Heisenberg’s astrophysics prediction finally confirmed after 80 years
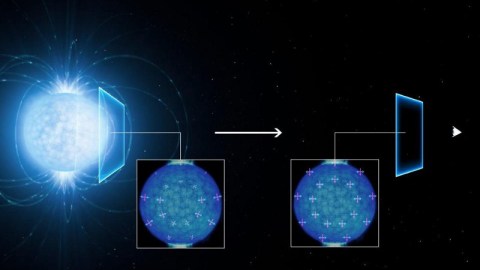
One of the most famous quantum physicists of all makes his mark in space, 80 years after first predicting it.
“What we observe is not nature itself, but nature exposed to our method of questioning.” –Werner Heisenberg
Discovering that our Universe was quantum in nature brought with it a lot of unintuitive consequences. The better you measured a particle’s position, the more fundamentally indeterminate its momentum was. The shorter an unstable particle lived, the less well-known its mass fundamentally was. Solid, material objects exhibit wave-like properties. And perhaps most puzzlingly of all, empty space — space that’s had all of its matter and radiation removed — isn’t empty, but is rather filled with virtual pairs of particles and antiparticles. 80 years ago, physicist Werner Heisenberg (who determined the two fundamental uncertainty relations), along with Hans Euler, predicted that because of these virtual particles, strong magnetic fields should affect how light propagates through a vacuum. Thanks to neutron star astronomy, that prediction has just been confirmed.
We might take the name “neutron star” quite literally, and assume that it’s made out of neutrons exclusively, but that’s not quite right. The outer 10% of a neutron star consists mostly of protons and even electrons, which can stably exist without being crushed at the surface. Because neutron stars rotate extremely rapidly — more than 10% the speed of light — those charged particles are always in motion, meaning they produce electric currents and magnetic fields. The magnetic fields themselves should affect the particle/antiparticle pairs present in empty space differently, since they have opposite charges. And if you have light passing through that region of space, it should get polarized dependent on the strength of the field.
This effect is known as vacuum birefringence, and occurs as the charged particles get yanked in opposite directions by the strong magnetic field lines. Because the effect scales as the square of the magnetic field strength, it makes sense to look at neutron stars for this effect. While Earth’s magnetic field is about 100 microTesla, the strongest magnetic fields we produce on Earth are only about 100 Tesla: strong, but not strong enough. But with the extreme conditions of neutron stars, large regions of space contain magnetic fields in excess of 10⁸ Tesla, making this an ideal place to look.
Although not very much light is emitted from the surface of the neutron star, the light that is emitted must pass through the strong magnetic field on its way to our telescopes, detectors and eyes. Because the space exhibits this vacuum birefringence effect, the light passing through it must get polarized, and it should all exhibit a common direction of polarization. By measuring the light from the very faint neutron star RX J1856.5–3754 with the Very Large Telescope in Chile, a team led by Roberto Mignani was able to measure the polarization degree for the first time. The actual data shows a large effect: a polarization degree of around 15%.
If you do the calculation for what the effect of vacuum birefringence ought to be and subtract it out, as the authors do, you can clearly see that it accounts for nearly all of the polarization. The data and the predictions match practically perfectly.
The reason this neutron star — as opposed to others — is so perfect for this measurement is that most neutron stars have their surface obscured by a dense, plasma-filled magnetosphere. If we tried to look at the pulsar in the Crab Nebula, for example, we’d have no chance of making this observation at all. The region around it is simply opaque to the types of light we’d like to measure.
Heisenberg and Euler made this prediction all the way back in 1936, and it’s gone completely untested until now. Thanks to this pulsar, we have confirmation that light polarized in the same direction as the magnetic field has its propagation affected by quantum physics, in exact agreement with the predictions from quantum electrodynamics. A theoretical prediction from 80 years ago adds another feather in the cap of Heisenberg, who can now posthumously add “astrophysicist” to his resume. But RX J1856.5–3754 can, in the future, confirm vacuum birefringence even more strongly by looking in the X-rays.
We don’t have a space telescope capable of measuring X-ray polarization today, but the ESA’s upcoming Athena mission will do exactly that. As opposed to the ~15% polarization the visible light exhibits, X-rays ought to be ~100% polarized. Athena is currently slated for launch in 2028, and combined with giant ground-based observatories like the Giant Magellan Telescope and the ELT, should deliver this confirmation for many such neutron stars. It’s another victory for the unintuitive but fascinating quantum Universe.
Reference: Evidence for vacuum birefringence from the first optical polarimetry measurement of the isolated neutron star RX J1856.5−3754, R. P. Mignani, V. Testa, D. Gonzalez Caniulef, R. Taverna, R. Turolla, S. Zane and K. Wu, MNRAS 465, 492 (2016).
This post first appeared at Forbes, and is brought to you ad-free by our Patreon supporters. Comment on our forum, & buy our first book: Beyond The Galaxy!