JWST’s “most distant galaxies” might be fooling us all
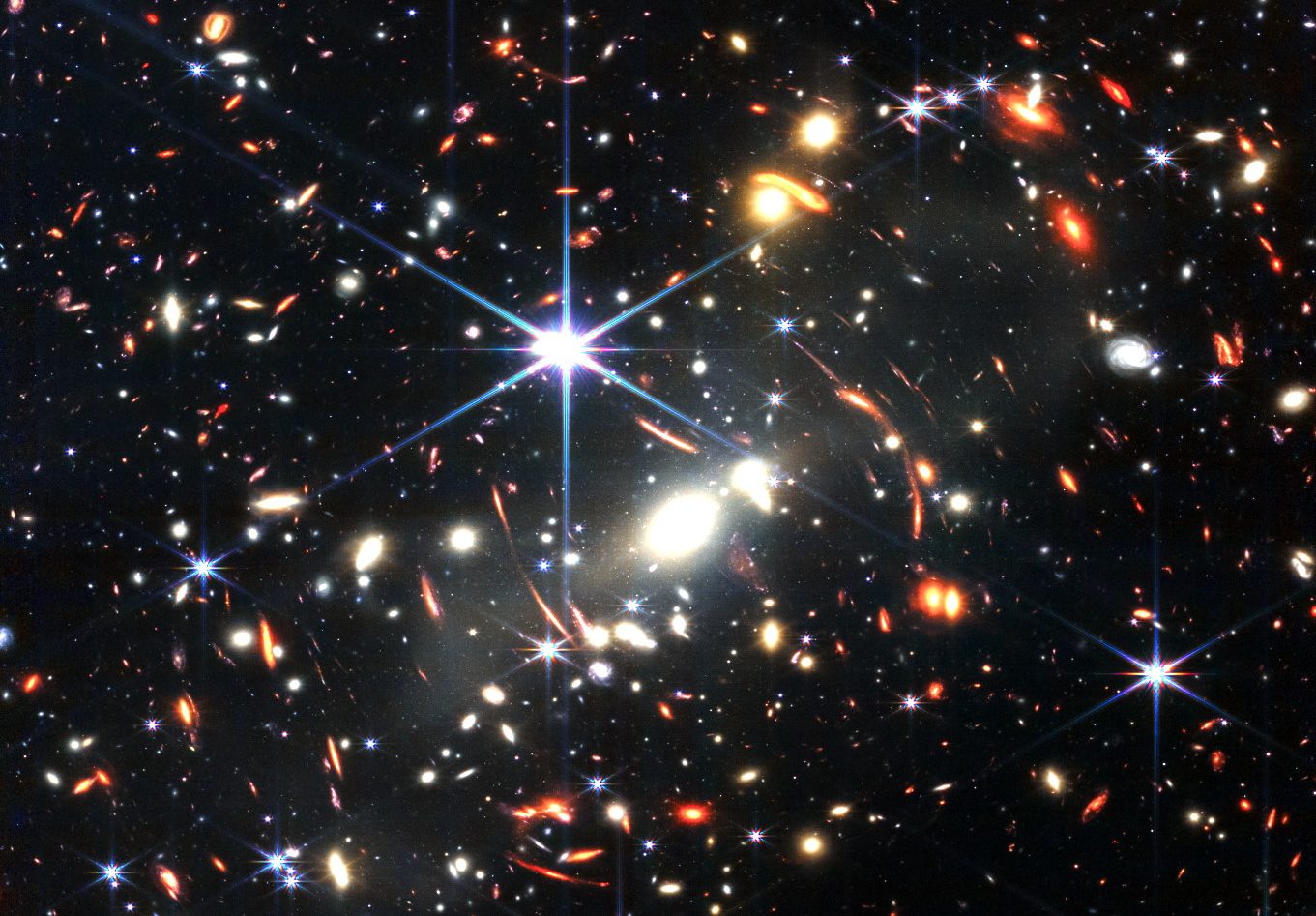
- In late 2022, despite only being operating for a few months, JWST broke Hubble’s all-time record for the most distant galaxy ever observed.
- In its very first deep-field image, in fact, there were a total of 87 “candidate ultra-distant galaxies” identified in JWST’s one viewing of galaxy cluster SMACS 0723.
- But there’s an excellent chance that many of those candidates, perhaps even most or almost all of them, aren’t actually ultra-distant at all.
Somewhere out there, in the distant recesses of the expanding Universe, is the farthest galaxy we’re capable of seeing. The farther away an object is, the more time it takes light to travel through the Universe to reach us. As we look to greater and greater distances, we’re seeing objects as they were farther and farther back in time: closer back toward the start of the hot Big Bang. The Universe, because it was born hot, dense, and relatively uniform, requires lots of time — hundreds of millions of years, at least — for those first galaxies to form; beyond that, there’s nothing to see.
We’ve known there needed to be galaxies out there beyond the limits of what Hubble was capable of seeing, and the JWST was designed with precisely the specifications needed to find what Hubble cannot. Even in the very first science image released by JWST scientists, showcasing the gravitationally lensed galaxy cluster SMACS 0723, a large number of objects that had all the properties an ultra-distant one would have were identified, despite taking up just a tiny region of the sky. If all of these ultra-distant galaxy candidates were real, we’d have too many of them too early, forcing us to rethink how galaxies begin forming within the Universe. But we might be fooling ourselves completely, and we won’t know for sure with only our current data. Here’s why.
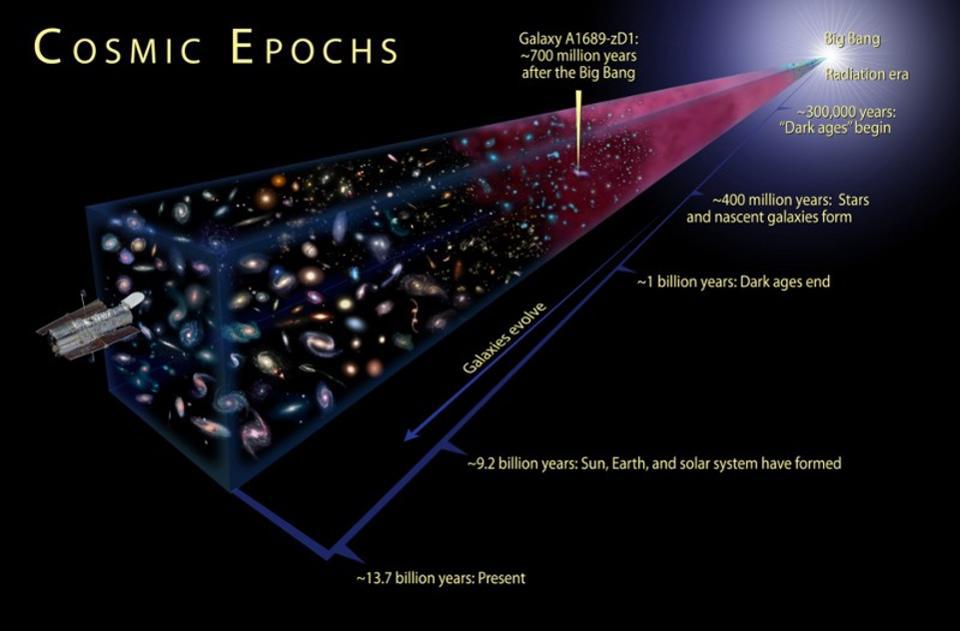
We know, observationally, that there were no stars or galaxies shortly after the Big Bang. We also know, observationally, that at the observational limits of Hubble — taking us back 13.4 billion years in time, to objects that existed just ~400 million years after the Big Bang — galaxies are already massive, rich in structure, and evolved in terms of the elements that exist within them. Somehow, we have to go from a Universe that was born almost perfectly uniform, with the densest regions just a few parts in 100,000 denser than average, to one that’s rich in evolved, massive galaxies in only a few hundred million years.
Unfortunately, we can’t simply look for the light that those distant galaxies emit. There’s a tremendous difference between the light that a distant galaxy emits and the light that arrives at our eyes after journeying for billions of light-years across the Universe. That initially emitted light gets affected by everything that interacts with it along its journey, including:
- light-blocking neutral matter,
- hot gas and plasma that scatters and disperses that light,
- growing and shrinking clumps of matter that change the gravitational potential in the region where the light is propagating,
- and the expansion of the Universe, that stretches the wavelength of any light that travels through it.
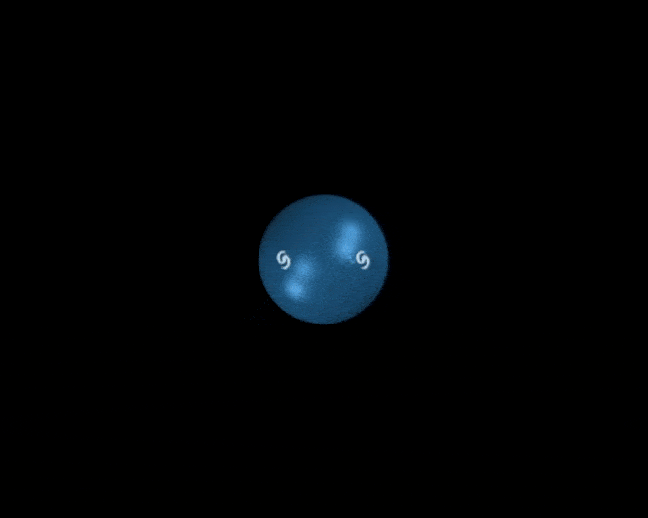
Even though the laws of physics — from the quantum physics that governs electrons, atoms, and ions to the thermal and stellar physics that governs stars and galaxies — are the same everywhere throughout the Universe, objects at different distances won’t appear the same way when you observe them. The environments they’re in, as well as the environments they must pass through on their way to our eyes and instruments, alter that light irrevocably. If we want to understand and discover what’s out there, we need to be able to not only observe the most distant light possible, but to reconstruct what that light was like when it was first emitted so long ago.
One of the most suggestive hints you can see that might make you suspect you’re seeing something from long ago and far away is simply based on the color of what you’re looking at. Stars, by and large, emit light from the ultraviolet through the visible and into the infrared portion of the spectrum. When you see an object that’s redder in color than the typical, nearby objects we observe in our vicinity, there are many possible reasons why it might appear red. It could be full of intrinsically red stars. It could be extremely dusty, where light-blocking material obscures the shorter-wavelength light. But one fascinating possibility that must be considered is that it’s red because the expansion of the Universe shifted that light, emitted at much shorter wavelengths, to the long wavelengths we now observe.
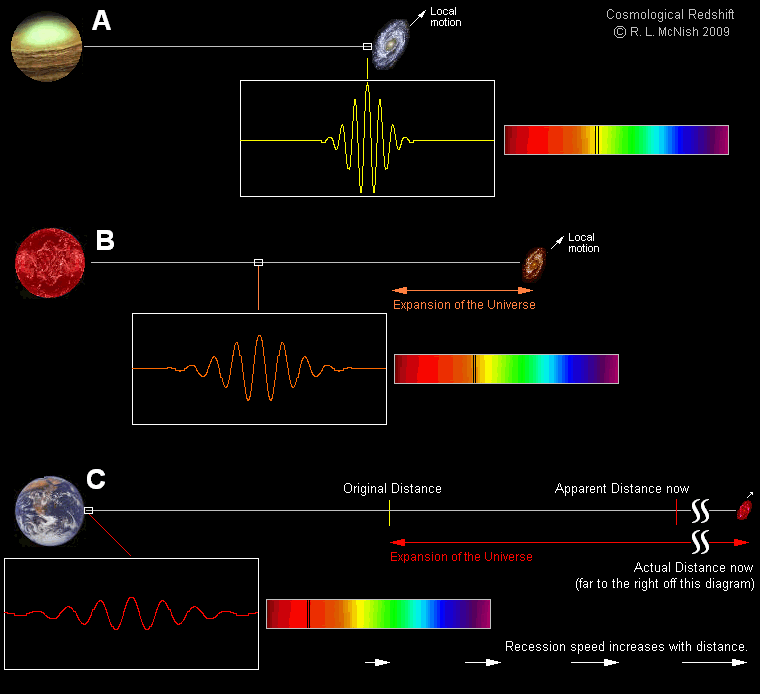
One of the keys to unlocking our understanding of our cosmos, as well as our place within it, came about in the 20th century when we discovered the expansion of the Universe. The fabric of space itself is like a ball of leavening dough, and the galaxies within it are like raisins sprinkled throughout it. As dough leavens, it expands, and all of the raisins mutually get farther apart from one another. From the perspective of any individual raisin — or of any observer located within a galaxy — the other raisins (galaxies) move away from it, with more distant raisins (galaxies) receding more quickly, and the light traveling from one to the other experiencing a greater shift in its wavelength than the ones found more nearby.
You can’t simply detect light of any arbitrary wavelength with any old telescope, detector, or observatory. Longer, redder wavelength light corresponds to lower energies and cooler temperatures, and if you want to detect it, your telescope and its instruments must be cold enough so that the low-energy light you’re seeking to detect is the signal that can rise above all forms of noise that would be present. While Hubble can see light out to a wavelength of about 1.5 microns, JWST is cold enough to see light up to ~20 times longer in wavelength: all the way out to ~30 microns in wavelength. Only because of its cold, cryogenic, pristine properties can it see the reddest, most distant objects of all.

It should come as no surprise to anyone that, even in its very first science observation that was released, the JWST found a large number of extremely red objects. But just because you see something that’s red doesn’t mean it’s an ultra-distant galaxy. There are many signals that can fool you:
- galaxies where all of the hot, blue, massive stars have died, but the redder stars remain,
- galaxies that are rich in dust grains of small, common sizes, which are efficient at blocking the bluer light but are transparent to redder light,
- or galaxies that exist along a line-of-sight that scatters away or blocks the bluer wavelengths of light passing through them, while leaving the red ones behind.
This is the problem with the most basic of astronomical techniques that allow you to measure the color of an object or set of objects: photometry. Just as humans have three types of cones in our eyes — sensitive to red, green, and blue — our telescopes have multiple filters on them, sensitive to different wavelength ranges of light. When you see that the shorter wavelength ranges show no light, and then longer wavelength ranges beyond a certain threshold show lots of light, you’ve got an excellent candidate for an ultra-distant galaxy.
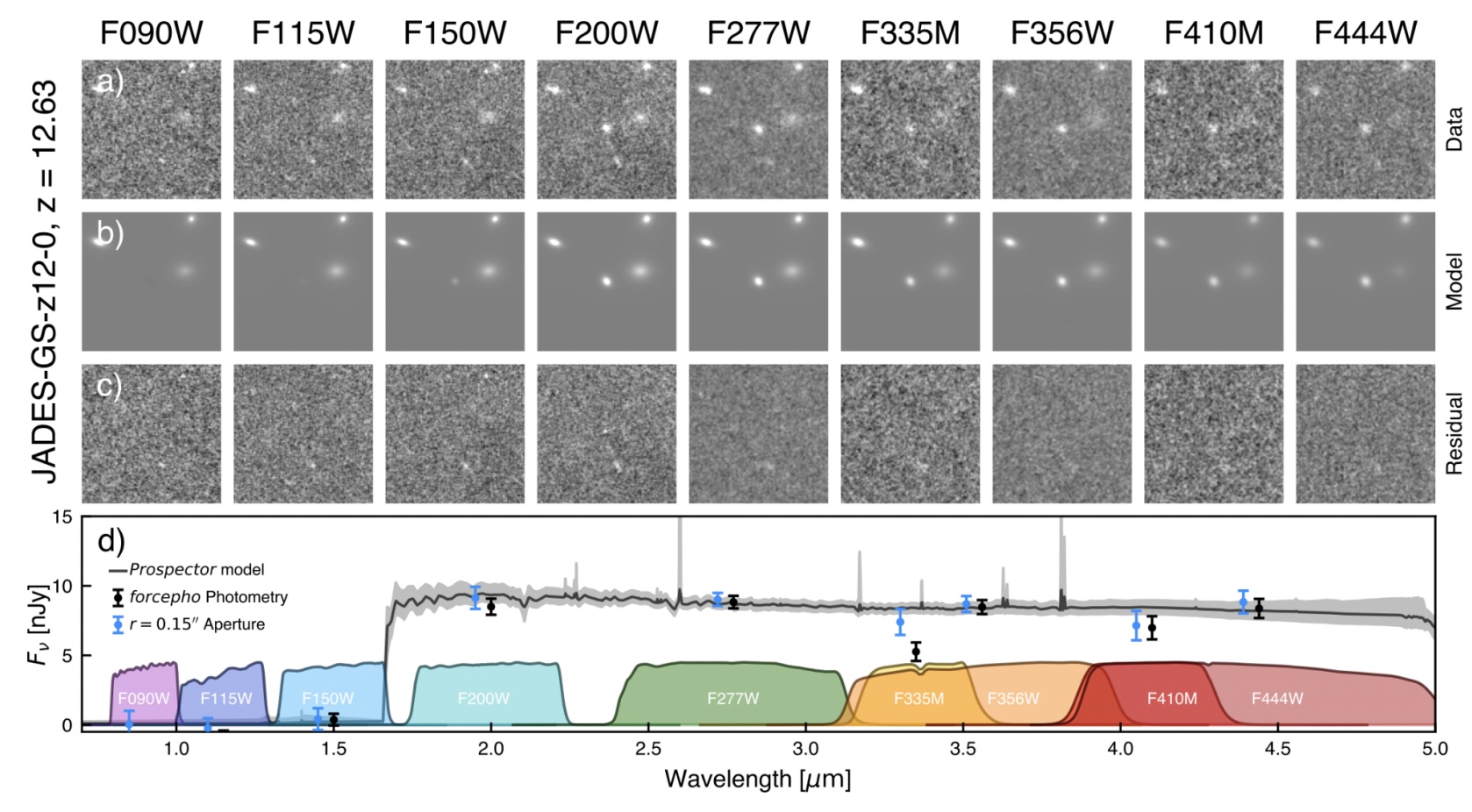
But there’s a reason we only call such an object a “candidate” ultra-distant galaxy: sure, it’s red, and it’s suggestive of the idea that we might be seeing extremely redshifted light, but we need to confirm that idea with superior, unambiguous data.
How do you confirm the distance to an object whose light appears extremely red?
That’s where the technique of spectroscopy comes into play. Spectroscopy is much finer than photometry; instead of a few wide “bins” that span a variety of wavelengths, we break the light up into incredibly fine components, enabling us to discern differences in flux over tiny fractions of an ångström. In particular, we look for a feature known as a Lyman break: corresponding to the most powerful atomic transition of hydrogen: from the 2nd-lowest energy level down to the ground state. We know that always occurs at the same wavelength: 121.5 nanometers. If we can measure that feature and measure the observed wavelength at which it appears, we can just do a little math to determine, unambiguously, the unique and intrinsic redshift of the distant object in question.
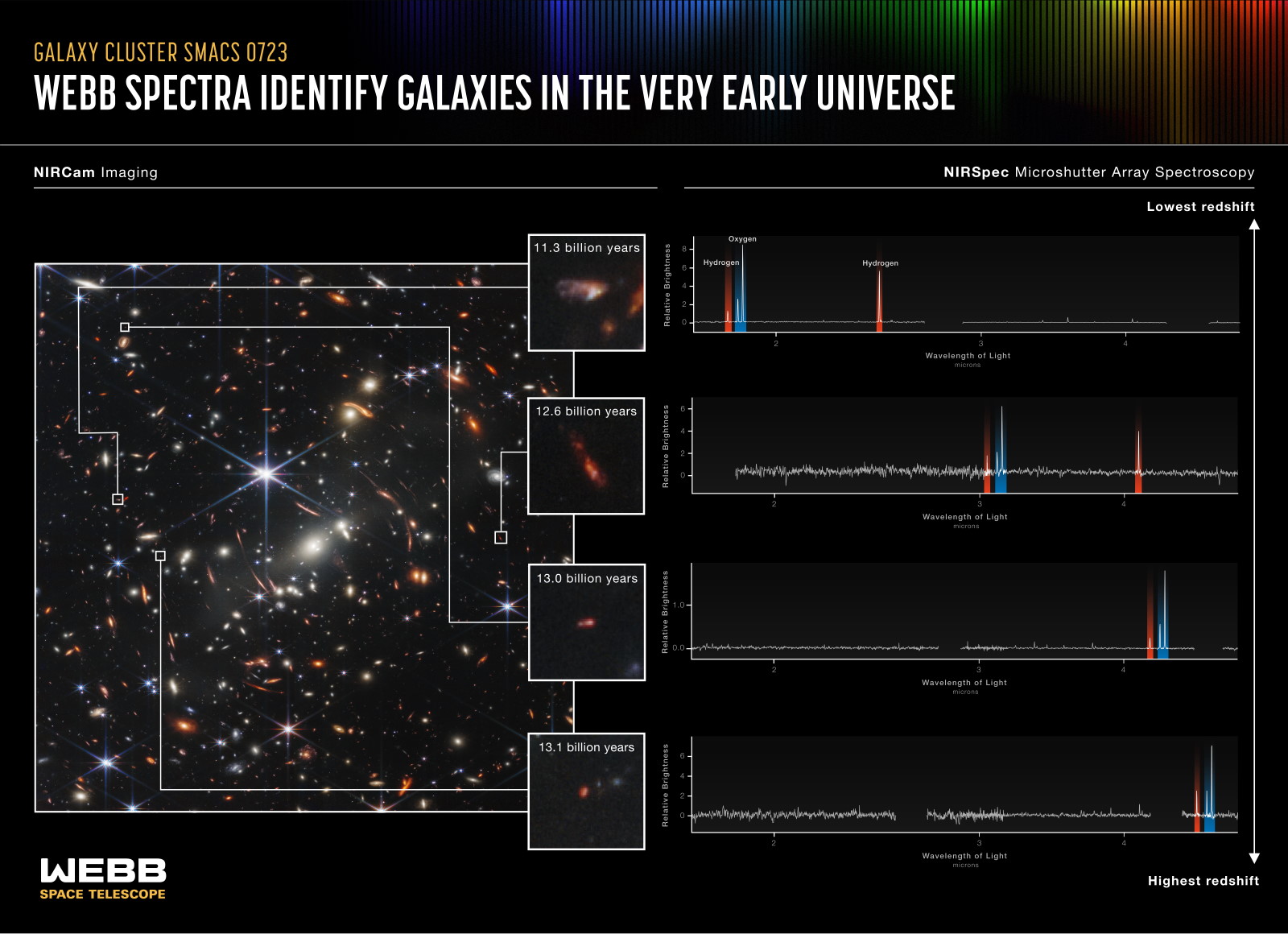
The very first science image ever released by the JWST team, of galaxy cluster SMACS 0723, went extremely deep, observing the same region of sky in many different photometric filters for long periods of time. In that data set, there were many objects with a variety of properties, almost all of which were galaxies from the distant Universe. But among those objects, there were a number that stood out from the rest. In particular, 87 of those points of light were seen to be extraordinarily red, with no light at all visible in the shortest-wavelength JWST photometric filters. This is why they’re treated as candidate ultra-distant galaxies.
But being a candidate is only part of the game; you have to gather the critical, spectroscopic data if you want to answer the all-important question of, “How many of them are real?” In other words, how many of them aren’t just “candidates” for being ultra-distant galaxies but are actually ultra-distant galaxies, rather than impostor objects that exist at lower redshifts? Is it all of them? Most of them? Some of them? Or only a few?
At this point in time, for the 87 ultra-distant galaxy candidates in the field of JWST’s view of the SMACS 0723 galaxy cluster, only one of them has been observed spectroscopically: it’s distant, at a redshift of 8.6 (corresponding to an age of the Universe of ~560 million years at the time), but it’s not the ultra-distant galaxy we were hoping for.
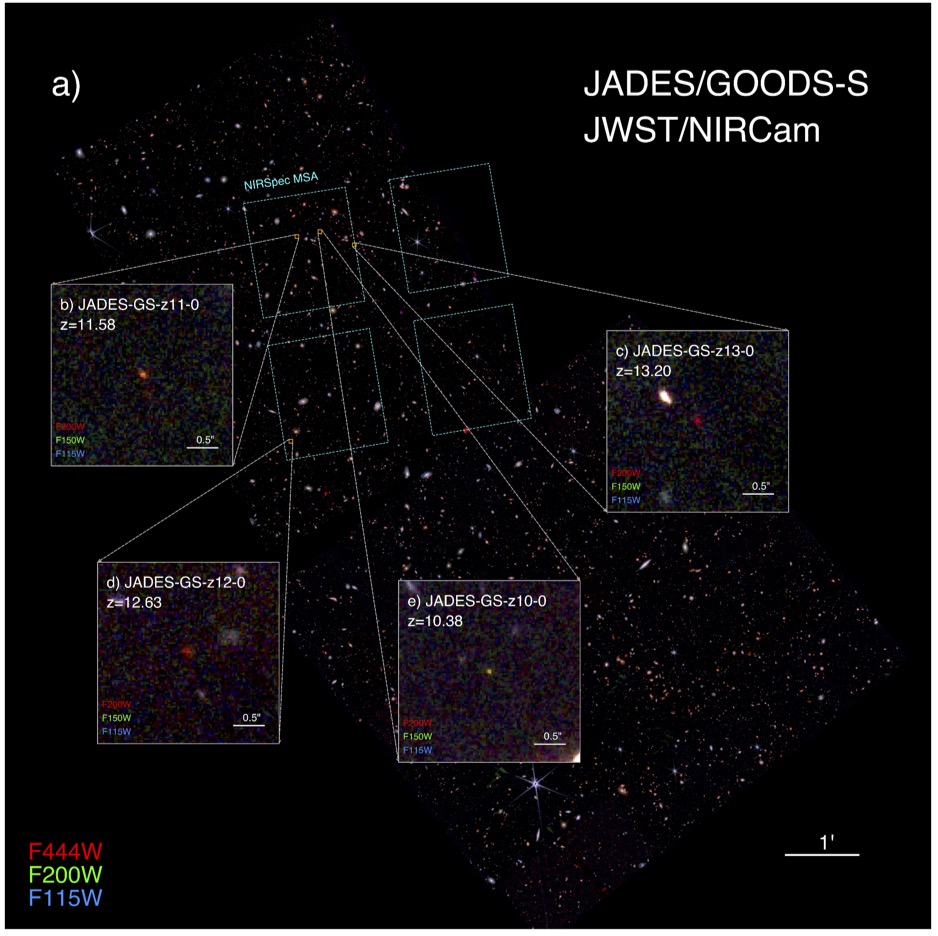
Fortunately, there is a JWST survey that does have both photometric and spectroscopic data already in hand: JADES. Standing for the JWST Advanced Deep Extragalactic Survey, JADES takes a region of space already observed at high resolution, in many filters, and over long periods of time by Hubble, and then added a layer of JWST photometric data atop it. By using both Hubble and JWST photometric data combined, they identified a series of potentially ultra-distant galaxy candidates. The exact number has not been published, but we know there were tens of candidates that were considered for follow-up observations.
The photometric data was then followed up with spectroscopy using JWST’s NIRSpec instrument. Although we have no way of knowing, at present, how many of those candidate galaxies were determined to simply be interlopers, we know that four galaxies from that sample were identified to be robustly at ultra-high distances. Two were candidates identified from Hubble data; two were candidates identified by JWST data. But all four are from extremely early times, when the Universe was less than half-a-billion years old; all four show that exquisite Lyman break feature; and the most distant is at a redshift of 13.2, whose light was emitted just 320 million years after the Big Bang: when the Universe was just 2.3% of its current age.
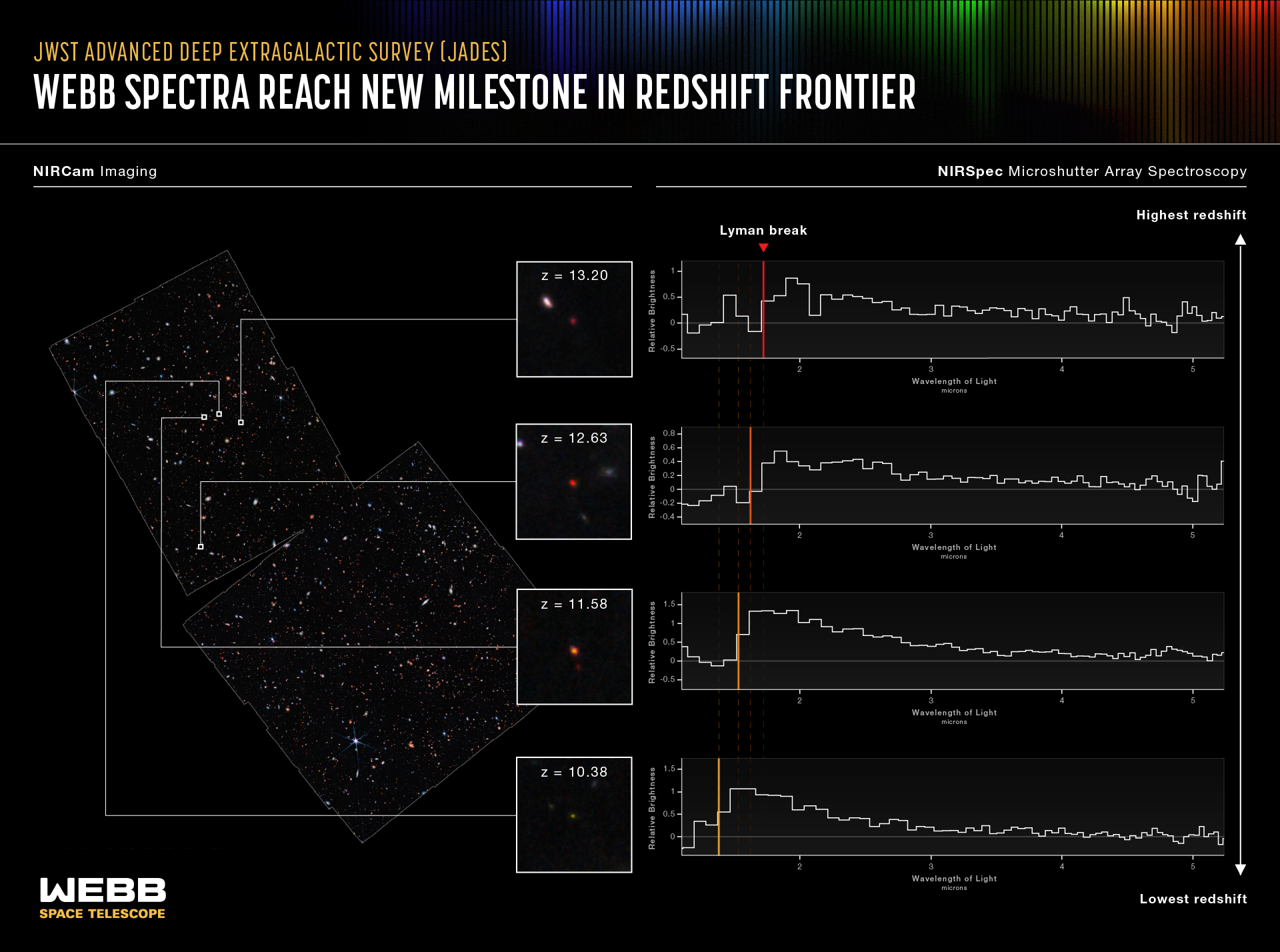
If all 87 of the ultra-distant galaxy candidates found in the field of SMACS 0723 turned out to actually be ultra-distant galaxies — if they later turn out to be spectroscopically confirmed — then this observation poses a significant problem for the standard picture of how cosmic structure forms in the Universe. There simply shouldn’t be such a large number of bright, massive, and already-evolved galaxies at this early stage in cosmic history.
In research presented at the 241st meeting of the American Astronomical Society, Professor Haojing Yan made a strong case that many of these galaxies likely were ultra-distant objects, and that astronomers and astrophysicists might be forced to rethink the early birth, growth, and evolution of galaxies if that’s the case. He was so confident in the quality of the photometric data and what it suggested, that he was willing to bet a very large beer that more than 50% of these galaxy candidates would wind up being spectroscopically confirmed, and that our ideas about the population, abundance, and properties of these many galaxies would demand a cosmic rethink of how they formed so early on.
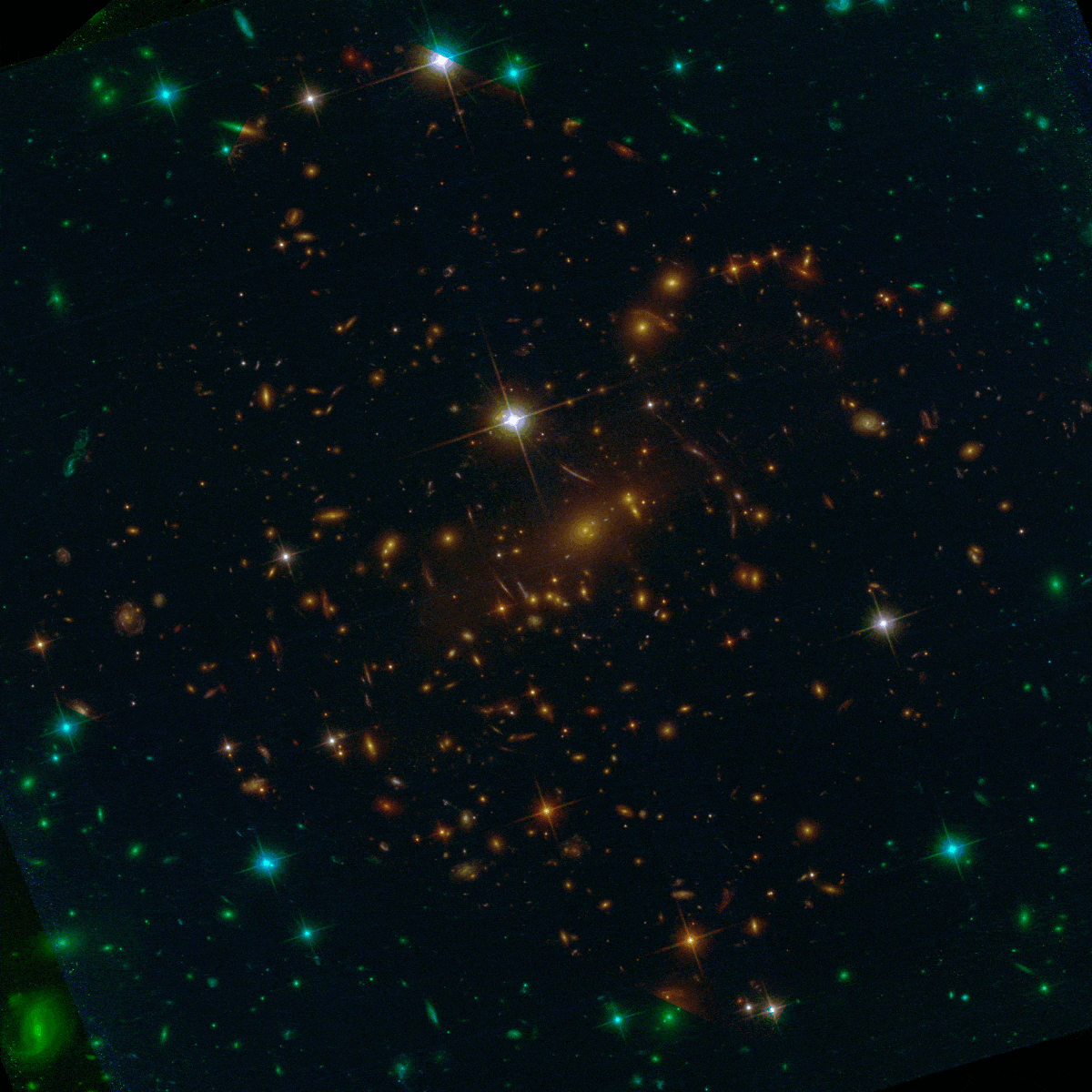
Without the critical data, all of this is simply speculation. The quest is not to determine whether someone’s hunch is correct or not, it’s to understand and measure the true nature of these objects, finding out which ones are ultra-distant galaxies, which ones are less-distant interlopers, and to understand what the false positive rate is and what determines it. But you can’t draw any definitive conclusions at all without spectroscopy; for the non-astronomers out there, you should trust a photometric measurement of redshift about as much as you trust a purported photo of the Loch Ness Monster to reveal the truth about its nature.
There are 87 candidates for being ultra-distant galaxies within the field of cluster SMACS 0723, and it’s a safe bet that some of them truly are ultra-distant galaxies. I’d even be willing to bet that at least one of those candidates is more distant than the current cosmic record holder for most distant galaxy: JADES-GS-z13-0. But without the critical spectroscopic data on these galaxies — enabling a measurement of the false positive rate from photometric candidates — we have no way of knowing whether a few of these galaxies, many of them, most of them, or even nearly all of them are less-distant impostors, tricking our inexperienced eyes into thinking they’re more distant than they are. In the meantime, as exciting as the possibility is that our cosmic story might need a rethink, we have to keep in mind that JWST’s alleged “most distant galaxies” might be fooling us all.
Note: Ethan Siegel has agreed to buy Dr. Haojing Yan at least a yard-long beer at next year’s AAS meeting, if more than 50% of the galaxy candidates put forth in his paper are spectroscopically confirmed.