Single JWST image encodes science’s three greatest mysteries

- With a new, incredibly rich mosaic of Pandora’s Cluster (Abell 2744), a cosmic smash-up of at least three separate galaxy clusters, JWST has unveiled a gravitational laboratory.
- The effects of gravitational lensing are extremely strong, revealing the location of mass, the impact of gravity, and a series of ultra-distant, magnified-and-stretched objects.
- From just this cluster alone, we can discover dark matter, dark energy, the matter-antimatter asymmetry, and more. But their physical causes remain mysterious and unknown.
Although we’ve learned so much about the Universe since the dawn of the 20th century, a number of the cosmic mysteries that our investigations have revealed remain unsolved. Arguably, the three greatest ones are:
- dark energy, which drives the expansion of the Universe and dominates our cosmic energy budget, but whose cause is unknown (even despite recent claims to the contrary),
- dark matter, which cannot be made of any of the known particles of the Standard Model and yet which out-masses normal, atom-based matter by a 5:1 ratio,
- and the matter-antimatter asymmetry, which is our observation that there exists 1 proton-or-neutron for every 1.4 billion photons in the Universe, but no corresponding antiprotons-or-antineutrons, despite us not knowing of a single reaction that can create more protons/neutrons than antiprotons/antineutrons.
And yet, we can be confident that dark energy, dark matter, and a cosmic matter-antimatter asymmetry all exist, even if we don’t know how or why they came to be. Remarkably, one single JWST image, assembled as a composite from an imaging campaign known as the UNCOVER survey, allows us to measure and further investigate all of these mysteries, as well as revealing so much more about how our Universe grew up. Here’s what we can learn from this one tiny region of the sky, and the lessons it holds for our entire cosmic history.
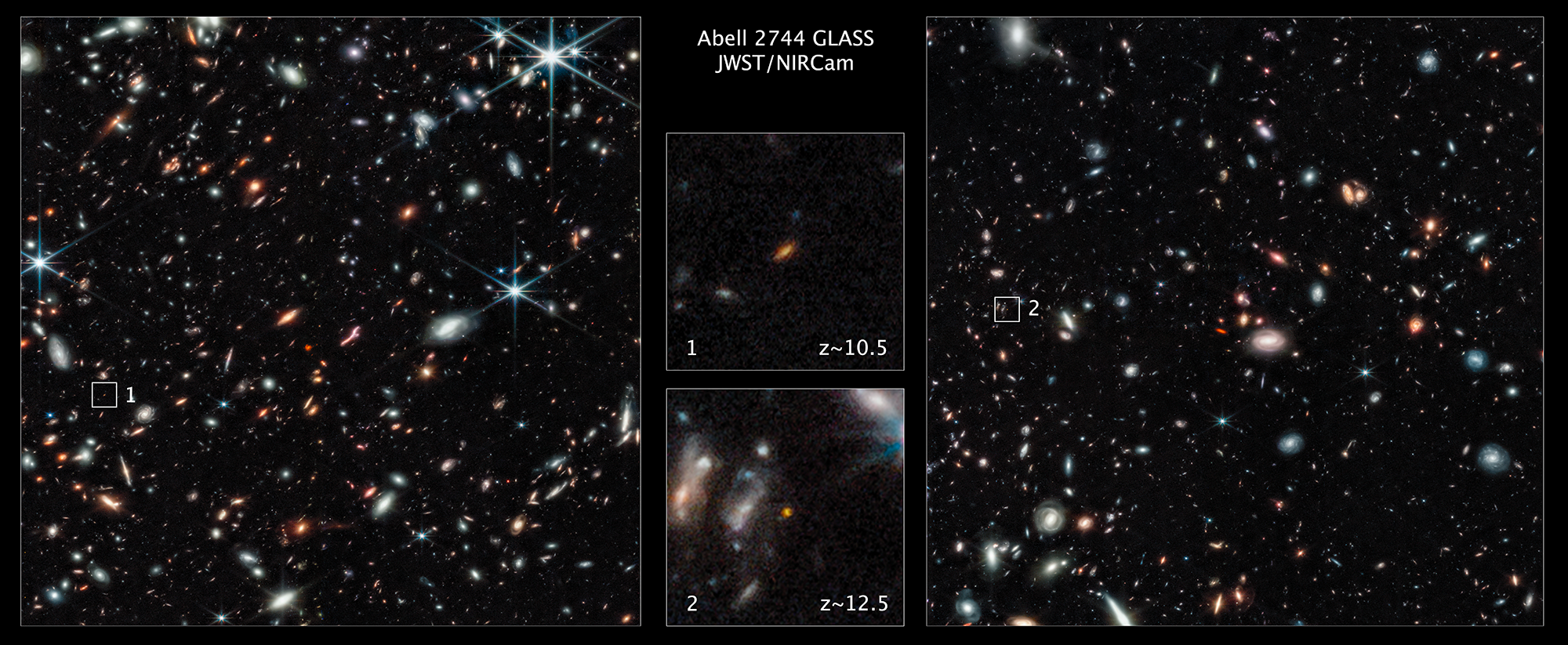
This object itself is a rich cluster of galaxies, and is part of the Abell catalogue that was constructed to map and keep track of rich clusters of galaxies in the distant Universe; this one has the particular number of Abell 2744. It’s been nicknamed “Pandora’s Cluster” after the Greek Mythology story, where Pandora (whose name quite appropriately means “all gifts”) was given a box with “gifts” inside that she was told she must never open. After (of course) opening the box, which set loose all of the ills that would forevermore plague humanity, she rapidly slammed the box shut, sealing inside the last of those gifts: hope.
But Pandora’s Cluster isn’t just a single cluster; it’s a conglomeration of at least three independent galaxy clusters, all in the process of interacting with one another and (eventually) merging together. After being observed by many observatories in the past, including NASA’s Hubble and Chandra observatories, it was most recently the target of the JWST UNCOVER Treasury Survey, with the goal of investigating this object-of-interest even more deeply.
UNCOVER is a (strained) acronym for the Ultradeep NIRSpec and NIRCam ObserVations before the Epoch of Reionization, and its goals were to deeply observe this region of space, uncovering new details into how galaxies grow up and evolve over cosmic time, including very early on.
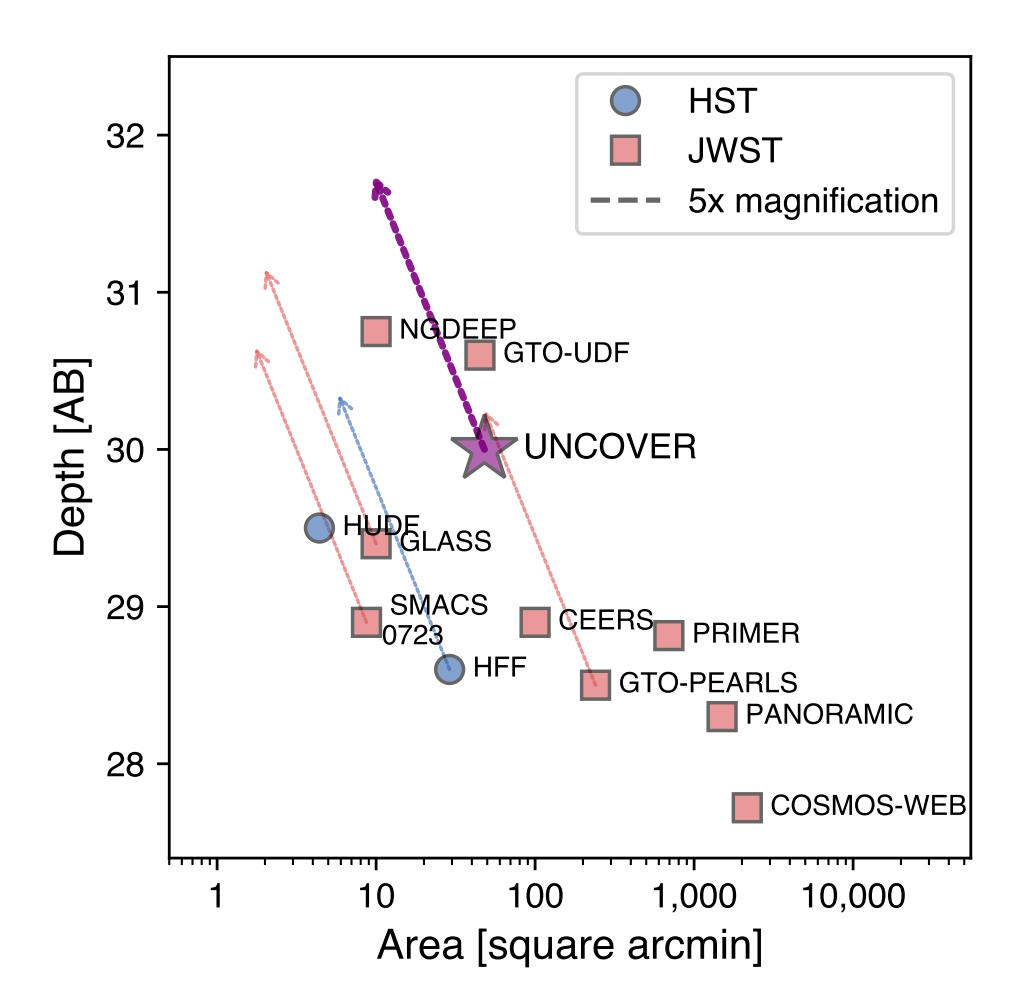
Immediately, the JWST data revealed something remarkable: some 50,000 sources of light across a region of space that covered just 0.007 square degrees of area; a region so small it would take 5,600,000 of them to survey the entire sky.
The brilliant “spikey” feature near the center is the one thing you must ignore: that happens to be a star located within the Milky Way galaxy that’s in the way. Beyond that, there are a few nearby, foreground galaxies in this direction, but most of them are faint and unremarkable. Behind them, there are the main features of Pandora’s cluster: these three galaxy clusters, highlighted in the above image, which contain a myriad of galaxies in various stages of evolution from about 4 billion years ago.
But most of the points of light that exist in this image are neither foreground objects nor are they part of Pandora’s cluster. Instead, they correspond to background galaxies: objects even more distant than the three clusters that compose Pandora’s cluster itself. They extend back into the distant past, when the star-formation rate was much higher (at so-called “cosmic noon,” when star-formation was at its peak), and then even farther: to the earliest stages ever probed.
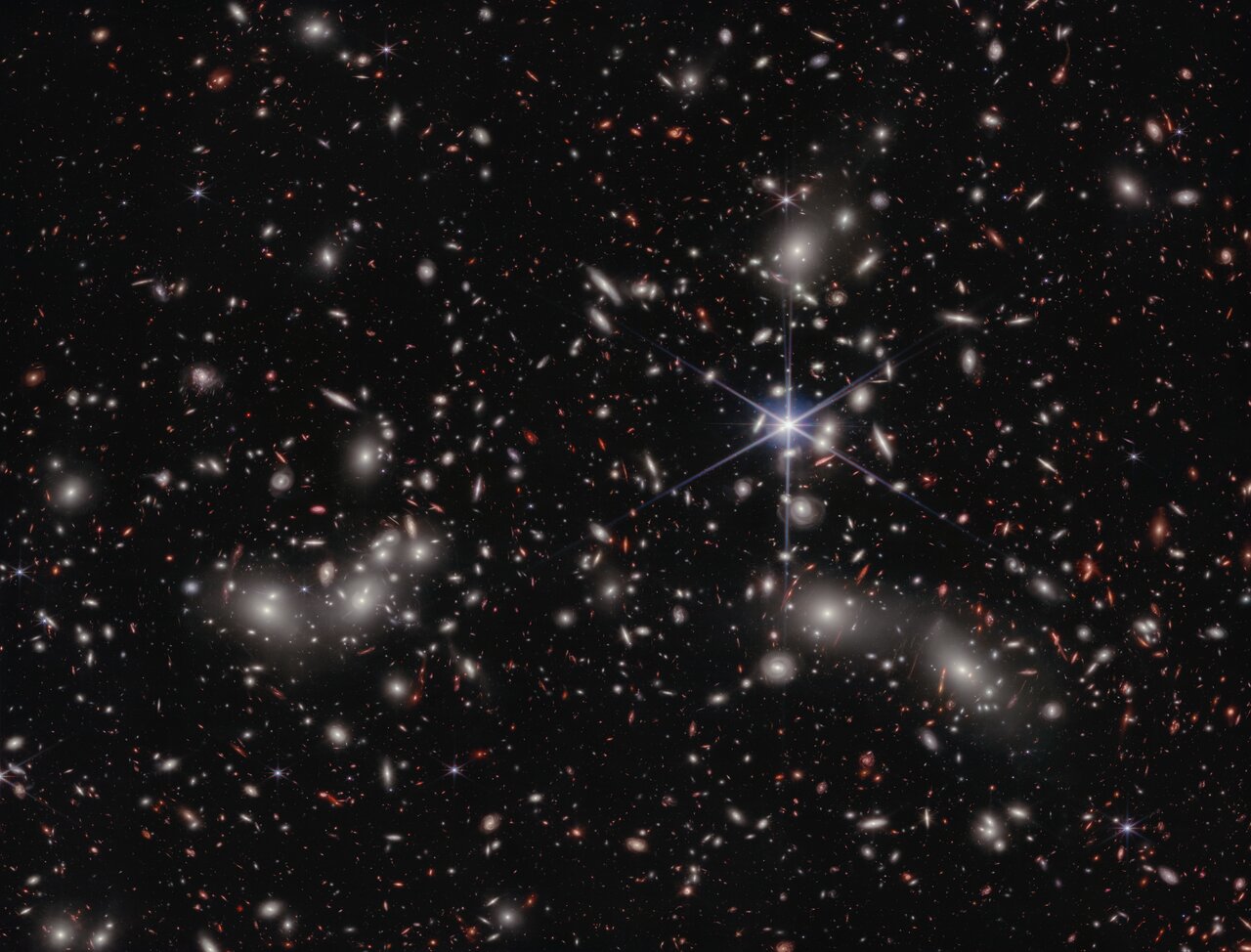
The mass of the three main clusters — combined with around 30 hours of dedicated NIRCam imaging that was performed with JWST — is the reason that the UNCOVER survey can take us farther than any other survey, past or present, that’s ever been performed. Wherever you have a mass in the Universe with other sources of light behind it, that mass behaves as a gravitational lens: distorting the fabric of space and distorting and magnifying the light behind it.
When there’s just the right geometry to that alignment, background objects can be stretched into streaks, arcs, and multiple images, and can be magnified many times over: by factors of 5, 30, or in very rare cases, by factors of hundreds or even thousands. This phenomenon, known as strong gravitational lensing, produces some of the most spectacular lensing features we’ve ever witnessed.
However, irrespective of how good your alignment is, all background objects will experience a more subtle phenomenon: weak gravitational lensing. Unlike strong lensing, which truly enhances what we can observe, weak lensing is primarily a distortive effect, stretching galaxies out along circular/ellipsoidal paths and compressing them in the perpendicular direction. It’s the combination of these two features, of strong and weak gravitational lensing, that most successfully enables us to reconstruct what the masses and mass distributions of foreground clusters are.
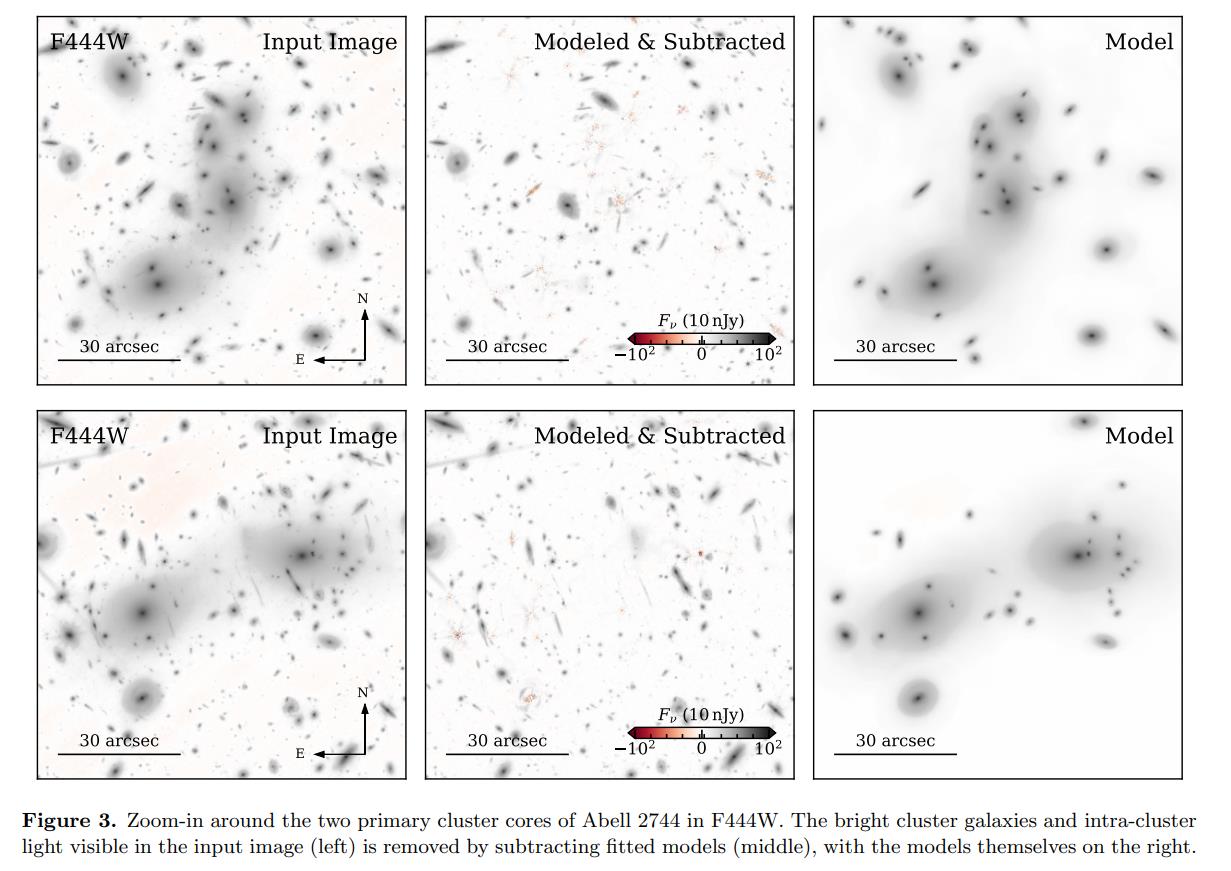
Meanwhile, the galaxies that appear — all 50,000 of them — have been imaged in many different filters, allowing us to obtain redshift estimates for them. Although photometric redshifts aren’t 100% reliable, they’re extremely useful for identifying which galaxies are candidates-of-interest for being ultra-distant objects, and provide us with excellent estimators of how distant and long-ago a galaxy’s light comes to us from.
Because we know which galaxies are part of Pandora’s Cluster and which galaxies aren’t, we can subtract out the cluster galaxies and can additionally “un-lens” them further using what we know about gravitational lensing and the bending of light to determine what their undistorted shapes and unmagnified brightnesses are. We can then use that information, as long as we’ve observed enough galaxies, to determine what the aggregate, average properties of galaxies are and how those properties evolve over cosmic time.
With such good data over a modest-but-substantial portion of the sky, we have almost everything we need, even if we had no other data at all, to determine that the Universe really is:
- full of dark energy,
- rich in dark matter (and that the dark matter is not just “normal matter that’s dark”),
- and made of matter, not antimatter.
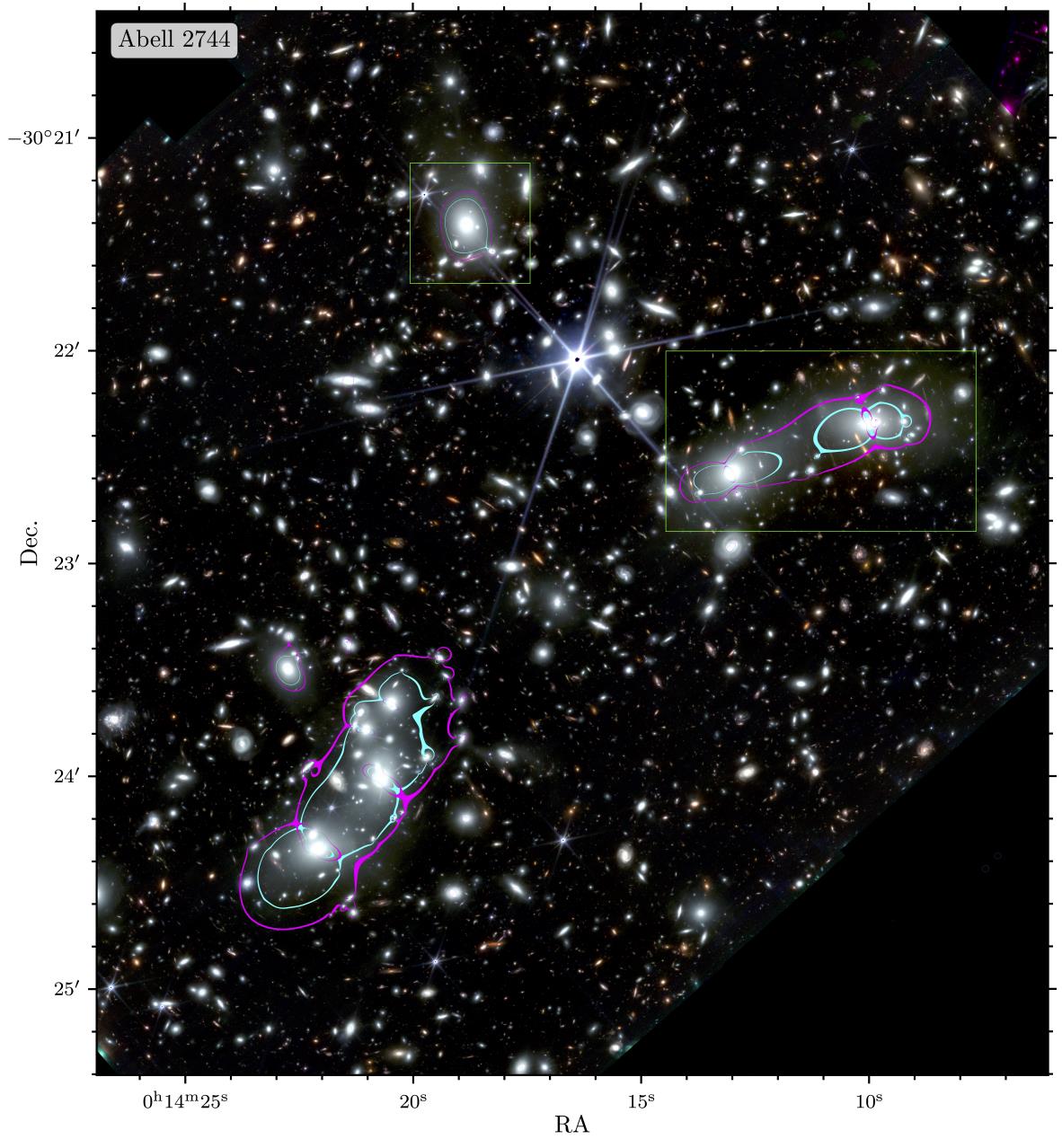
There are many different ways to measure how distant an object is from you. You can know something about how intrinsically bright the object is, and then you can measure its observed brightness, just like if you measured how bright a 100 W incandescent light bulb appeared, you’d be able to determine its distance from you. You can know how something measurable (like the period of a variable star within it) is related to something you want to know (like how intrinsically luminous that star is), and then use what you can measure to infer distances. Or you can leverage one of any number of empirical correlations — the Tully-Fisher relation, the Faber-Jackson relation, Surface Brightness Fluctuations, etc. — to infer the distance of your object based on measurable properties.
Because we also can measure (or at least estimate, from photometry) a distant object’s redshift, we can come up with simultaneous pieces of information for:
- how distant an object is from us, right now, in light-years (or whatever unit of distance you like),
- and how fast it appears to be receding from us, based on our models of the expanding Universe,
and that reveals to us what our Universe is made of. This simple idea, of measuring objects at a variety of distances from us throughout the Universe’s history, is the “distance ladder” method of measuring the expanding Universe, and was the method that first revealed dark energy’s presence to us.
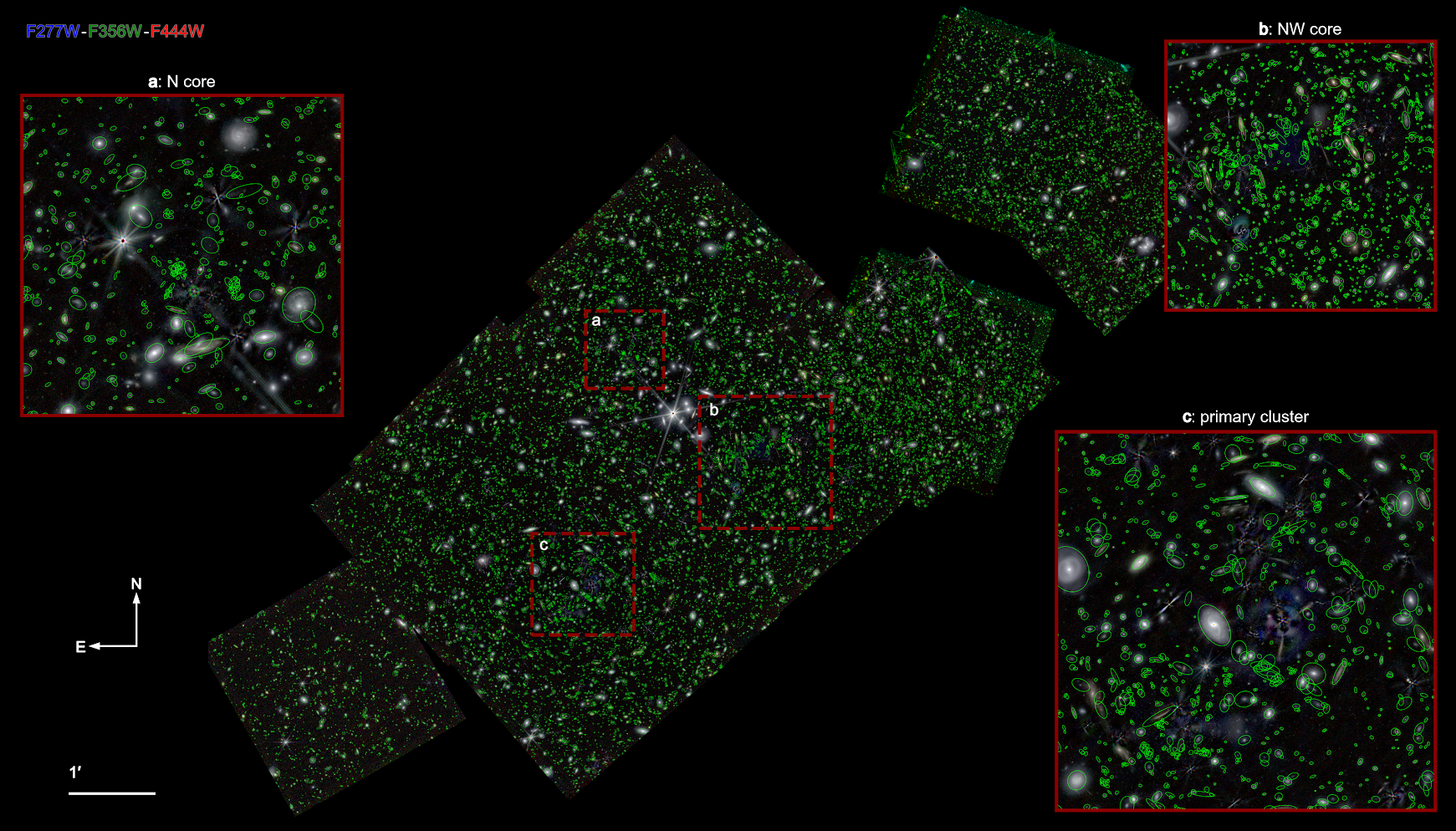
Another measurement we can make has absolutely nothing to do with what JWST is capable of, but rather highlights how important multi-wavelength views of the Universe are to the scientists that study it. Galaxies and galaxy clusters aren’t only made up of stars, but also of material between the stars: gas, dust, and plasma as far as normal matter is concerned, and if it exists, dark matter as well. When galaxies and galaxy clusters collide, the material that’s composed of normal matter interacts: forming stars, heating up, ionizing, and slowing down. The individual stars and the dark matter (if present), however, don’t interact in these ways, and instead they simply “coast” freely, unslowed by these interactions.
By looking in X-ray light — the specialty of NASA’s Chandra observatory and the great hope of the scientists who want to build the next-generation Lynx observatory — we can construct a map of where the normal matter that isn’t in stars is located. And by comparing that map with the gravitational lensing map, which maps out the total amount of mass present within the foreground object, we can determine whether there’s dark matter or not, how much of it there is if it’s present, and how its distribution does or does not align with the distribution of normal matter.
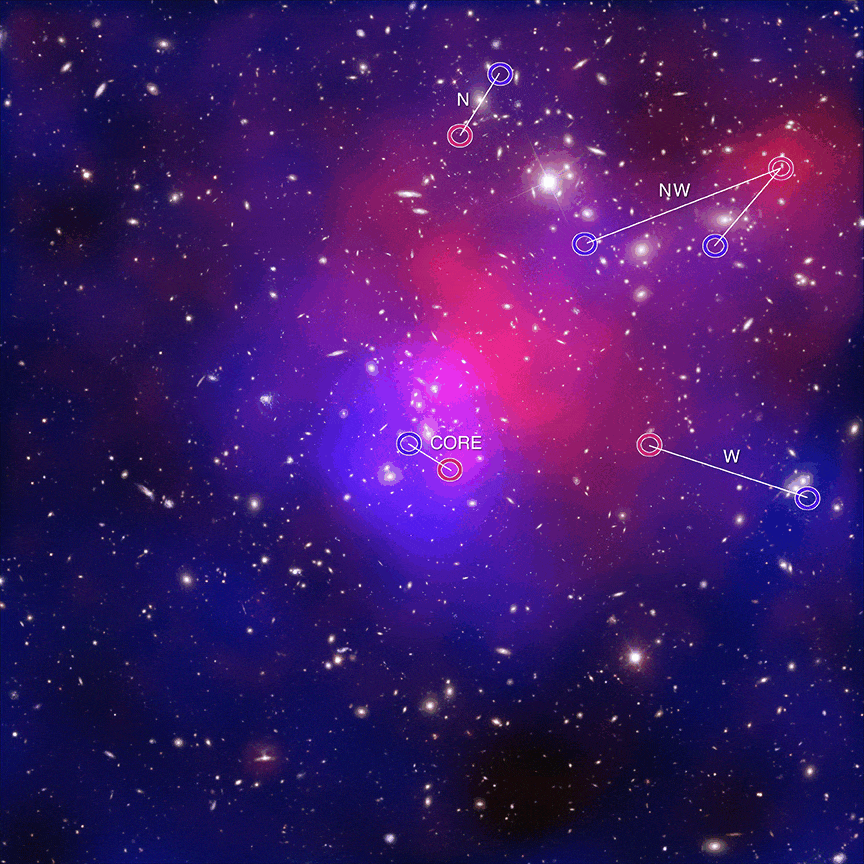
Only around 20% of the Pandora Cluster’s mass can be accounted for by normal matter, above, shown in pink, while at least 75% (and possibly more) of the total mass that causes the gravitational lensing effects must be in the form of dark matter, consistent with what we’ve found from similar cosmic smash-ups found elsewhere.
And finally, the alternative to having a matter-antimatter symmetry that’s the same all throughout the Universe would be to have stars, galaxies, or galaxy clusters found in different regions of space being made equally of matter and antimatter, only separated by substantial distances. There are many mechanisms one could imagine that would create some parts of the Universe that contain matter while others contain antimatter, and just as matter forms atoms, molecules, gas clouds, stars, galaxies, and clusters, there could be regions with anti-atoms, anti-molecules, and all the way up to cosmic structures made of antiparticles as well.
Here’s the thing, though: when different cosmic objects collide or otherwise come into contact with one another, they’ll interact according to conventional rules if they’re both made of matter (or antimatter), but if matter-antimatter regions contact one another, they’ll annihilate, creating an unmistakable signature of ultra-high-energy gamma rays.
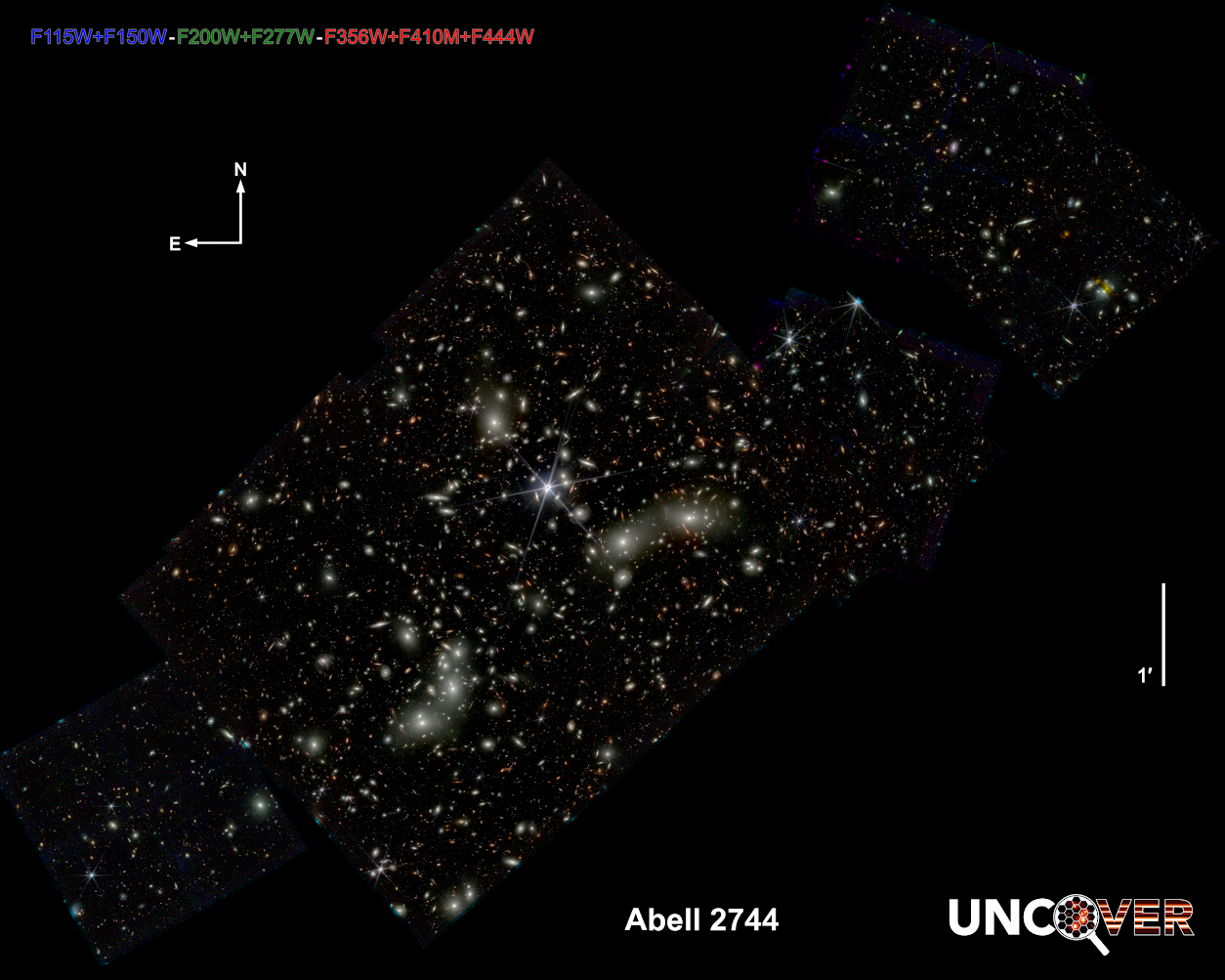
The lack of that signature:
- between clouds of gas in the intergalactic medium,
- between stellar systems within a galaxy,
- between galaxies within a cluster,
- and between clusters that collide and interact,
places incredible constraints on “How much of the Universe could be made of antimatter?” Even from objects like Pandora’s Cluster on its own, we can learn that no more than about 0.001% of the Universe is allowed to be made of antimatter, confirming for us that yes, there really is a matter-antimatter asymmetry within our observable Universe.
Additionally, we can learn all sorts of other information, like how galaxies grow and evolve over cosmic time. We can measure the star-formation rate of the objects within our cosmos and see how they evolve over cosmic time. We can examine galaxies and stars that formed within the first few hundred million years of the Universe to learn when and how the neutral atoms in the intergalactic medium became reionized. And we can look at the earliest galaxies of all to learn about star-formation and galaxy-formation and even how supermassive black holes first formed and began to grow up within the Universe.
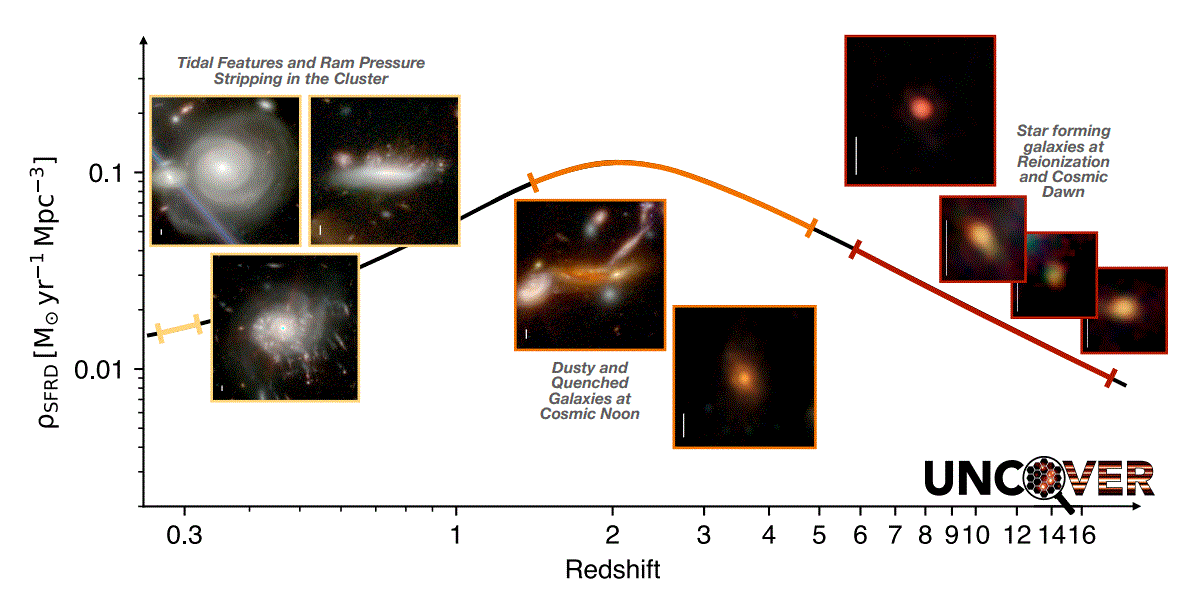
It’s hard to fathom, but if the modern Milky Way is akin to a 100-year-old human being, then the galaxies we find in Pandora’s Cluster are much more spry: like a 70-year-old collection of people, while the galaxies at “cosmic noon,” when star-formation peaked, are more like 20-year-olds in the prime of their youth and athleticism. JWST, with the UNCOVER survey and similarly deep studies, can take us all the way back to teenagers, children, and even toddlers, with the current record-holder appearing as the analogue of a 2-year-old. At its absolute limits, JWST could even take us back to galaxies in their true infancy: in the range of 8-to-18 month-old humans.
It can’t quite see all the way back to newborn galaxies, but the UNCOVER survey could yet be hiding a yet-to-be-identified cosmic record-breaker, just waiting to be spectroscopically observed and confirmed. Even though this survey is large enough to deliver some ~50,000 unique objects, it’s only as big on the sky as a dot of nail polish on one of your fingernails, held at arm’s length: 1/5600000th of the full sky. The full resolution NIRCam mosaic delivers a whopping 200 megapixels and will help us understand how galaxies were born and grew up all throughout our cosmic history. Although more data always enables superior conclusions, this one image is completely sufficient to reconstruct almost all of our cosmic history, and even just a tiny snippet of it can leave us agog with a sense of wonder at how marvelous it all truly is.
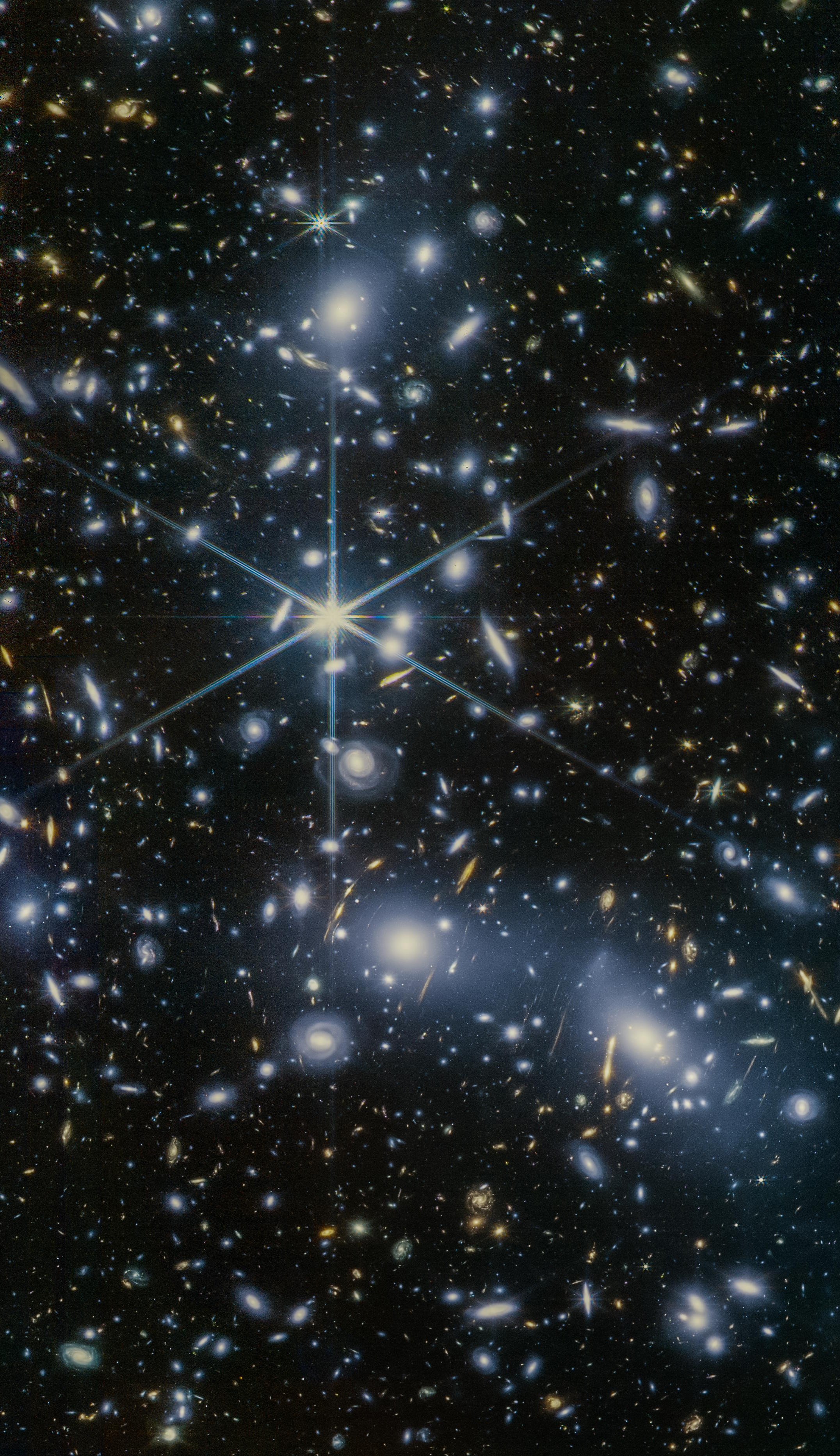