How did Mars get its two “impossible” moons?
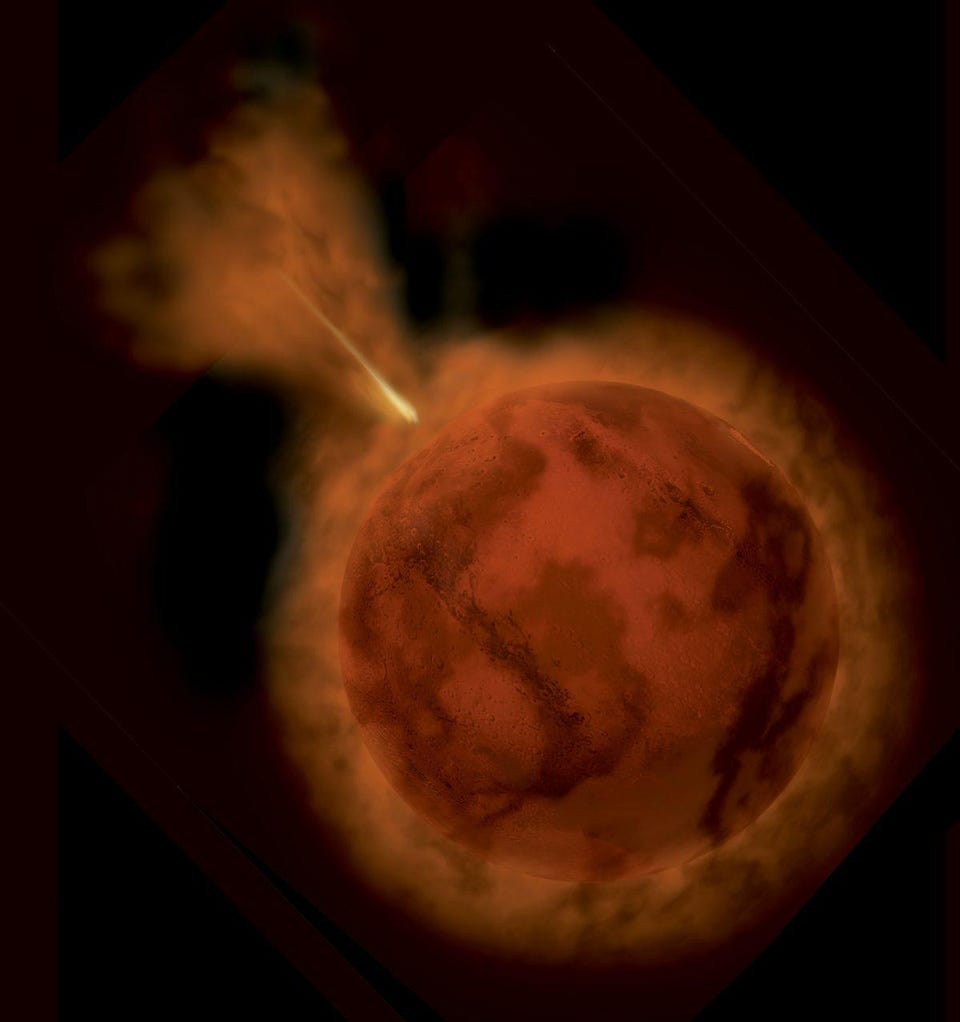
- Phobos and Deimos are the two small moons of Mars, the only rocky planet besides Earth with a moon.
- Captured asteroids would not orbit in the same plane, and impact simulations cannot reproduce Phobos and Deimos.
- But if an impact also created a large, innermost, third moon, maybe Mars can be explained after all.
As far as we know, there are exactly three ways that a planet can wind up possessing one or more moons.
The first way is from a circumplanetary disk, where the material that accrues around a proto-star not only fragments into planetesimals that grow and evolve but the largest protoplanets acquire their own disks of material around them, which leads to moons. This primarily applies to gas giants and is likely responsible for most of the moons in the Jovian, Saturnian, and Uranian systems.
The second way is through gravitational capture, which explains moons with bizarre orbital orientations and densities that do not match up with the parent planet’s material. This applies to moons like Saturn’s Phoebe or Neptune’s Triton, which likely both originated from the Kuiper belt.
And finally, the third way is through a major collision, which kicks up debris that coalesces into one or more natural satellites: This is the likely origin of not only Earth’s moon but all of the moons of Pluto.
And yet, when we look at all three of these methods, not a single one is capable of explaining the Martian system, with its two small, closely orbiting moons, Phobos and Deimos. On the surface, these Martian moons appear to be impossible on their own. Fortunately, when we put the other puzzle pieces together, one scenario stands out above all the rest.
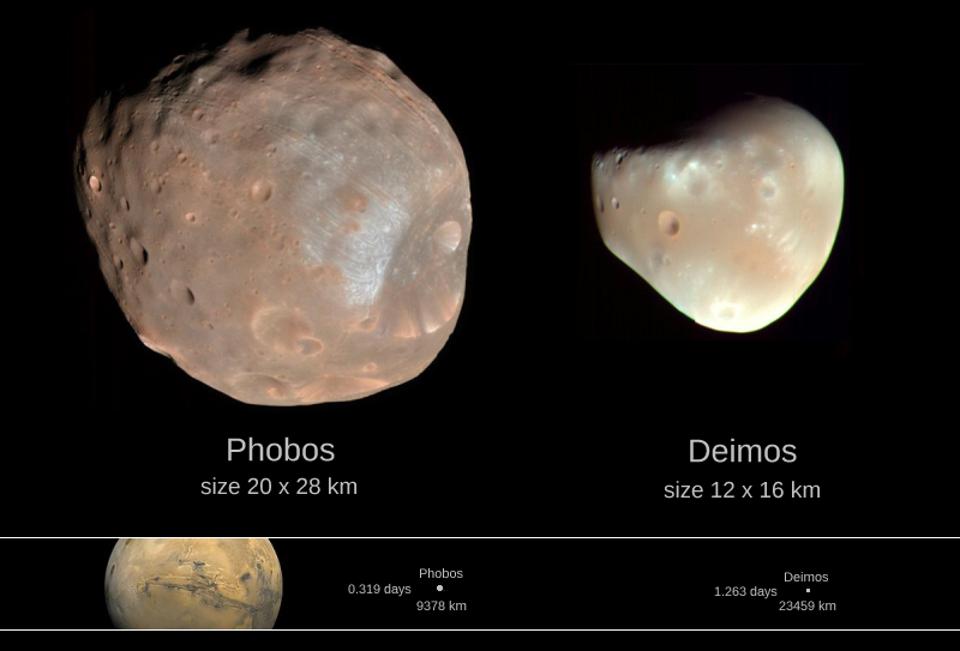
When it comes to planets beyond our own solar system, we have not yet advanced to the point where current technology can unambiguously detect the presence of a moon. Direct imaging has been able to reveal the extended material around a newly forming protoplanet — surefire evidence of a circumplanetary disk that will almost certainly grow into one or more moons — but cannot yet resolve moons around mature exoplanets.
Similarly, the transit method is also limited. Sure, when an exoplanet passes in front of its parent star from our perspective, it blocks a portion of the star’s light, revealing the physical size and orbital period of the planet, once numerous transits build up. If exomoons are present, they can lead to additional flux dips superimposed atop the one caused by the planet and can also lead to transit timing variations as the orbiting moon causes the planet to move forward-and-backward in its orbit by small amounts.
Unfortunately, a non-uniformly reflective planet can exhibit a signal that is observationally indistinguishable from a planet/moon combination with superimposed flux dips. Similarly, the gravitational pull of other masses, like yet-undetected planets, can cause identical transit timing variations as exomoons.
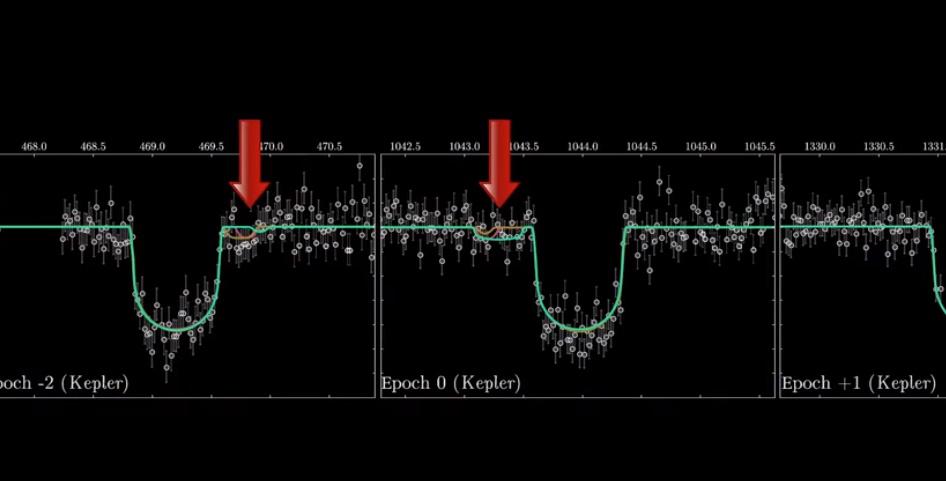
As a result, we can only look to our own solar system for information about where moons come from. When it comes to Mars, though, none of the three methods quite fit the bill.
Why the Mars moons are “impossible”
The circumplanetary disk option only seems to apply to worlds that are massive enough to have come to dominate their orbits very early on in the history of the solar system. Only by gathering large amounts of mass in one place, early on, can a planet draw enough material into its gravitational well to lead to its own lunar system. Put simply, Mars is too low in mass to have formed with moons around it.
Gravitational capture looks tempting, especially given the superficial similarities between Phobos and Deimos and the other asteroids. However, captured bodies always wind up in randomly oriented orbits: frequently inclined and just as likely to be retrograde (opposite to the planet’s rotation) as prograde (in the same direction as its rotation). Yet Phobos and Deimos not only orbit in the same plane as one another, they orbit within ~1° of Mars’ rotational plane. If these are captured asteroids, it was an almost magical occurrence.
And yet, while that leaves the collisional origin as the third and final option, that does not work well, either. No matter what parameters are inputted into simulations — a fast or slow impactor, a massive or low mass one, a shallow or deep impact angle, etc. — there is no combination of parameters that yields two small, low-mass moons like we actually find around Mars.
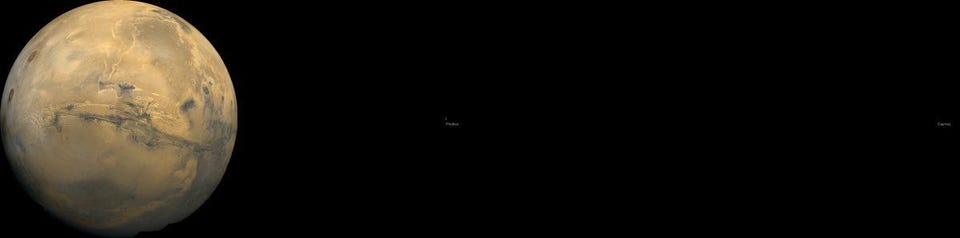
When we take all of this together, it might be tempting to conclude that Mars is a mystery, and the origin of its lunar system remains obscure to us. But the more we have studied the red planet, the more circumstantial evidence we have begun to gather that simply looking at the moons that Mars possesses does not tell us the full story. In fact, the same can be said of the most familiar moon in our solar system: our own.
The origin of Earth’s moon
On Earth, for example, we did not know where the moon came from for an extremely long time. As recently as the 1980s, scientists considered that the moon might be a gravitationally captured object, despite the fact that it orbits prograde, out of the plane of Earth’s orbit around the sun by only 5°, and is tidally locked to the Earth.
However, in order to gravitationally capture a large, massive object, it has to be capable of shedding both momentum and angular momentum, requiring that something else get ejected. Unless Earth had either a rich lunar system of its own at birth — as we assume Neptune once did, leading to the capture of Triton at the expense of all its pre-existing exterior moons — or an enormous atmosphere capable of causing a tremendous amount of aerobraking, the capture scenario would be an impossibility.

Many hypotheses about the origin of Earth’s moon have been floated throughout history, but modern analyses of the material brought back from the moon during the Apollo missions have largely settled the story. The Earth and moon, as determined by analyzing the elements and isotopes that their surface rocks are made from, have identical oxygen isotope ratios, something that is different between our planet and every other planet.
Not only does this point to a common origin for the rocks found here and on the moon, but two other pieces of evidence show that the moon is almost as old as, but not quite as old as, the oldest objects in the solar system. While the most ancient asteroids have been dated to be 4.56 billion years old, we have two independent methods of estimating the age of the moon.
- Lunar samples brought back from the Apollo 14 mission contained zircon fragments, which allow for an incredibly accurate form of radiometric dating to be performed: uranium-lead dating, which yields an age for the moon of 4.51 billion years.
- By combining data about the thermal conductivity of the moon’s crust with the cooling properties of a hypothetical lunar magma ocean and folding in the ages and compositions of moon rocks, an age estimate of 4.43 billion years was obtained.
The Moon formed early on in the history of the solar system, but well after the completion of planetary formation. A major collision is the only explanation that fits on all counts.
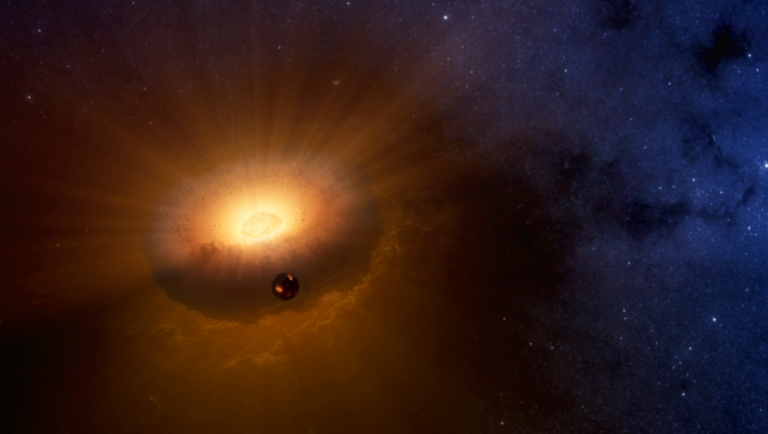
An explanation for Mars’ “impossible” moons
Given that so many pieces of evidence needed to be combined to reveal the origin of our own moon, it makes sense to gather any and all potentially relevant information about Mars and its moons. Sure, our simulations pretty definitively show that no combination of collisional parameters would have produced two small moons around Mars and nothing else, but that does not rule out an impact scenario for the origin of Phobos and Deimos.
Observational sciences, like astronomy, are fundamentally different from laboratory sciences where you can perform and control your experiments however you like. In an observational science, all you get is a snapshot of what the system you are examining is like over the very brief interval you get to observe it.
For our solar system, which has been around for over 4.5 billion years, we have only a few thousand years of documented astronomical history, at most. The moons of Mars were only discovered in the late 19th century, less than 150 years ago. To claim that Mars has two moons is certainly correct, today, but we have to keep the cardinal rule of all observational sciences in mind whenever we draw conclusions. When we look at what we have, today, all we are seeing are the survivors. It is eminently possible that what exists today is just a subset of what once existed long ago.
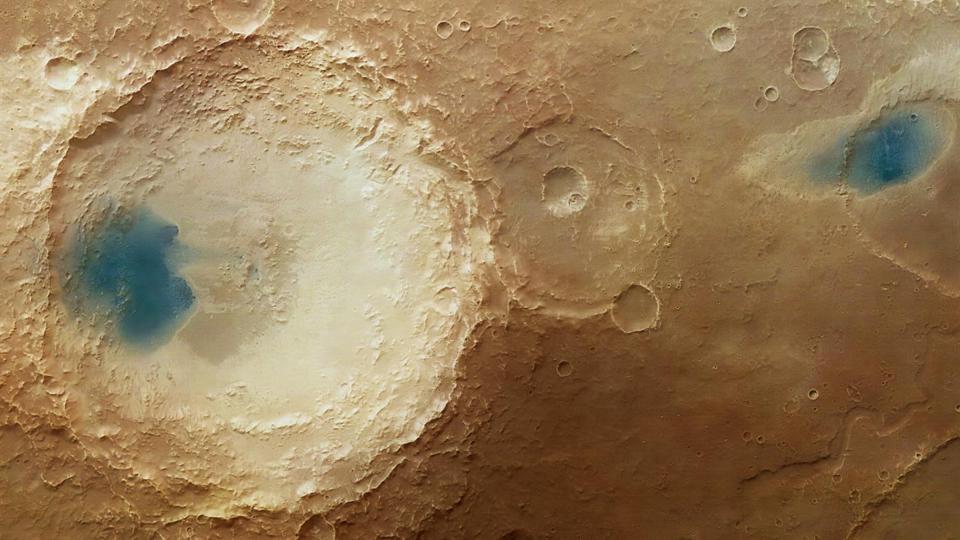
When we look at Mars, it is easy to notice its surface features, which are numerous and varied and tell a dramatic story. Mars has a reddish color to it, evidence of widespread ferric oxide: the result of reactions taking place between iron and oxygen. The atmosphere of Mars is rich in both water vapor and carbon dioxide, both of which can oxidize iron and also combine to point toward a wet, watery history on the red planet. Its presently thin, tenuous atmosphere with what appear to be dried-up riverbeds on its surface and hematite spheres in lowland regions further indicate a watery past, with a much thicker atmosphere that must have persisted for a billion years or more.
But another spectacular feature of Mars is its heavily cratered outer layer, with dramatic highlands and lowlands. Although there are a number of prominent features like mountains, volcanoes, basins, and multiple layers of craters, perhaps the most dramatic difference can be seen between the northern and southern hemispheres of the red planet. While there are topographical variations across both hemispheres, there is an enormous basin covering half of the planet, where for some reason, roughly 50 percent of Mars is around five kilometers (three miles) lower in elevation than the rest of the planet.
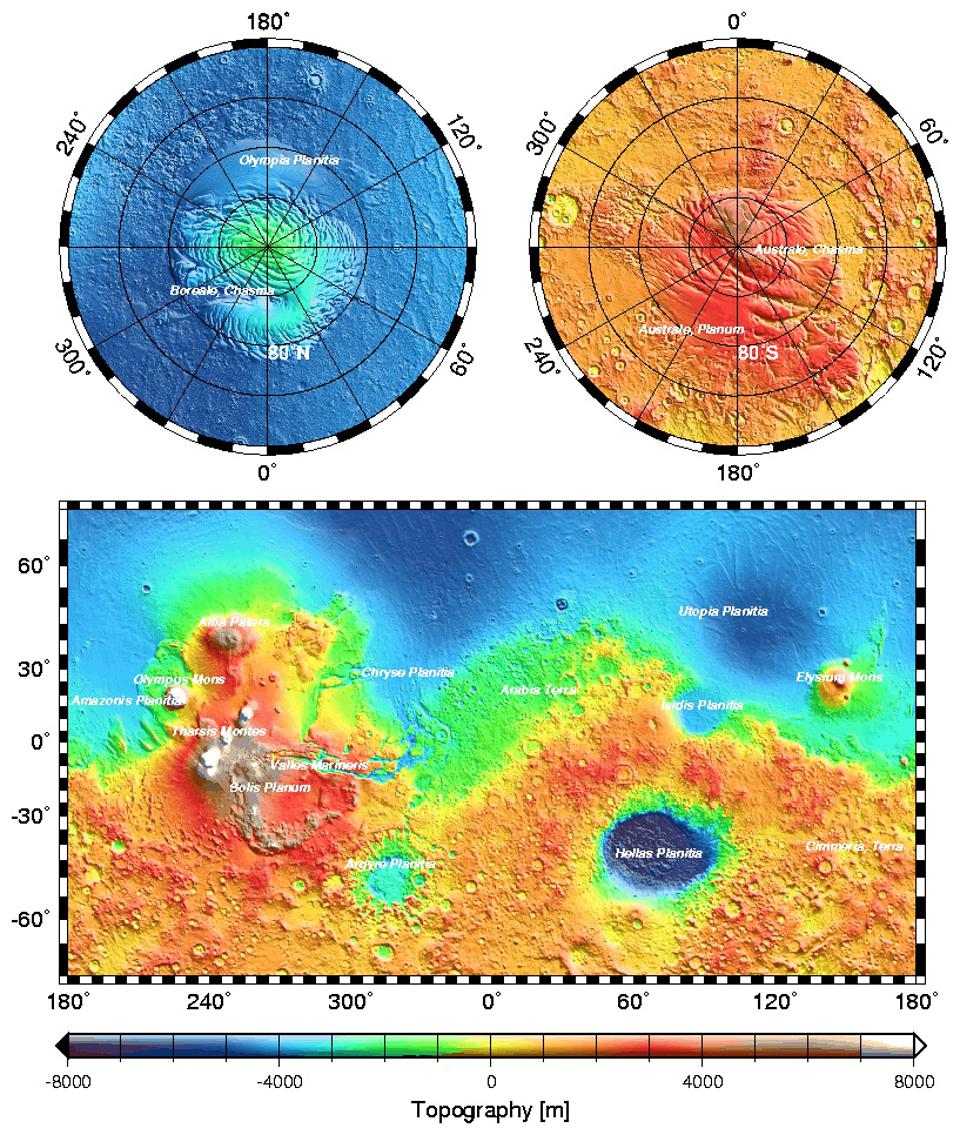
How could this be possible? Even on a geologically active world like Earth, such a configuration probably never existed. Even back when the continents were all interconnected, forming Pangaea, there were likely large ridges, subduction zones, and other tremendous variations in elevation along the ocean floor, preventing the uniform, deep basin that has persisted on Mars for at least the past 3 billion years or so.
It is generally quite difficult to make a planet lopsided like this, particularly if its structure is driven by gradual, internal processes. However, there is an easy way to make a large, deep, sustaining basin: from a large impact. Not, mind you, the type of impact that created our own moon, which required a Mars-sized body striking a world nearly as large as Earth already is today. Instead, a slower collision between perhaps a Pallas-sized body (Pallas being the 3rd largest asteroid in our asteroid belt, well behind Ceres but nearly as massive as Vesta) and early Mars could have left a dramatic scar of precisely this type.
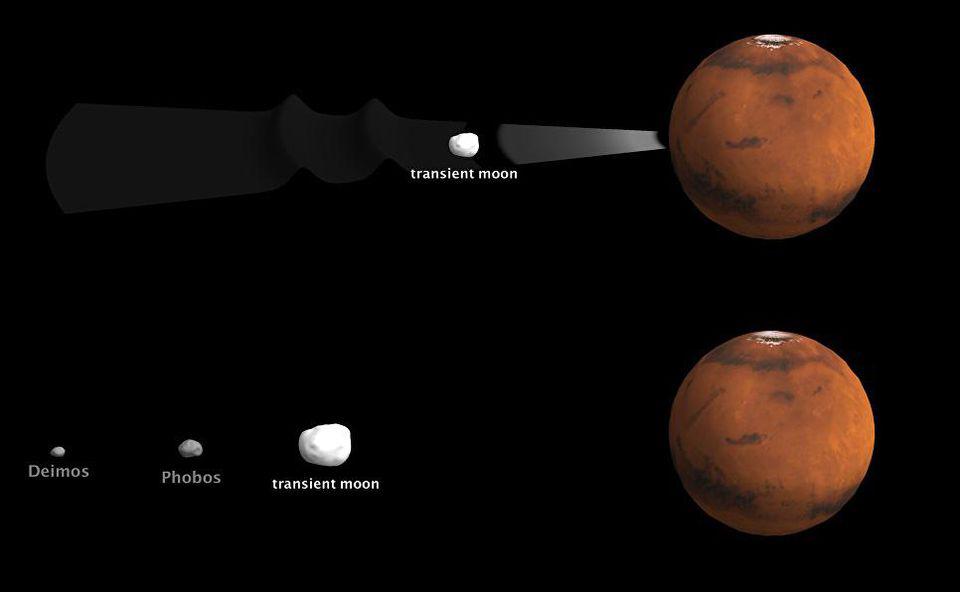
I am not attempting to suggest that a slow, massive collision kicked up debris that then created Phobos and Deimos; that is not consistent with any realistic scenario. Instead, however, it is plausible that:
- an early major collision gave rise to a large debris cloud,
- that cloud coalesced into not two but three moons,
- where the innermost moon was largest, followed by Phobos and then Deimos,
- and then the innermost moon eventually fell back onto Mars, perhaps after being tidally destroyed, creating the depressed basin we see today.
This provides a possible mechanism for explaining what remains around Mars today, while still being consistent with what is obtainable within realistic physical scenarios. When we run our simulations for the types of lunar systems that could have arisen from a giant impact on a Mars-like body back in the early stages of our solar system, a large, few-hundred-kilometer inner moon could have existed alongside Phobos and Deimos, having fallen back to Mars in an era in which only single-celled life existed on Earth. It is the one scenario that has no major conflicts with the full suite of available evidence.
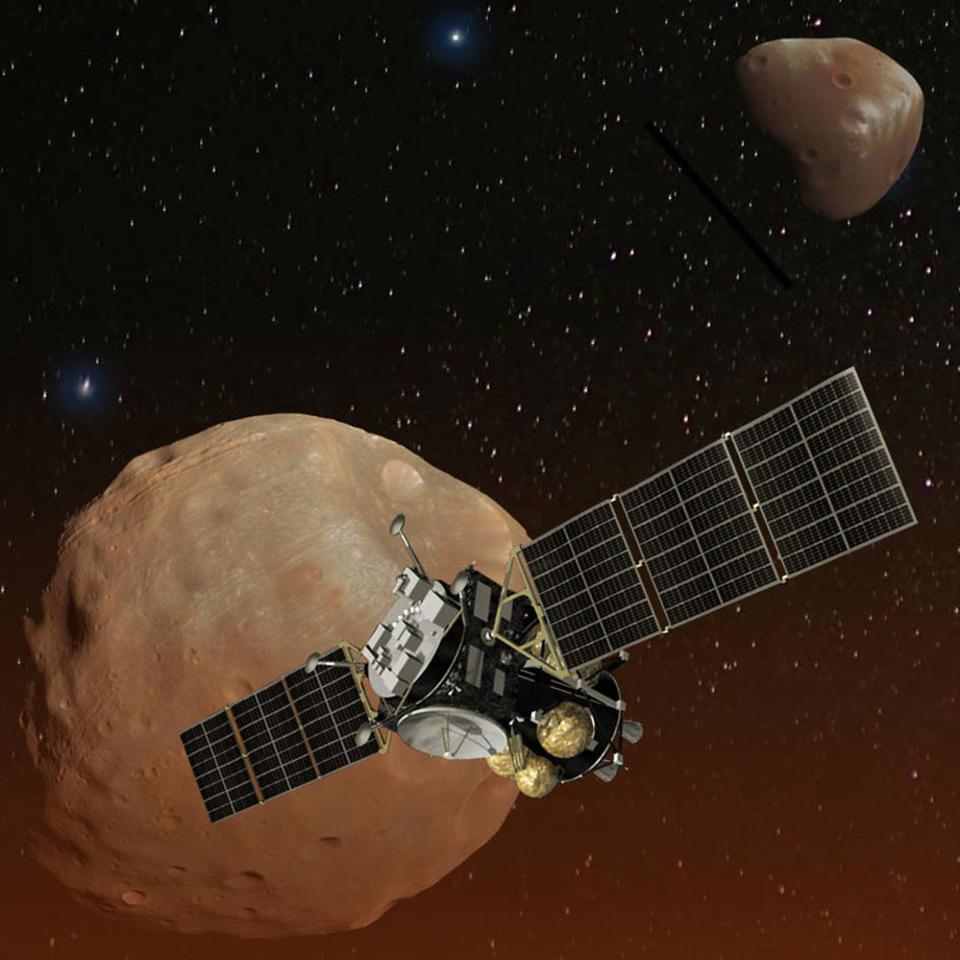
Of course, there is only one way to be certain that Phobos and Deimos are made from the same materials as Mars, rather than being captured asteroids: to land on one of them and return that material to Earth. That is precisely what Japan’s Mars Moons eXploration (MMX) spacecraft is designed to do. The plan is to land on Phobos in 2024, collect material, and return it to Earth before the end of the decade, similar to what previous sample return missions have done on asteroids Itokawa and Ryugu. If Phobos is made of the same material that Mars is composed of, this will be the surefire way to find out.
For more than a century — for practically the entire duration that we have known Mars possesses moons — we have wondered about the origin of these Martian bodies. Yet the fact that we have only been around for an astronomical instant makes reconstructing the history of the solar system that much more difficult. Considering that in another few billion years, Phobos will likely fall back to Mars as well, leaving only Deimos behind, perhaps we should adopt a more positive perspective. After all, at least we have enough remaining clues today to reconstruct the cosmic story that tells us how our solar system grew up. The more time that goes by, the greater the odds that our early history gets overwritten in a way that completely erases the critical evidence we need to know the answer to perhaps the greatest question of all: where did all this come from?