Has LIGO already discovered evidence for quantum gravity?
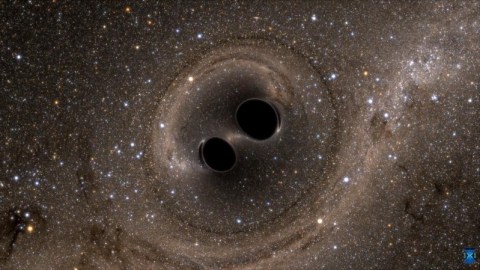
Merging black holes are some of the most extreme events in the Universe. Could a modified event horizon reveal quantum gravity?
“The bedrock nature of space and time and the unification of cosmos and quantum are surely among science’s great ‘open frontiers.’ These are parts of the intellectual map where we’re still groping for the truth — where, in the fashion of ancient cartographers, we must still inscribe ‘here be dragons.’”
–Martin Rees
When Einstein first wrote down the general theory of relativity in 1915, this brand new theory of gravity not only explained phenomena that Newton’s old one couldn’t, it predicted a whole host of new ones. In strong gravitational fields, clocks would run slower, light would shift its frequency, particle trajectories would bend, and accelerating masses would emit a new type of radiation: gravitational waves. While a great many of Einstein’s predictions had been borne out and verified over the years, it took until 2015 for the first gravitational wave signals to be directly detected by humanity. There were two that had enough significance to be announced as “discoveries,” while one other remains a strong candidate. But perhaps these events — created by merging black holes — will do us one better than Einstein: perhaps they’ve already given us our first hints of quantum gravity. In a new paper by theoretical physicists Jahed Abedi, Hannah Dykaar and Niayesh Afshordi, they claim the first evidence of gravitational effects beyond general relativityin the data of these mergers.
The reason it’s so difficult to go beyond general relativity is because the scale at which quantum effects should become important happen at extreme scales. Not extreme like at the LHC or in the center of the Sun, but at energies well beyond anything the Universe has seen since the Big Bang, or at distance scales some 10¹⁸ times smaller than a proton’s width. While quantum effects show up for the other forces at much more accessible scales and energies, part of why a theory of quantum gravity has been so elusive is that we have no experiments to guide us. The only hopes we have, realistically, are to look in two places:
- At the echoes of cosmic inflation, the ultra-high-energy state of spacetime prior to the Big Bang.
- At and around the event horizons of black holes during catastrophic events, where quantum effects will be strongest.
For the first one, there are teams looking for particular polarization signals of the Big Bang’s leftover glow. If that signal shows up in the data with a particular pattern on a variety of angular scales, it will be an unambiguous verification of inflation, plus the first direct evidence that gravity is quantum in nature. While many things in the Universe produce gravitational waves, some of these processes are classical (like inspiraling black holes), while others are purely quantum. The quantum ones rely on the fact that gravitation, like the other forces, should exhibit quantum fluctuations in space and time, along with the inherent uncertainty that quantum physics brings with it. In cosmic inflation, those fluctuations get stretched across the Universe, and can imprint in the Big Bang’s leftover glow. While the initial report of such a detection a few years ago, by BICEP2, was shown to be false, the prospects remain enticing.
But there’s another approach: to look for quantum effects that show up along with the classical ones in the strongest gravitational wave signals this Universe generates. LIGO’s announcements earlier this year gave the scientific community a celebratory jolt, as the first and second gravitational wave events from merging black holes were unambiguously detected. A third probably detection was also released, but was just below the significance threshold for discovery. While LIGO has just recently fired back up at increased sensitivity, a new idea gives us something important to look for: quantum corrections that show up in the mergers.
According to Einstein, a black hole’s event horizon should have specific properties, determined by its mass, charge and angular momentum. In most ideas of what quantum gravity would look like, that event horizon would be no different. Some models, however, predict notably different event horizons, and it’s those departure models that offer a glimmer of hope for quantum gravity. If we see a difference from what Einstein’s theory predicts, perhaps we can uncover not only that gravity must be a quantum theory, but what properties quantum gravity actually has.
The templates for LIGO generated by teams working with numerical relativity fit the merger events extremely well. After all, that’s how they were able to tease the signal out of such spectacular noise; they knew exactly what they were looking for and how to find it. If there’s a secondary, sub-dominant signal in there, arising from quantum gravity, a similar approach should be able to uncover it. The key — if these are quantum gravitational effects — is that they should occur at the Planck scale: at energies of 10¹⁹ GeV or distance scales of around 10^-33 meters. This is exactly the type of signal that Abedi, Dykaar and Afshordi decided to look for.
In classical (Einstein’s) general relativity, there are a few problems that arise from black holes: that there ought to be a firewall at the event horizon; that information about what falls into the black hole appears to be destroyed; how you reconcile a black hole-containing Universe with one that has a non-zero, positive cosmological constant. Some of the proposed quantum gravitational resolutions modify the event horizon of a black hole. When two black holes merge under these scenarios, the differences in the event horizons from Einstein’s theory should lead to “echoes” visible in the merging gravitational wave signal. They’ll be dominated by the main, Einsteinian prediction, but with good enough data and good enough algorithms, we should be able to tease that signal out, too.
In particular, there should be an echoing timescale, defined solely by the masses of the merging black holes and the frequencies at which they are merging or inspiraling. There should be these periodic echoes as the signals from the two event horizons interact, and it should exhibit “after-echoes” that continue for some time after the merger is complete.
Interestingly, when they compare it to the data from all three mergers, they arrive at a prediction for what they ought to see: it ought to exhibit these extra waves on timescales related to the echo period and the merger/inspiral period. The most unambiguous and easy-to-detect signal, from GW150914, contains the greatest information and significance: it shows evidence for this signal at almost exactly the predicted frequency, with only a 0.54% offset. (And they searched over a range with a ±5% offset.) If you then add in the signals for the other two black hole mergers using those same parameters, the statistical significance increases from 95% (about a 1-in-20 chance of random fluctuations) to 99.6% (about a 1-in-270 chance).
On the one hand, this is incredible. There are very few prospects for detecting a signal from quantum gravity because of the fact that we don’t have a working theory of quantum gravity; all we have are models and approximations. Yet some classes of models make some actual, testable predictions, albeit with uncertainties, and one of those predictions is that merging black holes, in some models, should emit additional echoes of particular frequencies and amplitudes.
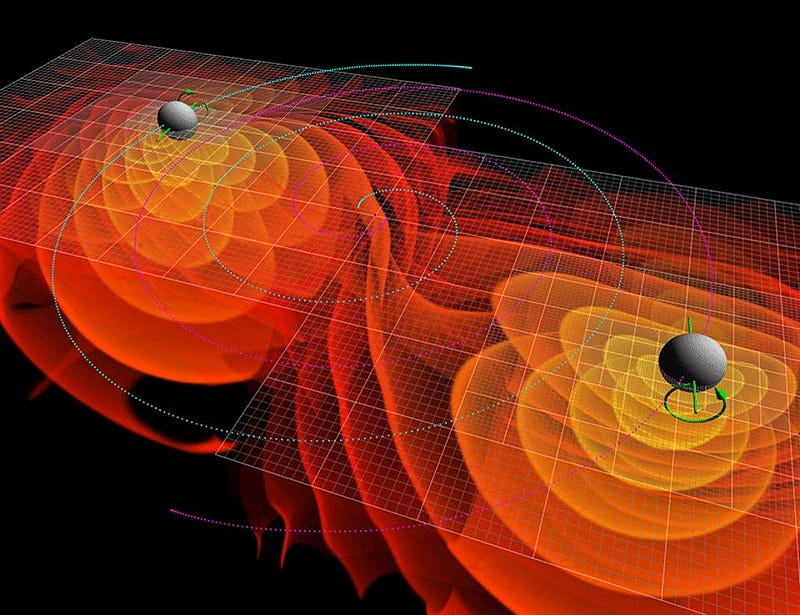
But on the other hand, there are reasons to doubt that this effect is real.
- Only the first gravitational wave signal, GW150914, exhibits enough significance to have this additional signal stand out against the background on its own. The other two are undetectable without assuming the prior results from GW150914.
- There is an additional signal offset by -2.8% from the predicted frequency at nearly 95% confidence when all three gravitational wave signals are included, and three more at greater than 80% confidence.
- And perhaps most damningly, we have known for months that there are additional signals, likely from external sources, superimposed on the LIGO data at a 3.2-sigma (99.9%) confidence level.
In other words, there may or may not be a real signal there, and it may have nothing to do with quantum gravity at all even if it is real.
But this new paper is remarkable for the fact that it makes an explicit prediction for what a quantum gravitational signature in the LIGO data will look like. It takes advantage of the actual LIGO data to show that there is the hint of a signal already there, and it explicitly tells the LIGO team what signatures they should look for in future events to see if this model of quantum gravity has it right. As LIGO is now operational once again at even greater sensitivity than during its prior run, we have every reason to expect that more black hole mergers are coming. The smart money is still on this signal not being real (or if it is, that it’s due to an external source rather than quantum gravity), but science never advanced without looking for an out-of-the-mainstream possibility. This time, the technology is already in place, and the next 24 months should be critical in revealing whether quantum gravity shows itself in the physics of merging black holes!
This post first appeared at Forbes, and is brought to you ad-free by our Patreon supporters. Comment on our forum, & buy our first book: Beyond The Galaxy!