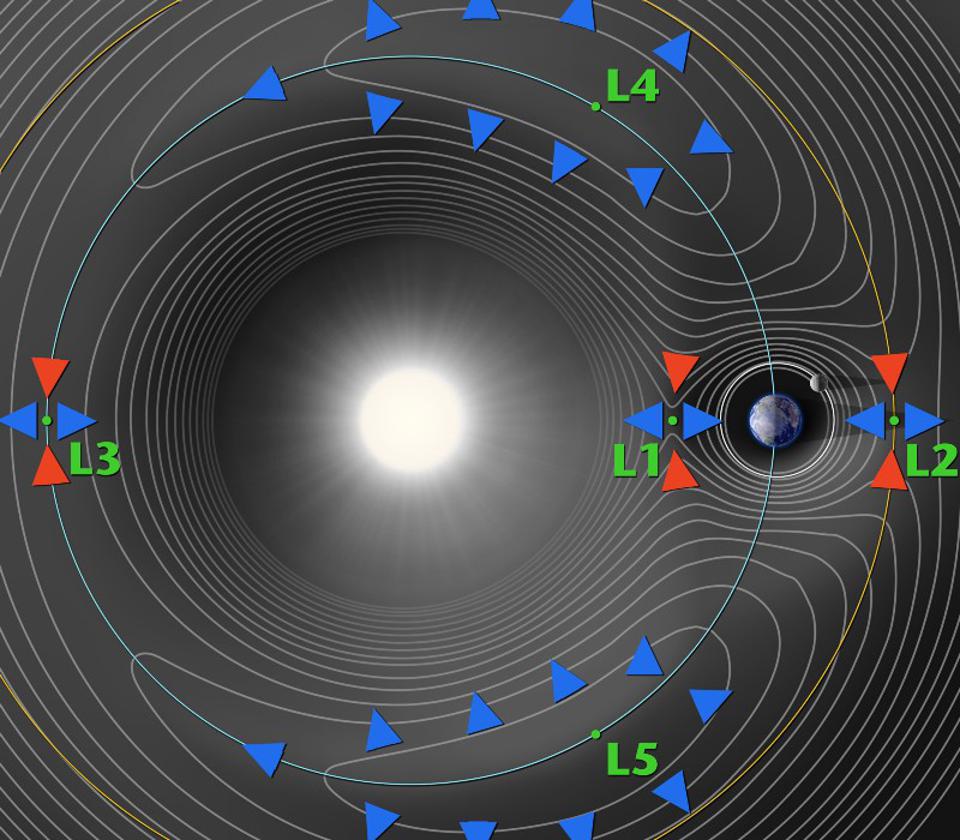
This occurs with tremendous efficiency in our own Solar System, as the giant planets, especially Jupiter, have a large collection of objects that orbit around their L4 and L5 Lagrange points. These rocky and icy bodies are known collectively as Trojans, with the “ahead” (L4) and “behind” (L5) objects respectively divided into Greek and Trojan camps. These populations of objects are typically gravitationally captured late in the Solar System’s history, well after planet formation has concluded. Some of them are transient, and will be ejected owing to gravitational interactions, but some may remain, stably or quasi-stably, for as long as the Solar System continues to exist.
The conditions for an object either at or in orbit around L4 or L5 to remain stable are simply threefold:
- The mass difference between the larger mass and the smaller mass creating the Lagrange points must be about 25:1 or greater.
- The mass of the object at or in orbit around L4/L5 must be insignificant (again, less than about 4%) of the mass of the co-orbiting body.
- And there must be no significant other masses in the system that can serve as a source of gravitational instability.
So long as these conditions are met, there should be five Lagrange points — two stable ones and three unstable ones — around the lower-mass object with respect to the higher-mass ones.
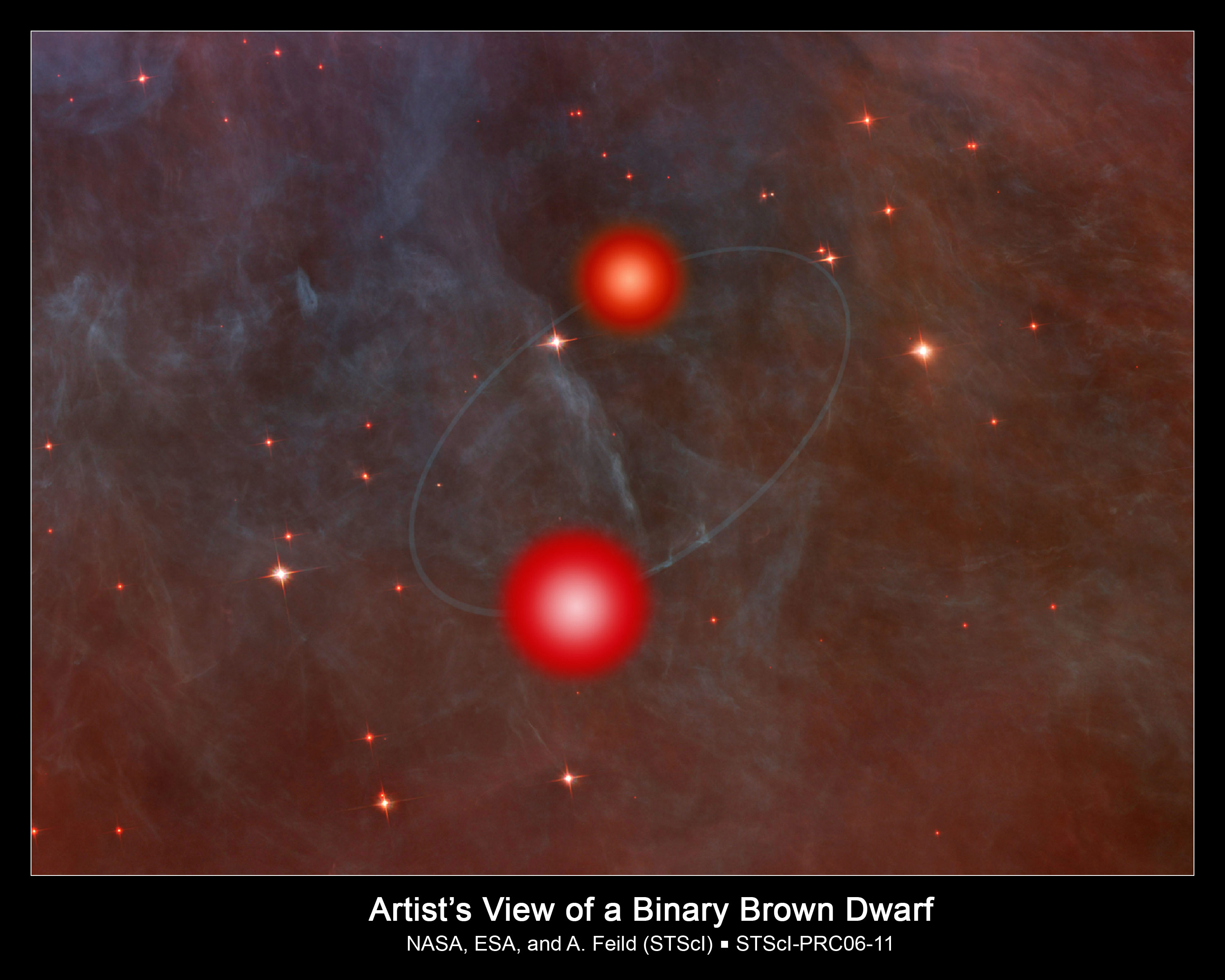
When it comes to binary stars, although most of them tend to form with comparable masses for the two stars, especially for the brighter, more luminous pairs, there are plenty of examples of mismatched binaries. The wider (i.e., greater in separation distance) the system is, and the greater the mass difference is, then the more stable the L4 and L5 points will be. This can be true, over billion+ year timescales, even for systems that don’t attain that critical 25:1 ratio or that have significant other masses in the system; each particular configuration must be worked out quantitatively to determine the specific level and timescale of instability.
But for eclipsing binary systems that do meet the proper stability criteria, a fascinating possibility emerges. Not only might there be a swarm of objects around the L4 and L5 points — representing a cloud-like distribution that could block part of the light from the larger-mass member of the binary system during part of the orbit — but there’s a real possibility, particularly for well-separated binaries of substantial mass differences, that true Lagrange planets exist. If the initial binary protostars formed with circumstellar disks around them with large separations and mass ratios, planet-formation could have funneled mass into the L4 and L5 points.
This would lead to massive exoplanets located at the Lagrange-points of the lower-mass star, and if our binary system is a sufficiently well-aligned eclipsing binary, these Lagrange exoplanets could transit across the higher-mass star with each and every orbit.
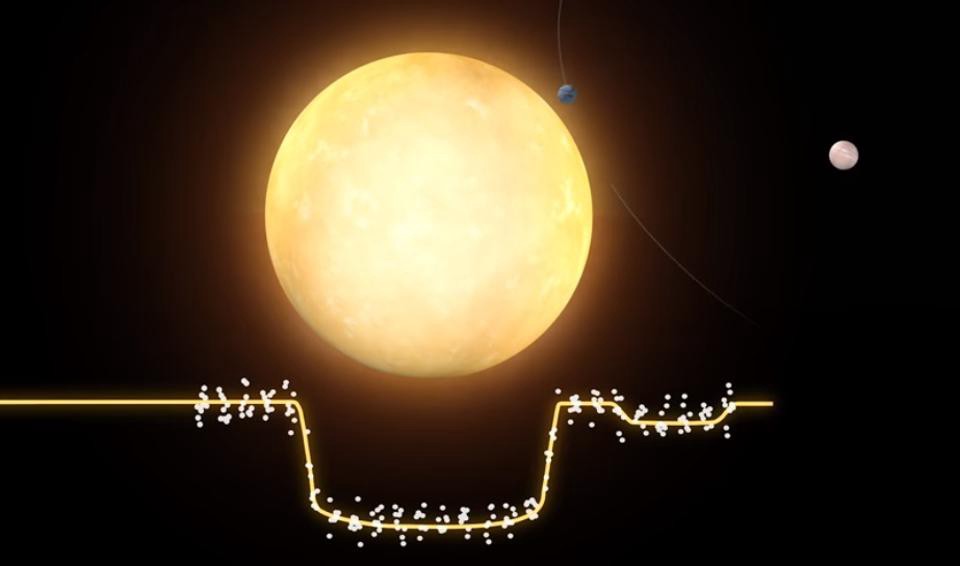
It’s important to remember, in our searches for exoplanets, that it truly is a numbers game. There are an estimated 400 billion stars within our own Milky Way, and 50% of those stars are members of multi-star systems. Sure, many of them will be in tight orbits, and many of them will have near-equal masses for the multiple members. But some fraction of these ~200 billion stars will:
- be in wide orbits,
- have large mass differences,
- and will have their L4/L5 points be gravitationally stable over multi-billion year timescales.
For these such systems, they’ll make excellent candidates to have not only a swarm of objects around the L4 and L5 Lagrange points, but they may even possess planets precisely located at L4 and L5.
If these systems are properly aligned with our line-of-sight, just as we observe eclipsing binaries, we can also observe the transits of these Lagrange-point exoplanets. Remarkably, it isn’t just the very massive, short-lived stars that could have a lower-mass binary companion that could meet this criteria; a star as low in mass as ~2 solar masses could have a red dwarf companion with perfectly stable L4 and L5 Lagrange points. We’ve never even looked for exoplanets around systems such as these, yet with the right orbital parameters, Lagrange-point exoplanets could even be rocky and habitable. Perhaps it’s time to expand our search, as we cannot know for certain what is out there unless we look.
The author acknowledges Dr. Jessie Christiansen and PhD candidate Eliot Vrijmoet for useful correspondence concerning this topic.