Forget WIMPs, Axions And MACHOs: Could WIMPzillas Solve The Dark Matter Problem?
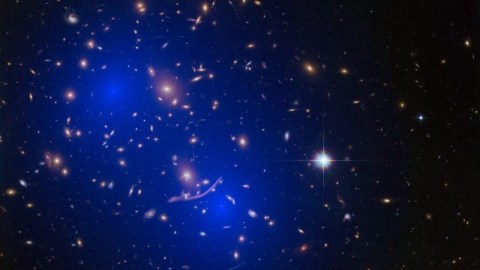
Our dark matter searches have yet to yield a robust detection. Could we be looking in all the wrong places?
There’s perhaps no more fundamental question to ask than, “what is the Universe made of?” What we see, directly, is dominated by normal matter: things made of particles we know well like protons, neutrons, and electrons, and the photons they emit. But our measurements of the largest structures in the Universe indicate that this is only 5% of what’s out there. The rest is dark matter and dark energy. While dark energy might be an inherent property of space itself, we assume, because of its gravitational effects, that dark matter clusters, clumps, and is made of particles.
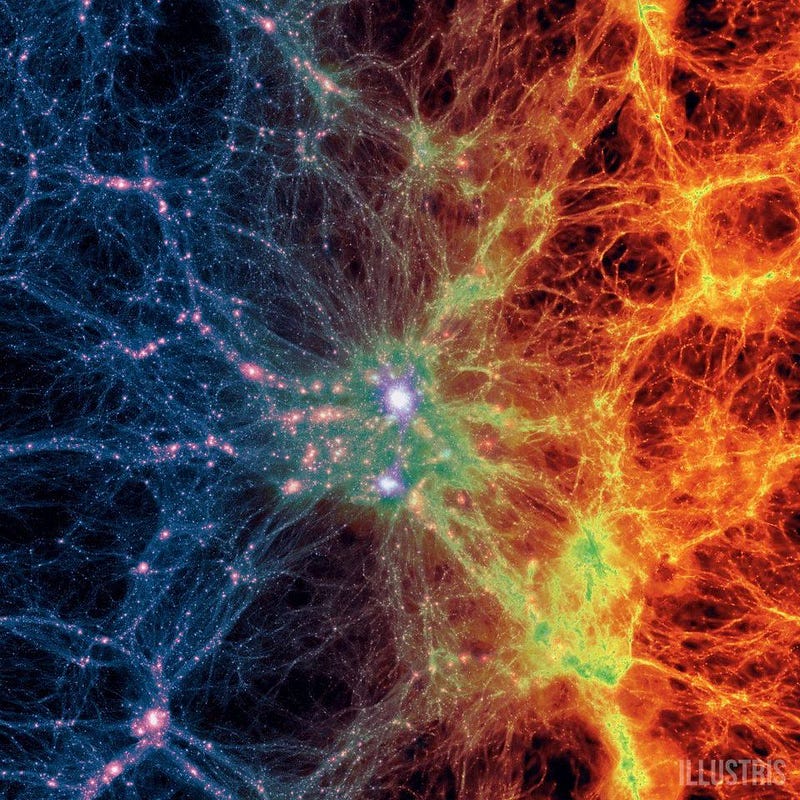
But what, exactly, is dark matter? And, moreover, can we be certain it exists? There are a huge suite of detectors and experiments out there searching for it, and yet no robust, verified, direct detection has ever been reported. There is no smoking gun we can point to and say, “this was an event caused by an interaction with dark matter.” The overwhelming majority of detectors out there are looking for WIMP-type dark matter, with a small contingent also looking for axions. (MACHOs, or other sources of “normal” dark matter, have been ruled out.) But all of this may be misguided. Dark matter might not be any of those things we’re looking for. In fact, it’s arguable that the candidate with the best motivations for it have no experiments to their name at all: WIMPzillas!
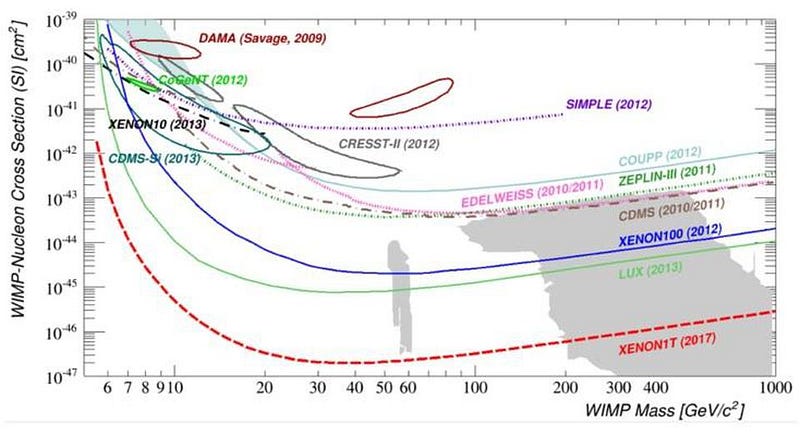
There’s an old story about a drunk looking for his keys under a lamppost outside of a bar. The drunk continues to look in the same place, over and over, despite not finding his keys there and it being quite obvious there are no keys there. A policeman approaches and asks the drunk what he’s doing, and the drunk says, “searching for my keys.” The policeman asks why he continues to search here, when it’s obvious they aren’t here. “Because that’s where the light is!” Clearly, there’s a lesson here: the evidence pointing to the absence of WIMP-type dark matter has no bearing on the evidence for all other types.
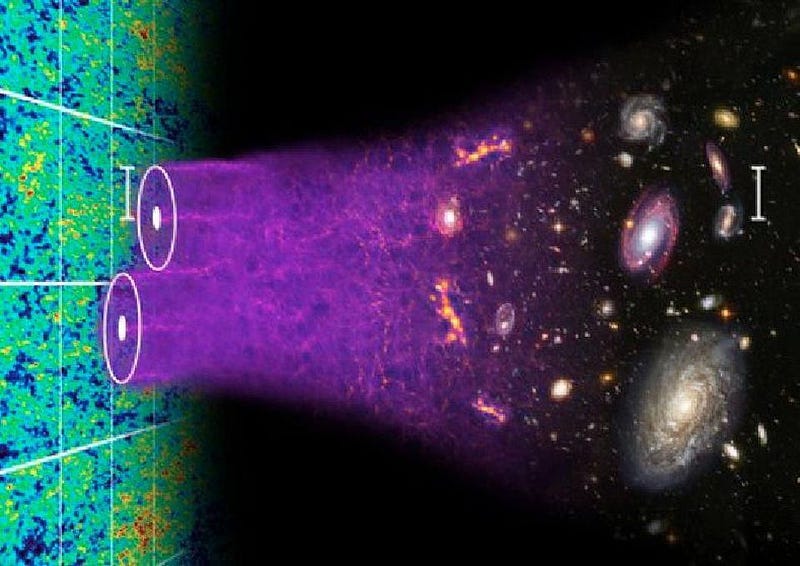
And yet, the full suite of evidence in astronomy, astrophysics, and cosmology all points to dark matter as being a necessity. In order to get the Universe we see and know today, including to give you:
- the observed fluctuations in the cosmic microwave background,
- the small-and-large-scale clustering features of galaxies,
- the rotation profiles of spiral and elliptical galaxies,
- the gravitational lensing effects of galaxy clusters, along with many more observations,
you need an extra type of matter in addition to what the Standard Model predicts: some type of dark matter. This dark matter must be about five times as abundant as all the normal (Standard Model) stuff combined, it has to be massive, it has to clump and cluster together, and it has to be moving slowly compared to the speed of light. All sorts of indirect evidence exists for dark matter, but we’ve never directly detected it. In order to find out what its nature actually is, we’ll have to do just that.
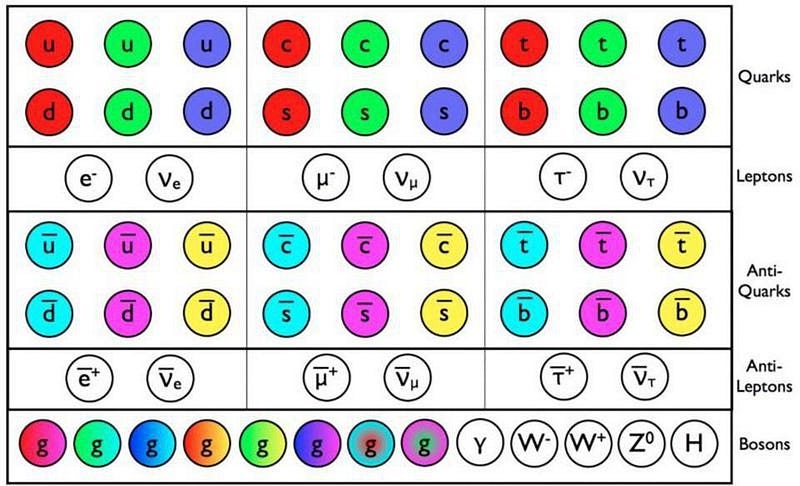
We understand the Standard Model of particle physics well enough to know how its particles behave, interact, and what their properties are. We can state with absolute certainty that perhaps no more than 1% (in the form of neutrinos) of the non-normal dark matter can be made out of all the things from the Standard Model. Whatever the overwhelming majority of dark matter is, it must be something not included in — or beyond — the Standard Model. This is a problem, because the Standard Model is so successful; it literally describes all the particle, their interactions, and properties that we’ve ever observed. The Universe needs physics beyond the Standard Model, but the particles we’ve observed give no indication that there is any beyond-the-Standard-Model physics we’ve yet discovered.
Except, that is, in one very important place.
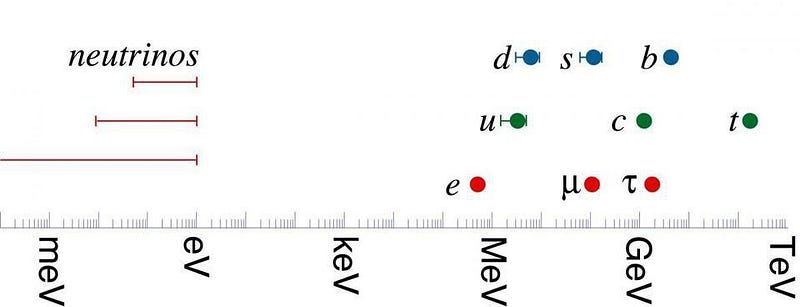
The biggest Standard Model mystery is the masses of neutrinos. All the other particles in the Standard Model either are completely massless (like the photon or gluon), or have a substantial mass that falls somewhere within a relatively large but well-defined range. The lightest particle, the electron, is about 511,000 electron-Volts in mass, while the heaviest, the top quark, is somewhere around 175,000,000,000 eV. This might seem like a big range, but a factor of less than 400,000 to cover all the particles is a pretty good deal.
For a long time, the neutrino was thought to be massless, too. But recent experiments have found that all three types — the electron, the mu and the tau — all have very small but non-zero masses, weighing in somewhere around the milli-electron-Volt range, or at least a factor of ten million times lighter than the electron!
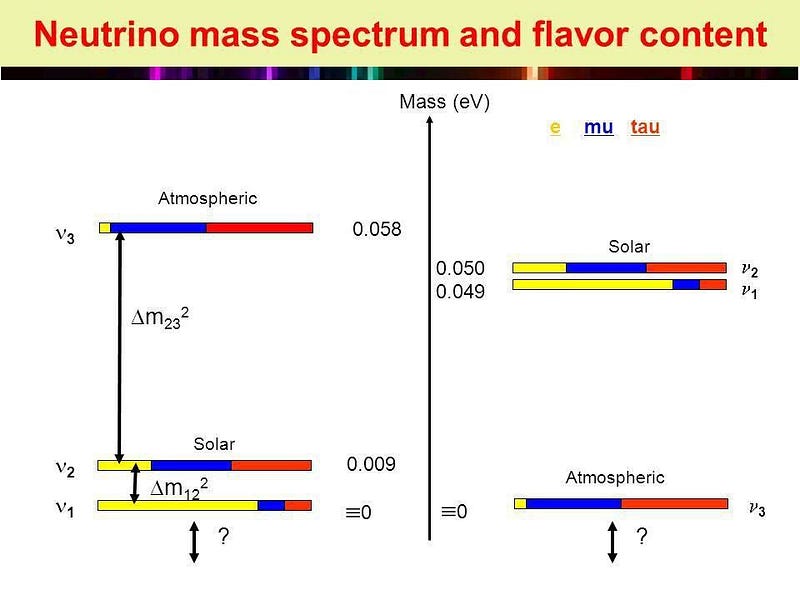
For particles that were predicted to be massless, this is a problem! Why would they not only have mass, but why would their masses be so remarkably tiny? One of the leading ideas — first put forth by a number of scientists in the late 1970s — is that neutrino masses could work like a see-saw! You see, the neutrinos that we see are all left-handed, meaning that if you orient yourselves in their direction of motion, they’re all seen to spin in the same fashion. Similarly, all the anti-neutrinos are right handed.
But if you assume that there’s a very large mass scale in nature, like a grand unification scale, then the neutrinos (both left and right-handed) could have had a “normal” mass like the other Standard Model particles, where they were sort-of balanced on a see-saw. But then that heavy mass from the unification scale comes along, sits on one side of the see-saw, and splits them: the left-handed neutrinos become very light, while the right-handed neutrinos become extremely heavy.
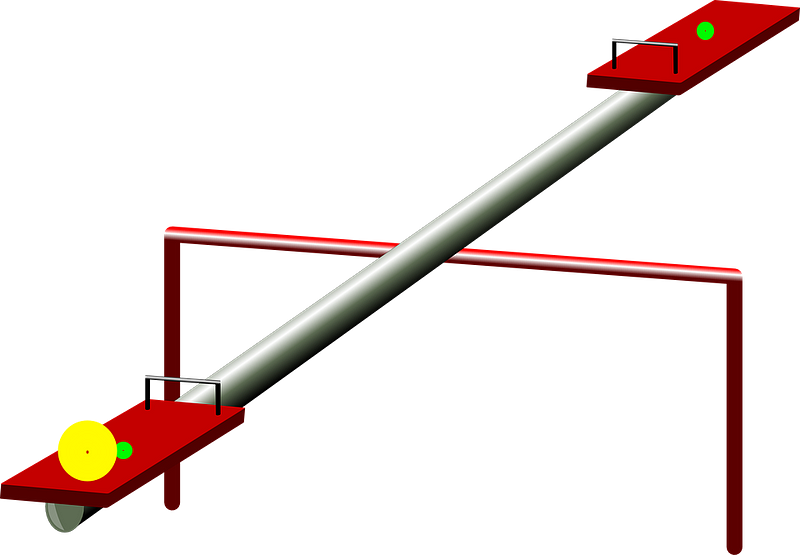
This is the leading explanation for how neutrinos oscillate, and also how they acquire such tiny (but non-zero) masses. But rather than hypothesizing supersymmetry, extra dimensions, axions or some other exotic solution to dark matter, here’s a fun possibility: the ultra-heavy, right-handed neutrinos could actually be the dark matter! Instead of being in the same range as the neutrino masses (like axions) or in the same range as the other Standard Model particles (like in SUSY or extra dimensions), they might be super-heavy: billions or even trillions of times heavier than the other Standard Model particles. In most models of particle physics, the hypothesized unification scale occurs around ~10¹⁵ GeV.
This new class of superheavy dark matter candidate, which could arise via this or any number of other mechanisms, such as purely gravitational interactions, has a fantastic name (coined by Rocky Kolb, Daniel Chung and Tony Riotto): WIMPzillas!
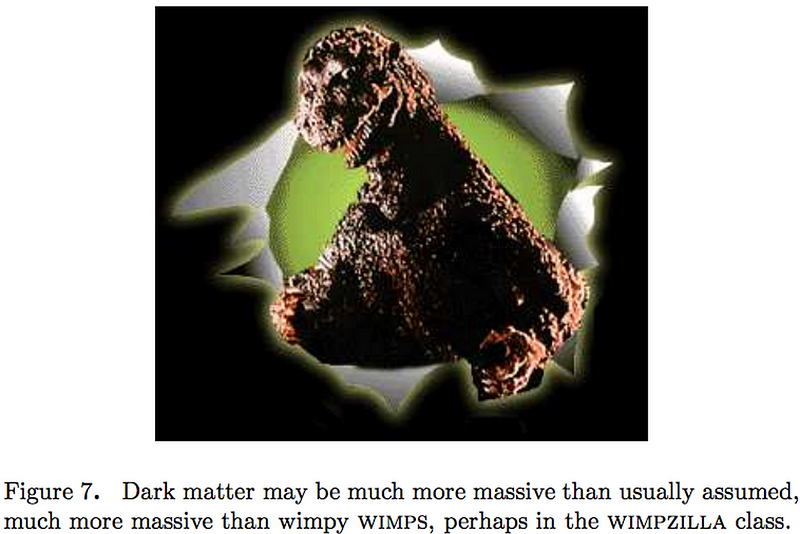
And yet, 20 years after they were proposed, there are zero experiments looking for WIMPzillas. The drunkards looking for their keys under the streetlamps still haven’t found them: dark matter has proved extremely elusive. The wimpy WIMPs they’ve been searching for, at around the ~GeV or ~TeV scale, have neither been created at the LHC nor have they shown themselves in direct detection experiment. While bigger and better searches will get you a more sensitive exclusion limit on these mass ranges, they won’t help you find any dark matter candidates outside of them.
And yet, it’s arguable that’s the mass range where we have the best motivation for dark matter to live: at these very high scales. The question, then, is what we’re going to do moving forward? Will we continue to build higher-intensity streetlights, hoping to at last illuminate these long-sought-after keys? Or will we try to illuminate the darkened landscape where we don’t even dare to look just yet? There aren’t particularly good, compelling ideas to search for such heavy dark matter, but that might be exactly the problem we need to crack to discover what dark matter actually is.
Ethan Siegel is the author of Beyond the Galaxy and Treknology. You can pre-order his third book, currently in development: the Encyclopaedia Cosmologica.