How are neutron stars magnetic?
If spinning-and-moving charges make magnetic fields, why does a giant neutral thing have one?
Image credit: NASA, Chandra X-ray Observatory, SAO, DSS, via http://apod.nasa.gov/apod/ap140725.html.
“By allowing the positive ions to pass through an electric field and thus giving them a certain velocity, it is possible to distinguish them from the neutral, stationary atoms.” –Johannes Stark
A little bit of physics goes a long way, and that’s especially true in astrophysics, where the tiniest of forces and the smallest of effects become the only things that matter. It is, of course, due to the extreme concentrations and amounts of material that we’re dealing with! Take something as innocuous as our little, insignificant planet.

The fact that we have a molten, rotating and changing core with an active magnetic dynamo inside of it does much more than make compass needles point towards the pole. The magnetic field generated at the Earth’s core extends well out into space, protecting us from cosmic dangers and diverting fast-moving charged particles away from us.
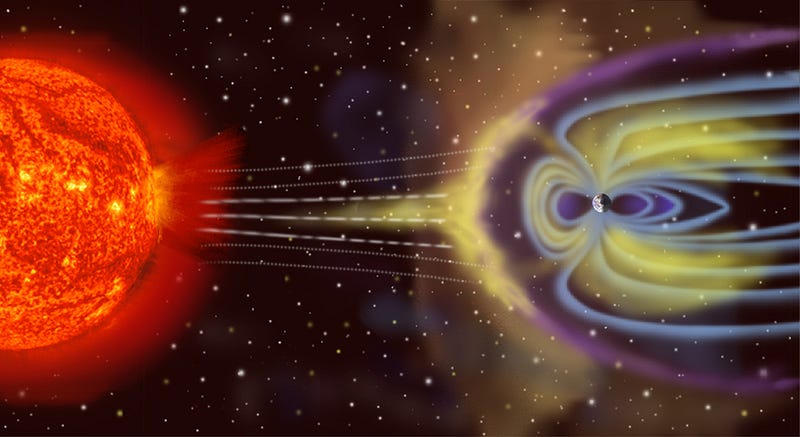
The Sun gets in on the action to an even greater extent; its magnetic field is huge, and the plasma often traces out the path of those field lines. We can often see the hot, ionized plasma of the Sun extending upwards and outwards many times the diameter of the Earth, even (on occasion) forming a complete loop and “raining down” like a fiery waterfall.
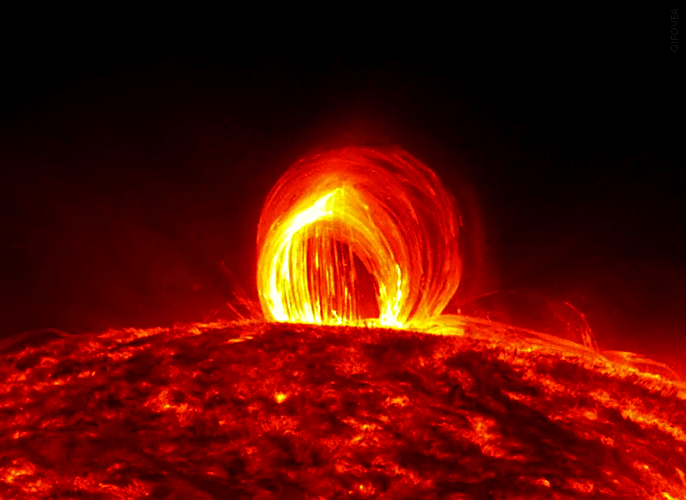
It’s not so hard to imagine why the Sun or the Earth does this. Think about the following facts:
- These objects are made up of atoms, which in turn are made up of positively charged atomic nuclei and negatively charged electrons.
- There’s a gravitational gradient and a temperature gradient, meaning that objects of different sizes, masses and cross-sections will be affected differently.
- If these phenomena can produce even a small separation of charge, since the Sun and Earth are spinning, these charges that move differently will generate magnetic fields.
And then we’re done!
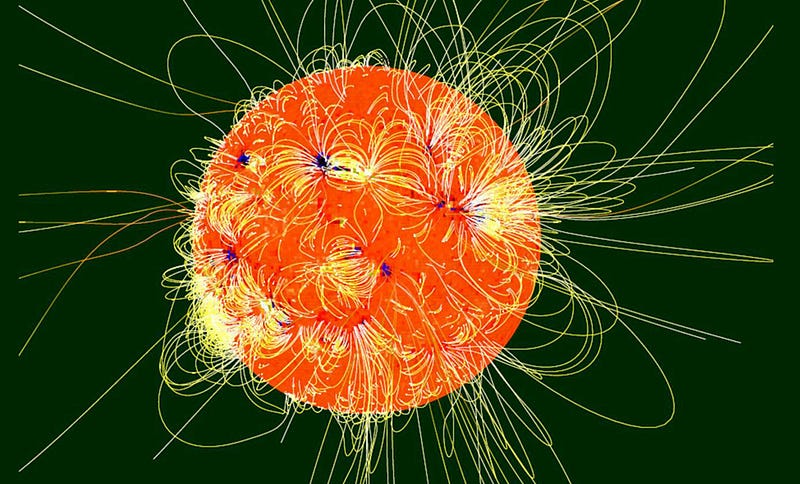
But what about neutron stars? Instead of being made out of atomic nuclei and electrons, aren’t they made out of… well, neutrons?
You know, those neutral things — found in atomic nuclei — that aren’t charged?

So how, then, would they make a magnetic field, which themselves are generated by moving electric charges?
This wouldn’t be such an interesting question if we hadn’t made observations like this one.
These are X-rays emitted from the Crab Nebula, as observed with NASA’s Chandra X-ray telescope. We know there’s a pulsing neutron star at its core, and that these X-rays are emitted as a result of a centrally located intense magnetic source affecting the ionized plasma around it.
It’s more than just in the X-ray, mind you; Hubble sees these effects in visible light, too!
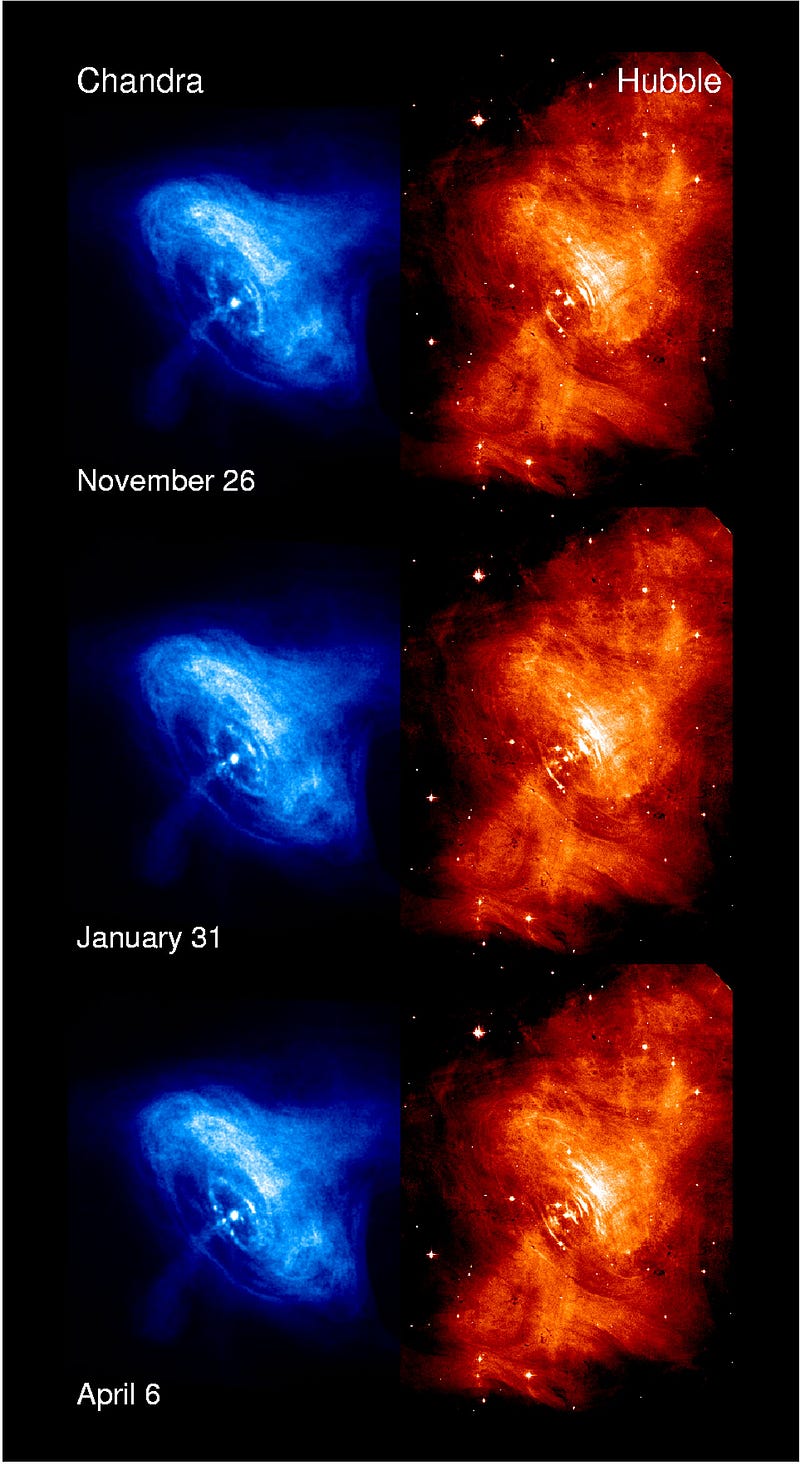
And as far as scale goes, the Crab Nebula — created in a 1054 supernova explosion — is about 3 light-years in diameter by this point, nearly a millennium after its birth. But what might surprise you is the tremendous size of this magnetic feature; it’s more than a light-year in size on its own!
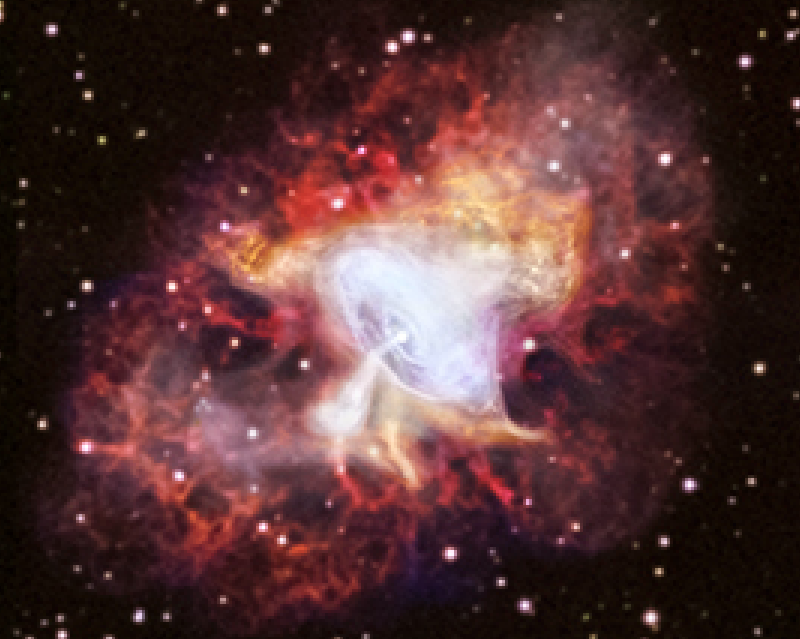
The key is that a neutron star isn’t just a simple ball of neutrons; it’s actually layered. As we progress from the outside-in, we find layers of:
- electrons, followed by
- the nuclei of atoms (like iron), followed by
- a layer where nuclei are layered (like impurities) inside an ocean of neutrons, followed by
- a transition zone to the core,
- where the core is a neutron superfluid (a liquid-like phase with absolutely zero friction) along with charged-particle impurities of various masses inside of it.
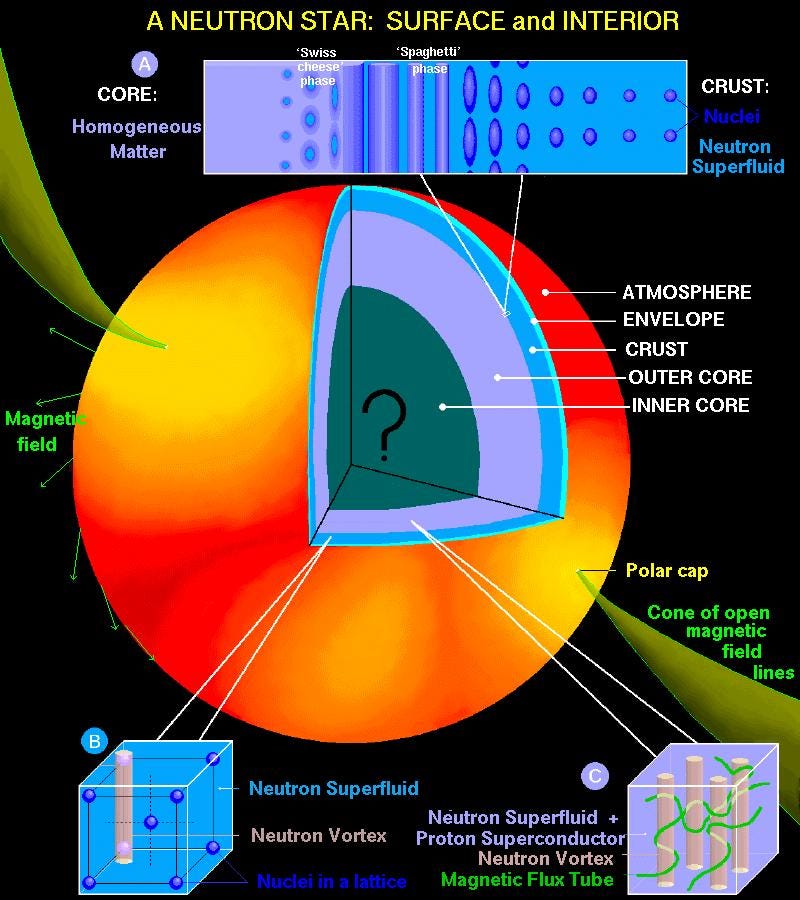
It’s not like having one single, neutral entity at all! And don’t forget that neutrons themselves are not fundamental, neutral particles, they themselves are made up of charged particles that have different charges and masses from one another!

The neutrons themselves have intrinsic magnetic moments (since they’re made up of these charged quarks), and the incredibly high energies inside the neutron star can not only create particle/antiparticle pairs, but can create exotic particles as well. The charged particles that exist inside the neutron star are highly conductive, plus there are still gravitational, density, temperature and conductivity gradients inside of the neutron star.
And at approximately 10 km in radius — with all the angular momentum of a typical Sun-like star — these things rotate at speeds of between 10-and-70% the speed of light!
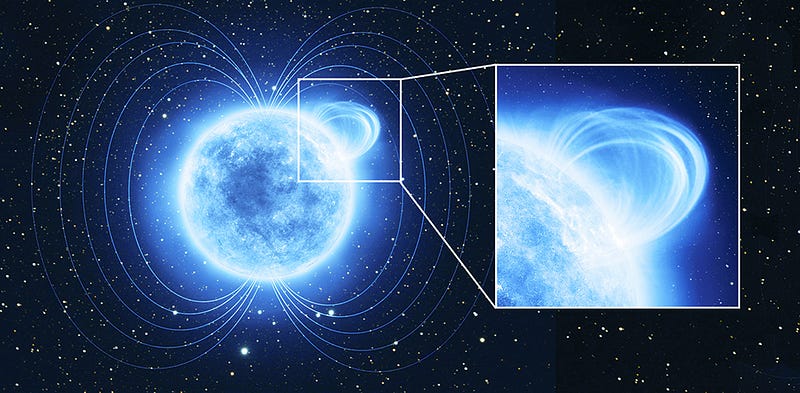
In short, that’s a recipe for a magnetic field on the order of 100 million Tesla, or about a trillion times what we find at the Earth’s surface.
No wonder that’s exactly what we see! Even without being absolutely certain as to what’s happening in the innermost core of a neutron star — whether we have high-energy quarks, muons and taus, or any other types of particles rarely found in nature — conservative, conventional physics in these extreme environments makes an ultra-strong magnetic field all but inevitable.
And that’s how a neutron star generates a super-strong magnetic field!
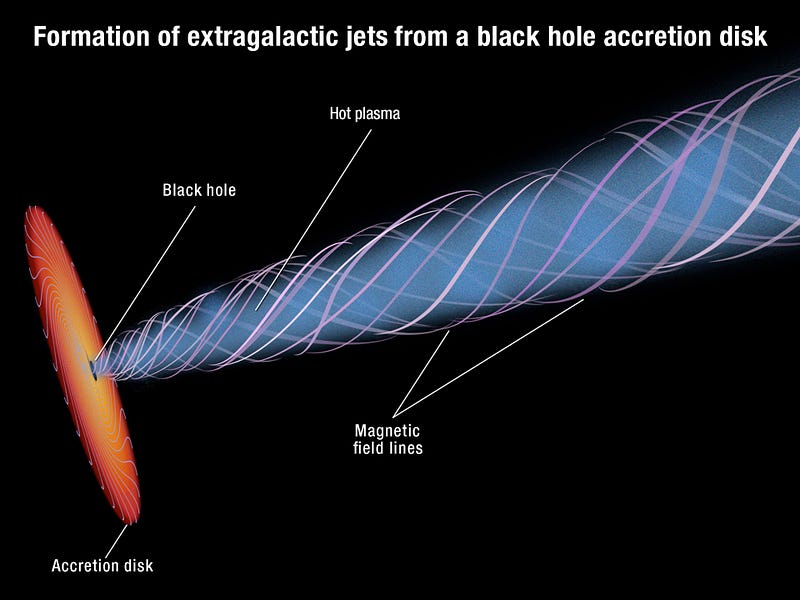
Now the big next question is: can we have a super-strong magnetic field coming from the inside of a black hole? (We see black hole magnetic fields, but are they generated inside the event horizon or outside, such as in the accretion disk?) And if they do come from the inside, what’s the physics behind that? Until we know the answer, the question provides us with more than enough food-for-thought to sate even the hungriest appetite!
Enjoyed this? Leave your comments at the Starts With A Bang forum here!