Everything we now know about the Milky Way’s supermassive black hole
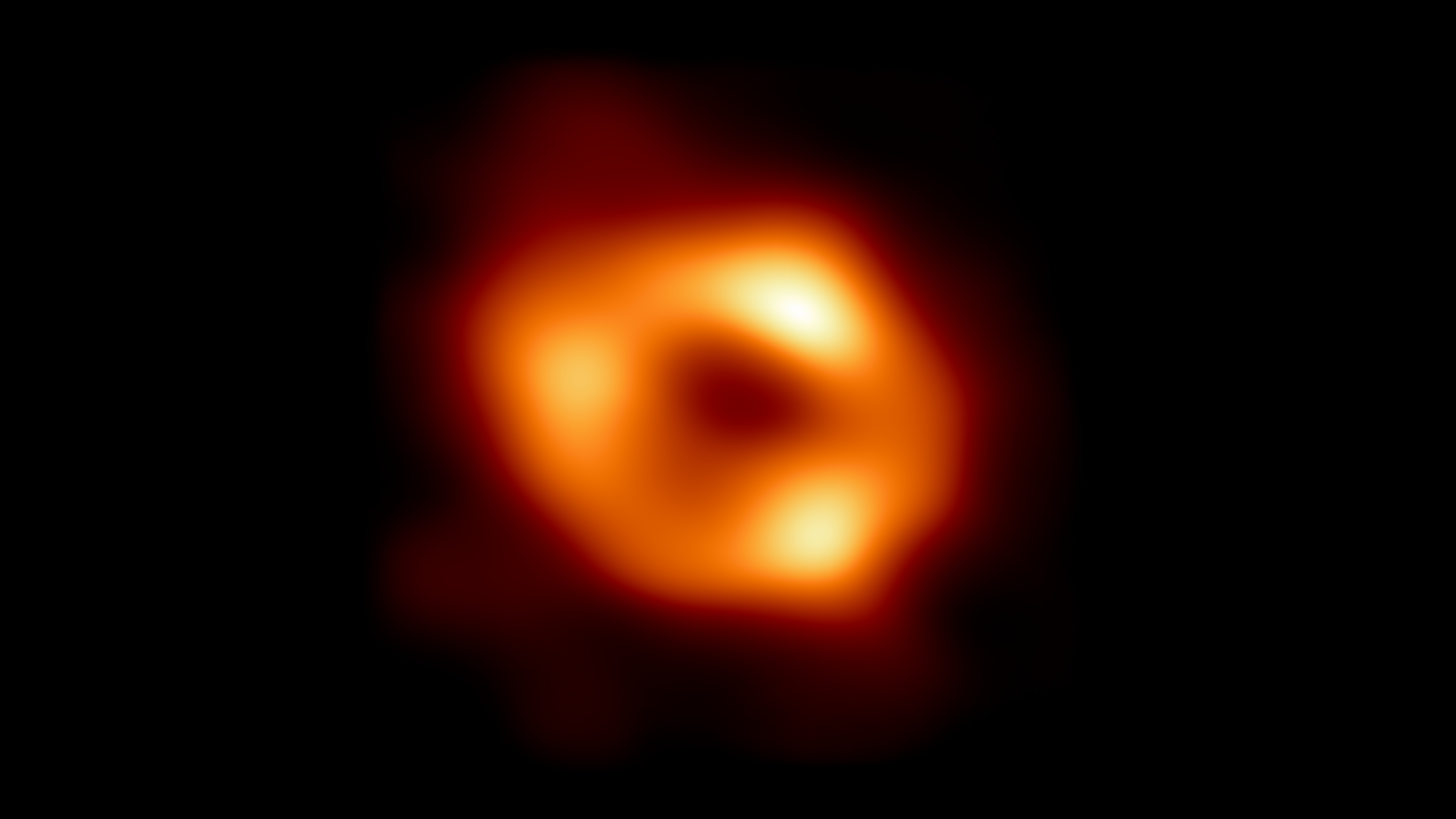
- For generations, many have suspected that the Milky Way, like all large galaxies, houses a supermassive black hole at its center.
- Indirect evidence, including X-ray flares, Fermi bubbles, and the orbital properties of stars near the galactic center have all supported its existence, but it’s never been directly imaged before.
- After taking data in 2017 from all around the world, the Event Horizon Telescope team has finally revealed our first view of Sagittarius A*: our supermassive black hole. And wow, was it worth the wait!
The moment has finally arrived. Back in 2015, the Event Horizon Telescope collaboration was formed, with the goal of using an array of radio telescopes located all around the world to directly image the event horizon of a black hole for the first time. In 2017, a series of objects were observed, all simultaneously, by that array of telescopes across the globe, collecting the data necessary for reconstructing what a black hole looks like for the very first time.
And even though the ultramassive black hole at the center of the giant elliptical galaxy, Messier 87, was released first (and years ago), that wasn’t the grand prize the Event Horizon Telescope team was seeking.
Instead, there’s a supermassive black hole right at the center of our own galaxy. Located a little under 27,000 light-years away, it’s known as Sagittarius A*, and from indirect measurements, we’ve known for decades that it weighs in at about 4 million solar masses. At its mass and distance, it should have the largest event horizon of any black hole visible from Earth. After more than 5 years of waiting to ensure they got everything right, the Event Horizon Telescope team has finally released their results of what the largest black hole in our own cosmic backyard looks like. Here’s the story of what we’re looking at, and how it came to be.
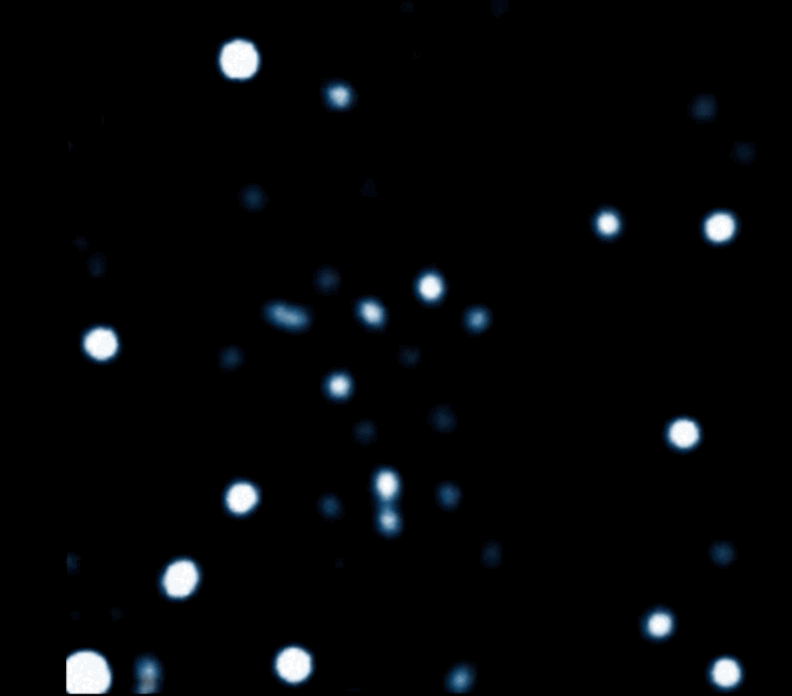
How do you image a black hole?
Black holes have this maddening property to them: they absorb all of the matter and radiation that gets too close to them, while simultaneously not emitting any light of their own. And yet, nevertheless, it’s possible to image them directly.
The key that makes it possible, believe it or not, is simply the black hole’s gravity.
We normally think of gravity the same way Newton did: as an invisible, attractive force between massive objects. Einstein taught us to think a little bit differently, however; the theory of General Relativity dictates that the presence of matter and energy curves the fabric of space — and curves it more severely close to a large mass than Newton ever predicted — and then that curved space dictates how everything, including not just massive objects but massless ones as well, moves through it.
When matter enters the vicinity of a black hole, it experiences not just the attractive gravitational force, but also tremendous tidal gravitational forces. In other words, any part of an infalling object that’s closer to the black hole’s center than another part will experience a different, stronger gravitational force than the part that’s farther away, and this differential force will rip the infalling object apart. In the end, that matter doesn’t just get sucked into the black hole, but gets stretched into a disk and a set of streams that accrete around and orbit the central black hole.
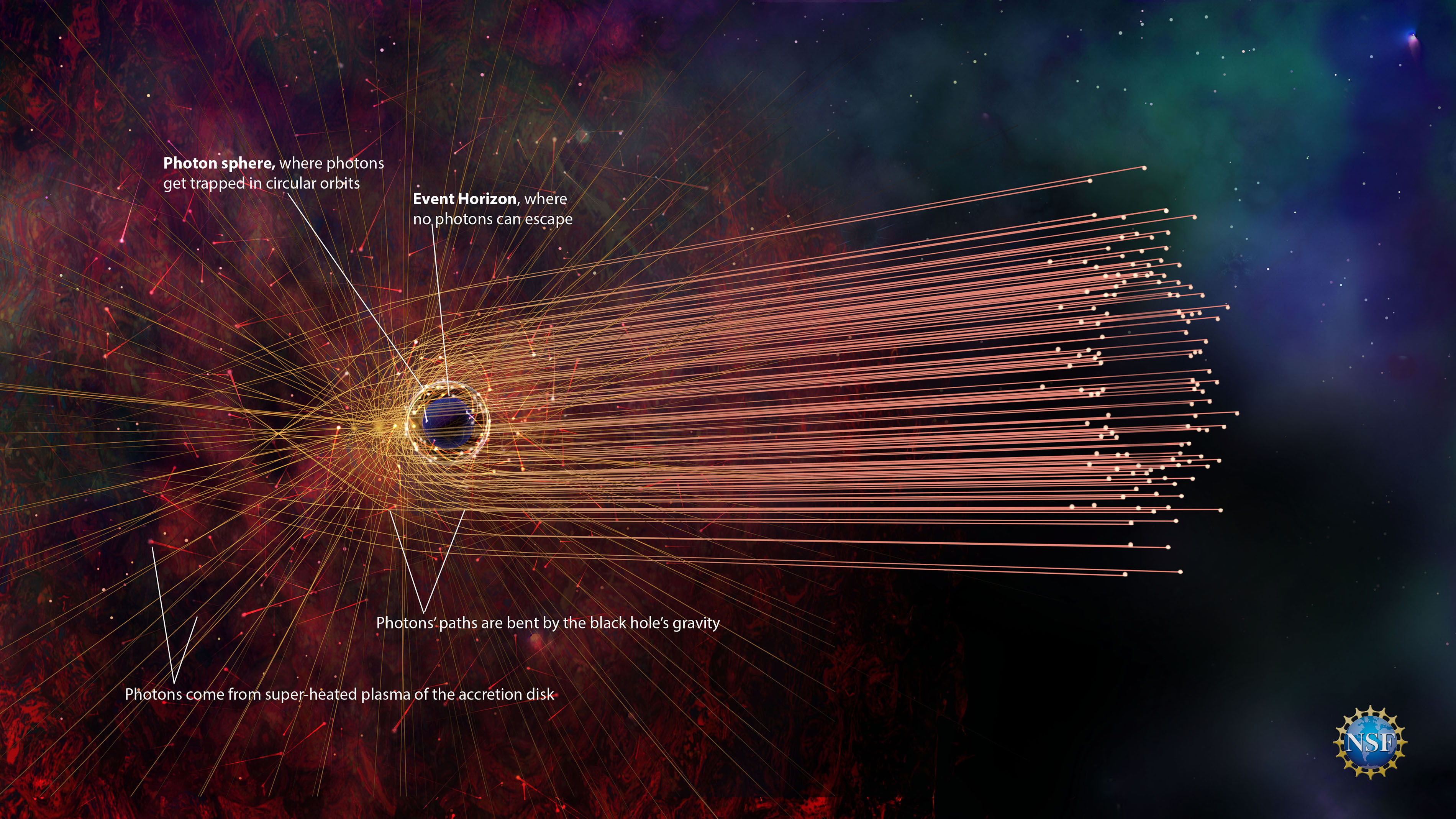
As the matter accelerates, it creates electric and magnetic fields. As the charged particles that make up matter experience those fields, they not only accelerate further, they also emit radiation. And as that radiation experiences the strong gravitational effects of the nearby black hole, it gets bent by the curvature of space. As a result, from our vantage point across the great cosmic distances, we’ll get to observe a ring of radiation around the event horizon of the black hole, and because of the space-bending effects of Einstein’s General Relativity, that ring will have a dark shadow at the center that corresponds to a size larger than the event horizon itself: about 250% the diameter.
That’s the physics that enables an event horizon to be imaged in theory; it’s real, it’s robust, and it’s led to predictions as early as the 1970s that have matched what the Event Horizon Telescope team saw around our first directly imaged black hole: the one at the center of the galaxy Messier 87.
But in practice, pinpointing that radiation is a tremendously difficult task, and it literally took 21st century telescope technology, unprecedented amounts of data that skyrocketed well into the petabytes (where each petabyte is over a million gigabytes), and a global effort to collect and synthesize the data. There’s one technique that made it possible: very long baseline interferometry, or VLBI for short.
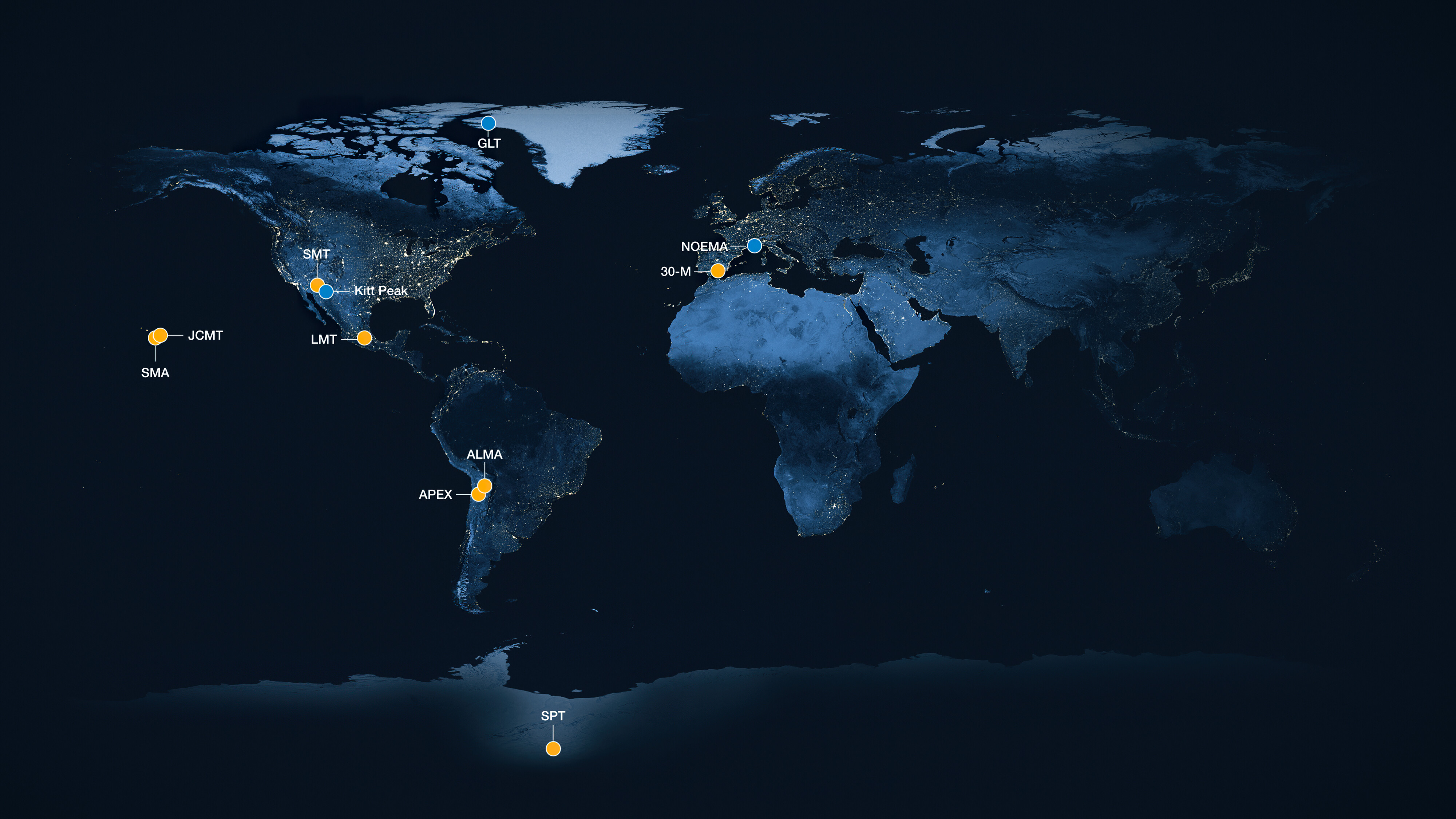
Ideally, we’d be able to construct a telescope that was as large as we dared to dream in order to image whatever object we wanted at the highest resolutions possible. In order to resolve what’s going on with even the most massive black holes closest to our planet — i.e., the ones with the largest event horizons as seen from Earth — it would take a telescope approximately the size of planet Earth itself.
Obviously, we can’t do that; we need the Earth for other things than astronomy, and cannot devote the entire planet to the endeavor of watching the skies.
But we can do something even better: we can set up an array of telescopes all across the Earth’s surface, and we can observe our targets simultaneously from all of those different locations. Even though there’s no such thing as “absolute time,” as time is relative depending on how fast you’re moving and where you’re located, there’s only one reference frame that matters: how long it takes the light to arrive that was emitted all at once from the black hole itself. Even though different points on Earth will have the light arrive at different moments, if we can synchronize those observations together, we can make the Earth behave as one giant telescope.
The Event Horizon Telescope, then, behaves both like a series of individual telescopes and also as one unified observatory. It acts like individual telescopes in the sense of light-gathering power; it can only gather the light that the various radio telescopes can collect individually, added all together. But the technique of VLBI, if the telescopes are properly synchronized together for the object(s) they’re observing at the moment, can enable them to have the resolving power of a single telescope that’s governed by the distance between the telescopes.
At the radio wavelengths that the components of the Event Horizon Telescope’s array are sensitive to, that means it behaves as a single telescope the size of planet Earth, and it can see the brightest objects of all down to resolution of billionths-of-a-degree, or a few tens of micro-arc-seconds. Only the brightest objects are visible, and the brightest objects at those small angular scales will be active black holes that are presently feeding on matter. That’s something we know our galaxy’s central black hole does, as we’ve previously seen emissions that range all across the electromagnetic spectrum from it.

Why is the Milky Way’s central, supermassive black hole so hard to image?
But imaging the event horizon of the black hole at the center of the Milky Way directly would prove to be a novel, herculean task. Even though the black hole we call Sagittarius A* (because it’s located in the constellation of Sagittarius and is a bright, compact radio source) has the largest event horizon of any black hole from our perspective, and the black hole is frequently active in terms of emissions, it’s much more challenging to tease out the shape of the radiation around the black hole than it was for the other large black hole we were able to observe: the one at the center of Messier 87.
In a variety of wavelengths of light, the emissions from Sagittarius A* are easy to see because:
- the black hole is so close to us,
- the black hole is in a dense environment of stars and gas and dust,
- the black hole actively feeds on that matter, which it accelerates,
- and that accelerated matter emits light from high-energy gamma rays and X-rays all the way down to low-energy radio light.
A series of observatories have not only caught those emissions emanating from the galactic center, but have seen flares in those emissions corresponding to various “feeding” events of the black hole as accreting matter flows onto it. But compared to the black hole at the center of Messier 87, getting a single image is far more challenging.
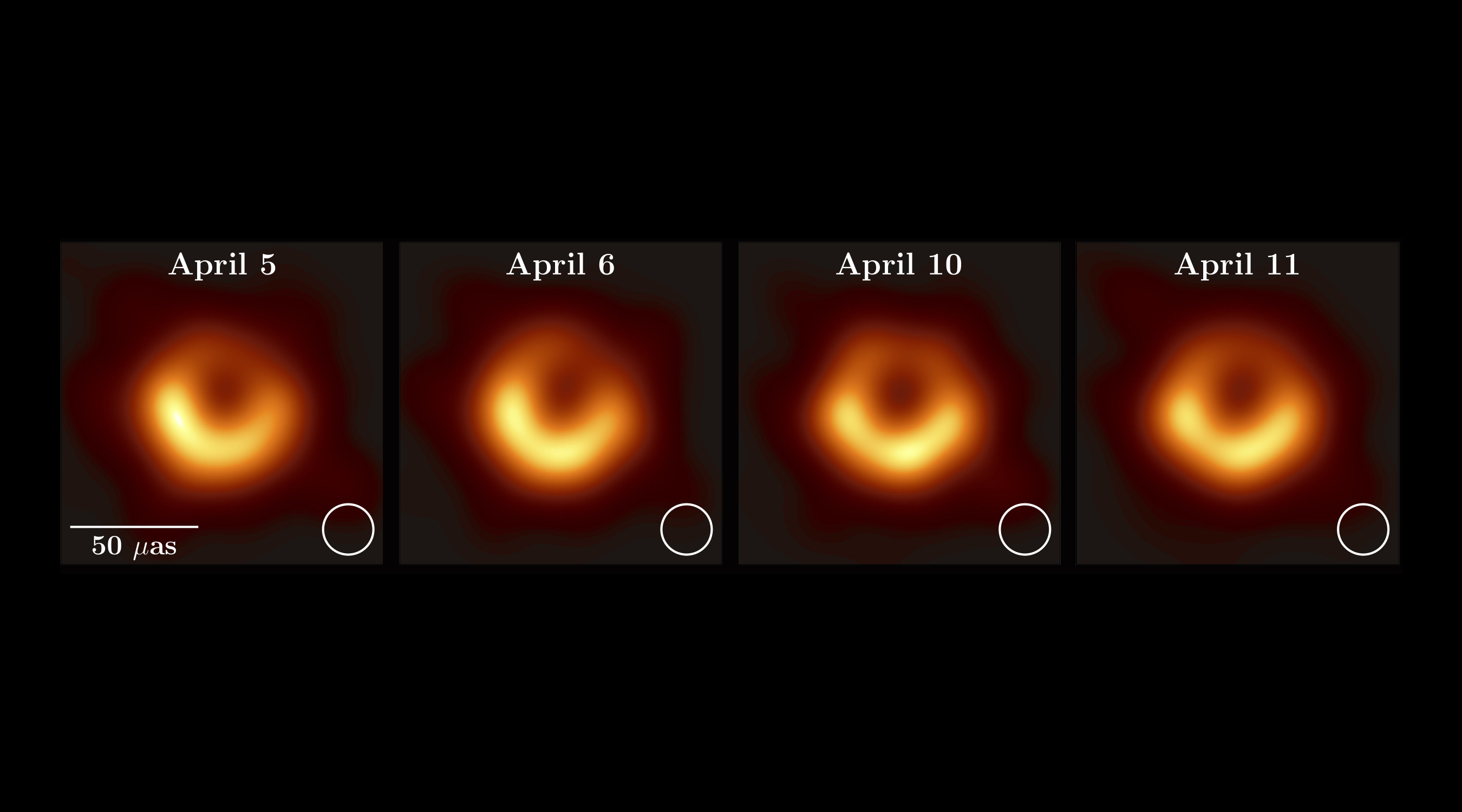
Although most of us remember the release of the Event Horizon Telescope’s first black hole as a single, glorious image, it’s actually better represented as a series of images taken over the timespan of a few days. With a heft of about 6.5 billion solar masses, the amount of time it would take light to traverse the black hole’s event horizon — to go across it from one end to the other — is a little over a full day. The event horizon is huge, and that means that as matter orbits around it, the radiation pattern we see changes over time.
If you make good observations over short periods of time, and “short” must be relative compared to the timescales over which the black hole’s radiation pattern changes, you can produce a single image of the black hole’s event horizon. For Messier 87’s central black hole, you can see that the two images that are a day apart, each, look less different from one another than the images that are separated by nearly a week.
But for the black hole at the center of the Milky Way, it’s a wildly different story. Sagittarius A* might be both close and large, but it’s much less massive than the one at the center of Messier 87. At a distance of just 27,000 light-years, Sagittarius A* would have to be placed more than 2000 times farther away to be at the same distance as Messier 87’s black hole. But Sagittarius A* has a mass of only 4 million solar masses as compared to 6.5 billion solar masses: a difference of around a factor of ~1600. That means the radiation pattern changes not on the timescale of days, but on the timescale of mere minutes.
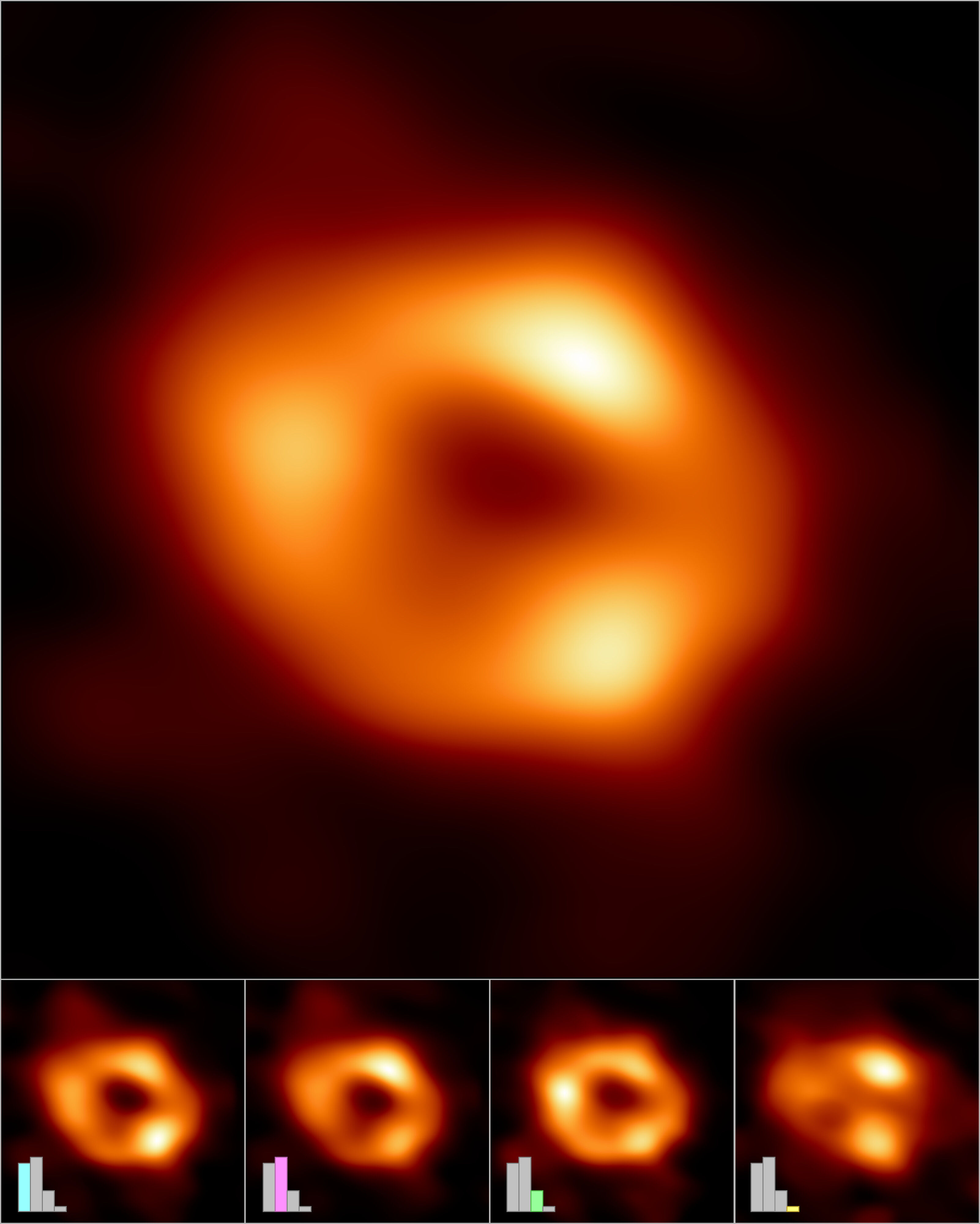
Is that why it’s taken five years to get an image?
Pretty much, yes. Many of us, for the reason that the radiation pattern around the Milky Way’s central black hole must change so quickly, have anticipated that we wouldn’t get just a single image of the radiation around Sagittarius A*, but would instead get a long series of stills and perhaps even a breathtaking movie out of it. Instead, there are thousands upon thousands of images, all averaged together to produce a series of time-averaged views of the central black hole.
The amount of work that goes into getting the synchronization right between the various telescopes at each moment in time — including accounting for the shape of the Earth, the changing atmospheric interference at each individual location, and the fact that it’s rotating relative to the black hole we’re observing — all pose significant challenges.
The overwhelming majority of the efforts of the hundreds of Event Horizon Telescope scientists has gone not into collecting the data or uncovering the theory behind why and how the light bends around the black hole, but rather into analyzing, cleaning up, and making proper use of the enormous amounts of data collected. It’s one of the most ambitious projects ever undertaken in astronomy; it was only made possible by the tremendous technical advances of the ALMA array of telescopes (the Atacama Large Millimetre/sub-millimetre Array); and yet, here we are, in 2022, with our first direct images of the black hole at the center of the Milky Way!
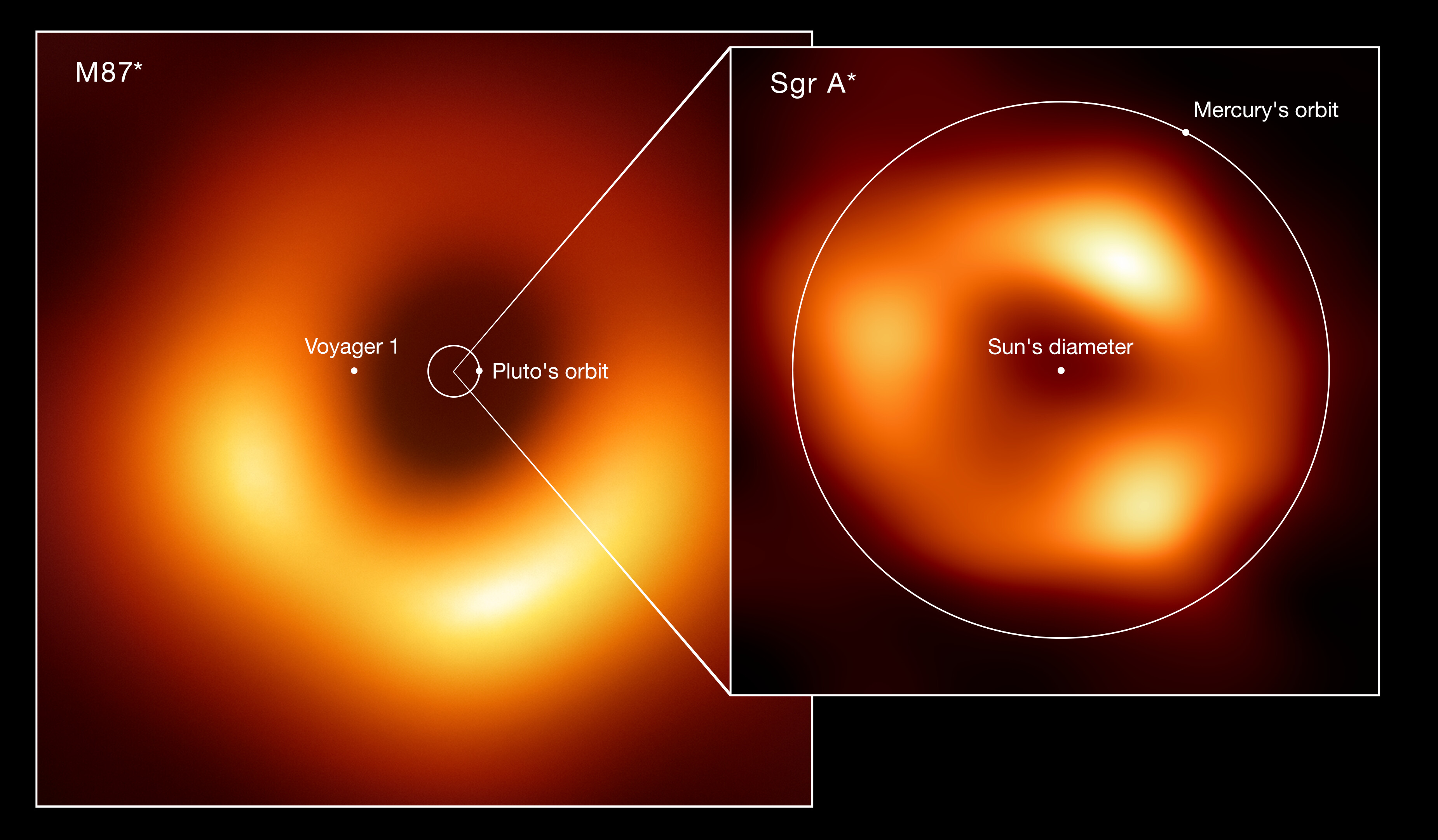
So, out with it; what did we find?
This was announced simultaneously by the European Southern Observatory and the National Science Foundation on May 12, 2022, and wow, it is more spectacular in many ways than almost anyone could have anticipated.
First off, there was a prediction for how large the ring of light ought to be, based on prior measurements of the mass of the black hole Sagittarius A*. When the data came in, it was tested against that prediction and the predictions of General Relativity. Despite the difficulty in constructing this image, the observations agreed with theory to within 5%: a remarkable agreement.
Second off, there were no major flaring events observed during the data run in any of the thousands of images produced, teaching us some remarkable information about how this black hole eats: relatively steadily during times of non-flaring, despite the variability in the motion of the matter around the black hole.
And finally, the construction of the image was only possible through averaging over many component images. When we take the individual snapshots, we can see that there is substantial variability from frame-to-frame, but that many features recur again and again, enabling us to conclude that the structure of the accretion disk and flows evolves relatively consistently in time.
There’s so much to marvel at here. For the first time, we know what the black hole at the center of our galaxy looks like. It does evolve very rapidly in time, and these first results give us hope that with an upgraded Event Horizon Telescope, we might be able to produce a time-evolving movie of what’s going on. There’s consistent “burbling” and “gurgling”, as Caltech scientist Dr. Katie Bouman described, which makes constructing such a movie difficult, but it’s gentler and slower in its evolution than some of us had worried previously.
In the future, we might get polarization data for Sagittarius A*, just like we had previously obtained for Messier 87’s black hole, showing a “cruller-like” structure rather than a donut-like one. There are other targets that the Event Horizon Telescope collaboration observed during its 2017 data-taking run, and perhaps there will be other images of other remarkable objects and features in the Universe forthcoming.
And although the Event Horizon Telescope team did construct a movie of the black hole, they didn’t release it, as the data they had weren’t sufficient to robustly conclude that, in fact, they were observing signal rather than noise.
Regardless, the first image of our galaxy’s central black hole is here, with hot spots and a rapid time-evolution inherent to it. Now that we’ve gotten our first image of the largest black hole as visible from Earth, we can look ahead to an incredibly bright future for this novel, growing field of ultra-high-resolution radio astronomy!