NASA’s big mistake: LIGO’s merging black holes were invisible after all
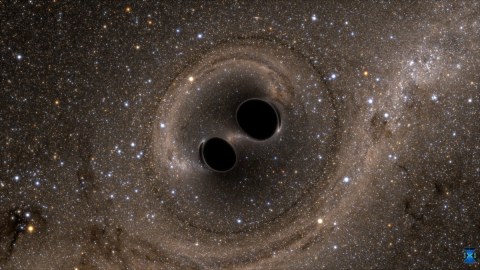
The gravitational waves were real. But earlier announcements that X-rays and gamma-rays were detected, too? Not so much.
“What’s really exciting is what comes next. I think we’re opening a window on the universe — a window of gravitational wave astronomy.” –Dave Reitze
On September 14, 2015, a tiny effect lasting 200 milliseconds passed through the Earth at the speed of light. The entire planet compressed and expanded in two mutually perpendicular directions by less than the width of a proton, oscillating back and forth roughly seven times in that span. And in two detectors separated by 2,000 miles, an interference pattern formed by two isolated lasers, reflected back-and-forth in a vacuum and then brought together again, gave us the telltale explanation for this effect. From 1.3 billion light years away, two black holes some 30 times the mass of the Sun had spiraled into one another, merging together and sending energetic ripples through the fabric of space itself. For the first time, a gravitational wave — one of the oldest unverified predictions of Einstein’s General Relativity — had been directly detected.
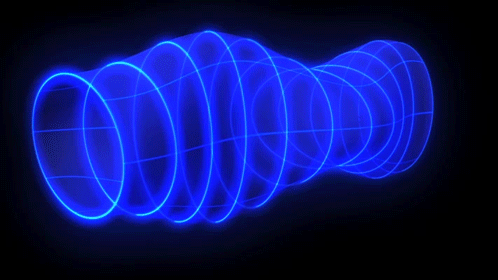
Optical telescopes didn’t see anything, as expected. Merging black holes weren’t anticipated to emit any light, unlike merging stars (which create a larger star), white dwarfs (which create a supernova), or neutron stars (which are thought to create a gamma ray burst); they should only be detectable by their gravitational wave signal. Yet there was a curious possible exception, as a team from NASA’s Fermi satellite claimed to detect gamma rays coincident with this event, offset by a meagre 0.4 seconds. An array of 14 crystal detectors on board — the Gamma-ray Burst detection Monitor (GBM) instrument — detected an unexpected burst of X-rays, and claimed there was only a 0.2% chance of a false positive.
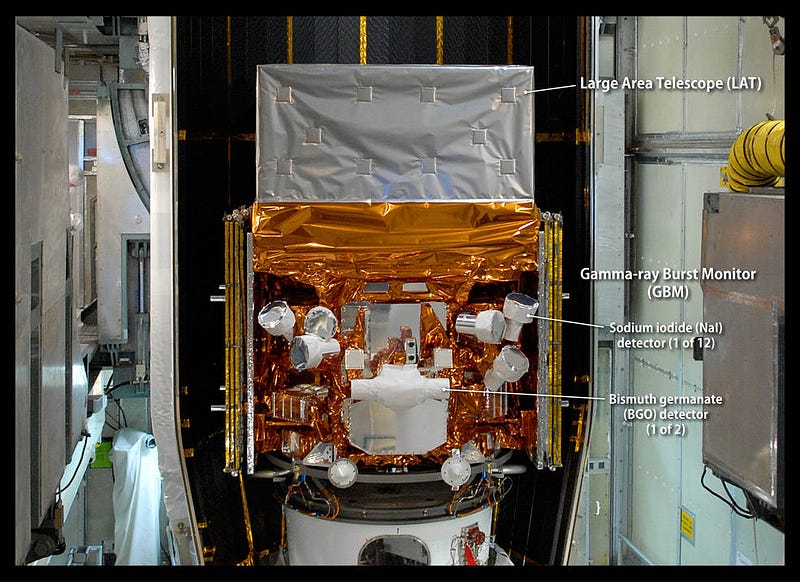
While NASA was celebrating, however, cautious scientists all over the world were skeptical. Not only would this overthrow the leading theoretical models for black hole mergers, and not only does a 99.8% chance of success correspond only to a 3-σ significance (rather than the 5-σ significance typically required for a discovery in physics), but a complimentary satellite in orbit — the ESA’s INTEGRAL satellite — failed to see the corroborating evidence it should have if this signal were real. On the contrary, INTEGRAL searched through all the data and failed to find any interesting signal coincident with LIGO’s gravitational wave at all. Far from a definitive detection, this conflicting data raised more questions than it answered.
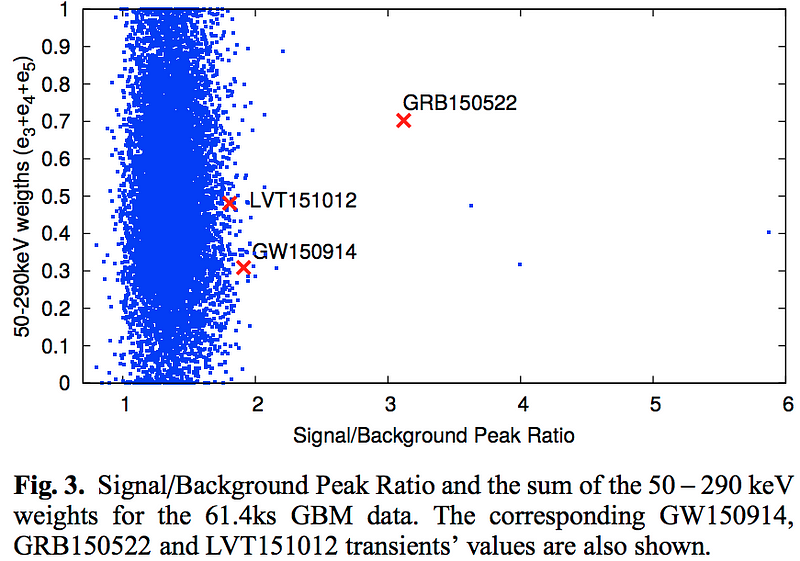
Thanks to a new paper now available from J. Greiner, J.M. Burgess, V. Savchenko and H.-F. Yu, however, the apparent conflict may at last be resolved. The secret lies in understanding how the GBM instrument aboard NASA’s Fermi satellite actually works. Rather than measuring an absolute signal, it measures a steady, continuous background of photons over a large energy range. The spikes above that background, when they appear, can show us either a real, physical event (like a burst or merger), or they can simply be evidence of a random fluctuation that has no physical origin at all. If you use an imperfect algorithm for discriminating which fluctuations are physical vs. non-physical, you could wind up drawing invalid conclusions about what’s real and what’s phantasmal. The huge advance of the new paper, submitted to the Astrophysical Journal as a Letter, isn’t observational or theoretical, but rather statistical; it more robustly and successfully discriminates between normal noise and a burst of high-energy light from an astrophysical source.
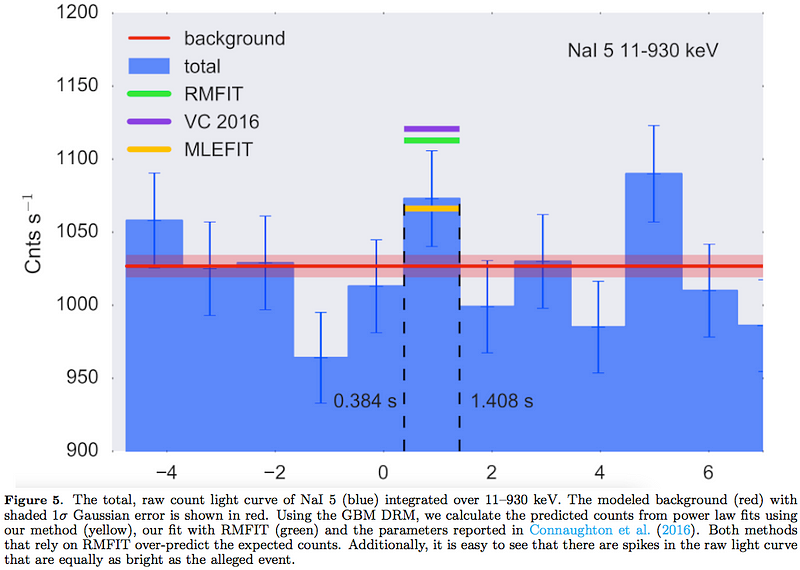
Above, you can see a number of different ways of reconstructing the apparent signal coincident with LIGO’s gravitational wave. The original Fermi team’s analysis is shown in purple: a clear detection. However, the superior reconstruction of this new paper is shown in orange, and lines up with both the raw data (blue) and also — more importantly — is consistent with a non-detection, meaning that there is no electromagnetic signal here. According to one of the paper’s authors, J. Michael Burgess, the original paper (claiming a detection) had some statistical flaws his team was able to spot, relating the following:
When I saw the announcement and the paper, the spectrum looked like what I always see as background.
After pulling his team together and developing some new analysis tools, they confirmed their suspicions:
We instantly saw that we got a much different answer. The spectrum of the event was basically zero: nothing there.
The new statistical technique developed by Burgess and his collaborators has proven to be incredibly powerful, successfully pulling out even faint gamma ray signals from noisy data and drastically reducing the number of false positives. By combining this new technique with the existing Fermi data, it should be possible to make huge strides forward in identifying true astrophysical events.
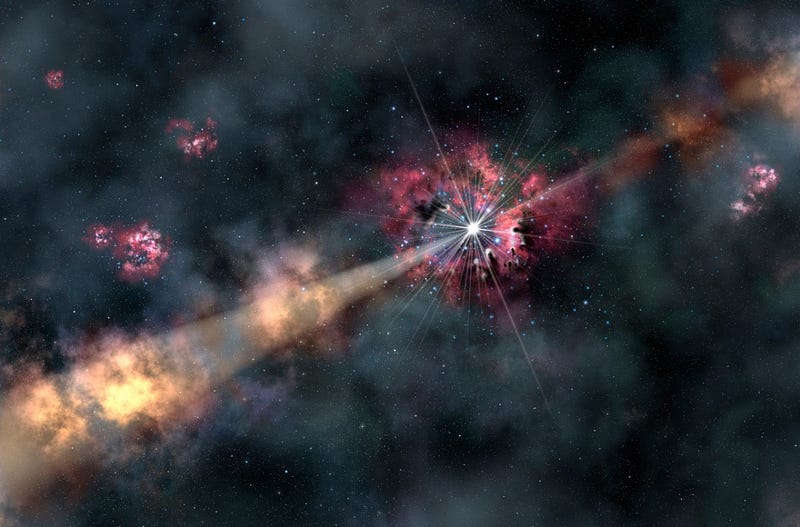
It’s important to remember that there can and will be correlations in the future not only between gravitational waves and gamma rays, but between LIGO and Fermi’s GBM instrument. When asked for comment, Burgess said the following:
GBM is an amazing instrument and its synergy with LIGO provides an amazing way for us to view the Universe. The GBM team has made a huge effort for this, and when a neutron star merger happens nearby, it is very likely GBM and LIGO (and others) will see something… and this will be amazing!
But in order to make sure we aren’t fooling ourselves, we have to do it right. Collaboration between the teams — the Fermi team, the INTEGRAL team, and the gravitational wave teams — are incredibly important. But the necessity of calibrating the signals that multiple observatories will see is essential to getting the right results. Merging black holes may, in fact, sometimes lead to electromagnetic radiation, a possibility which future events will hopefully test. But the golden rule in situations like these is the null hypothesis: in the absence of extraordinary evidence, as is the case here, bet on exactly what the leading physics ideas predict.
This post first appeared at Forbes, and is brought to you ad-free by our Patreon supporters. Comment on our forum, & buy our first book: Beyond The Galaxy!