Five Surprising Truths About Black Holes From LIGO
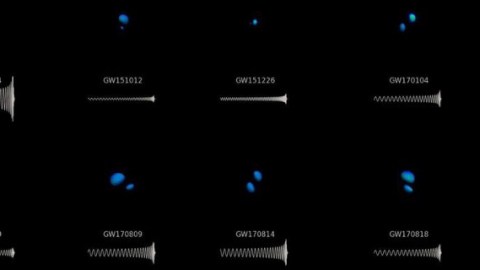
With a total of 10 black holes detected, what we’ve learned about the Universe is truly amazing.
On September 14th, 2015, just days after LIGO first turned on at its new-and-improved sensitivity, a gravitational wave passed through Earth. Like the billions of similar waves that had passed through Earth over the course of its history, this one was generated by an inspiral, merger, and collision of two massive, ultra-distant objects from far beyond our own galaxy. From over a billion light years away, two massive black holes had coalesced, and the signal — moving at the speed of light — finally reached Earth.
But this time, we were ready. The twin LIGO detectors saw their arms expand-and-contract by a subatomic amount, but that was enough for the laser light to shift and produce a telltale change in an interference pattern. For the first time, we had detected a gravitational wave. Three years later, we’ve detected 11 of them, with 10 coming from black holes. Here’s what we’ve learned.
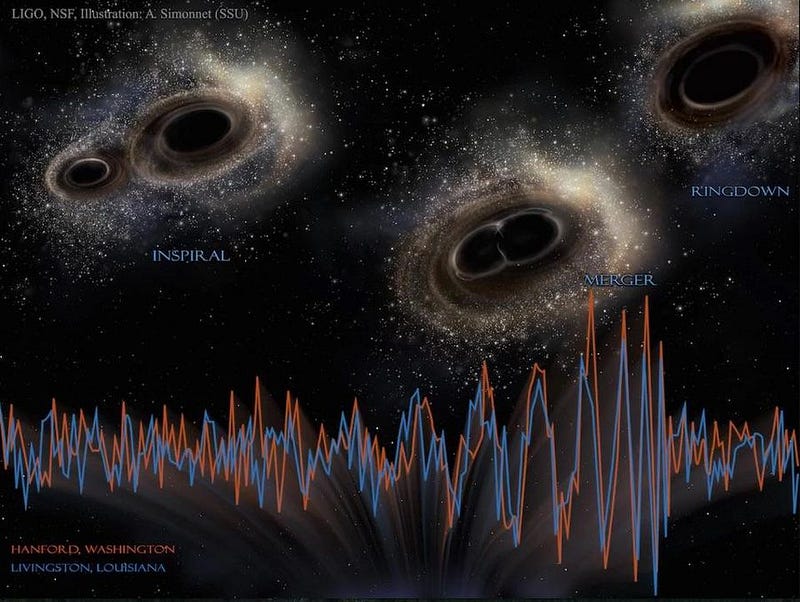
There have been two “runs” of LIGO data: a first one from September 12, 2015 to January 19, 2016 and then a second one, at somewhat improved sensitivity, from November 30, 2016 to August 25, 2017. That latter run was, partway through, joined by the VIRGO detector in Italy, which added not only a third detector, but significantly improved our ability to pinpoint the location of where these gravitational waves occurred. LIGO is currently shut down right now, as it’s undergoing upgrades that will make it even more sensitive, as it prepares to begin a new data-taking observing run in the spring of 2019.
On November 30th, the LIGO scientific collaboration released the results of their improved analysis, which is sensitive to the final stages of mergers between objects between about 1 and 100 solar masses.
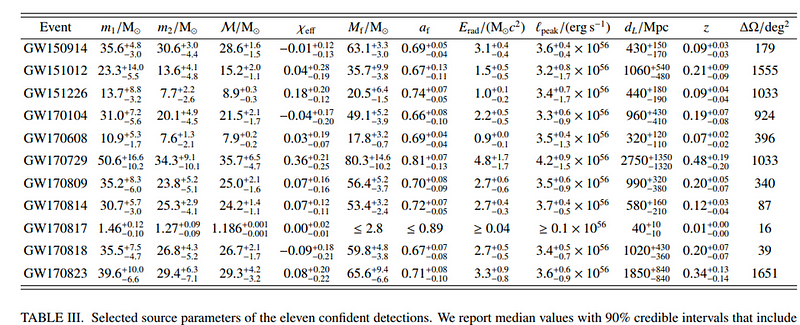
The 11 detections that have been made so far are shown above, with 10 of them representing black hole-black hole mergers, and only GW170817 representing a neutron star-neutron star merger. Those merging neutron stars was the closest event at a mere 130–140 million light years away. The most massive merger seen — GW170729 — comes to us from a location that, with the expansion of the Universe, is now 9 billion light years away.
These two detections are also the lightest and heaviest gravitational wave mergers ever detected, with GW170817 colliding a 1.46 and a 1.27 solar mass neutron star, and GW170729 colliding a 50.6 and a 34.3 solar mass black hole together.
Here are the five surprising truths that we’ve learned from all of these detections combined.
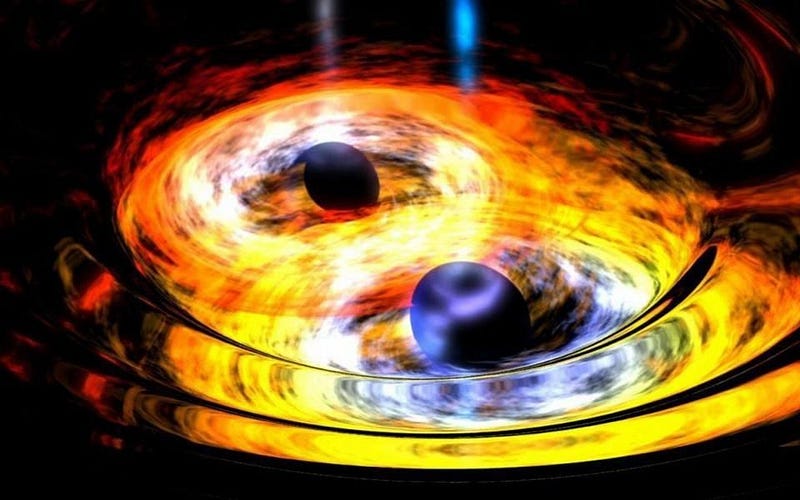
1.) The largest merging black holes are the easiest to see, and they don’t appear to get larger than about 50 solar masses. One of the best things about looking for gravitational waves is that it’s easier to see them from farther away than it is for a light source. Stars appear dimmer in proportion to their distance squared: a star 10 times the distance is just one-hundredth as bright. But gravitational waves are dimmer in direct proportion to distance: merging black holes 10 times as far away produce 10% the signal.
As a result, we can see very massive objects to very great distances, and yet we don’t see black holes merging with 75, 100, 150, or 200+ solar masses. 20-to-50 solar masses are common, but we haven’t seen anything above that yet. Perhaps the black holes arising from ultra-massive stars truly are rare.
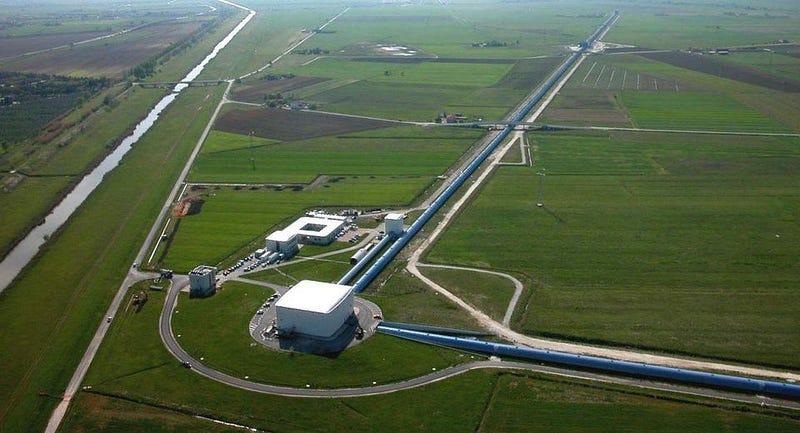
2.) Adding in a third detector both improves our ability to pinpoint their positions and increases the detection rate significantly. LIGO ran for about 4 months during its first run and 9 months during its second. Yet, fully half of their detections came in the final month: when VIRGO was running alongside it, too. In 2017, gravitational wave events were detected on:
- July 29th (50.6 and 34.3 solar mass black holes),
- August 9th (35.2 and 23.8 solar mass black holes),
- August 14th (30.7 and 25.3 solar mass black holes),
- August 17th (1.46 and 1.27 solar mass neutron stars),
- August 18th (35.5 and 26.8 solar mass black holes), and
- August 23rd (39.6 and 29.4 solar mass black holes).
During this final month of observing, we were detecting more than one event per week. It’s possible that, as we becomes sensitive to greater distances and smaller-amplitude, lower-mass signals, we may begin seeing as many as one event per day in 2019.
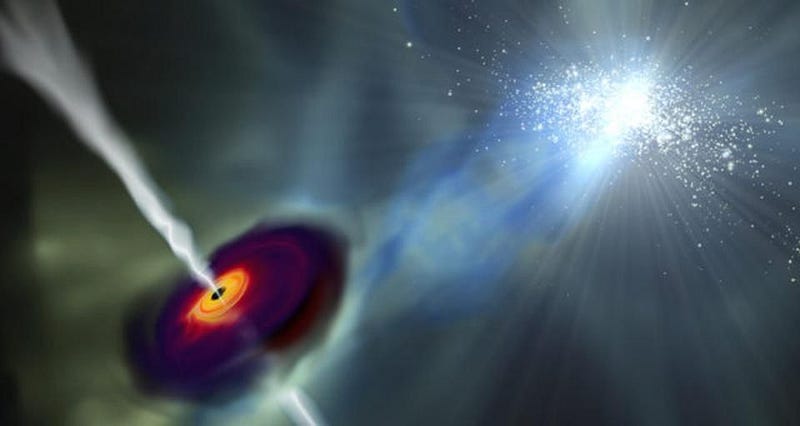
3.) When the black holes we’ve detected collide, they release more energy at their peak than all the stars in the Universe combined. Our Sun is the standard by which we came to understand all other stars. It shines so brightly that its total energy energy output — 4 × 10²⁶ W — is equivalent to converting four million tons of matter into pure energy with every second that goes by.
With an estimated ~10²³ stars in the observable Universe, the total power output of all the stars shining throughout the sky is greater than 10⁴⁹ W at any given time: a tremendous amount of energy spread out over all of space. But for a brief few milliseconds during the peak of a binary black hole merger, every one of the observed 10 events outshone, in terms of energy, all the stars in the Universe combined. (Although it’s by a relatively small amount.) Unsurprisingly, the most massive merger tops the charts.
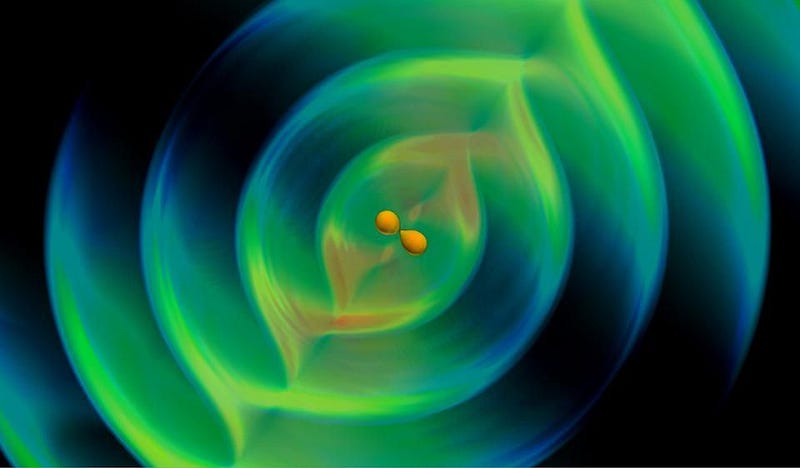
4.) About 5% of the total mass of both black holes gets converted into pure energy, via Einstein’s E = mc², during these mergers. The ripples in space that these black hole mergers produce need to get their energy from somewhere, and realistically, that has to come out of the mass of the merging black holes themselves. On average, based on the magnitude of the gravitational wave signals we’ve seen and the reconstructed distances to them, black holes lose about 5% of their total mass — having it converted into gravitational wave energy — when they merge.
- GW170608, the lowest mass black hole merger (of 10.9 and 7.6 solar masses), converted 0.9 solar masses into energy.
- GW150914, the first black hole merger (of 35.6 and 30.6 solar masses), converted 3.1 solar masses into energy.
- And GW170729, the most massive black hole merger (at 50.6 and 34.3 solar masses), converted 4.8 solar masses into energy.
These events, creating ripples in spacetime, are the most energetic events we know of since the Big Bang. They produce more energy than any neutron star merger, gamma-ray burst, or supernova ever created.
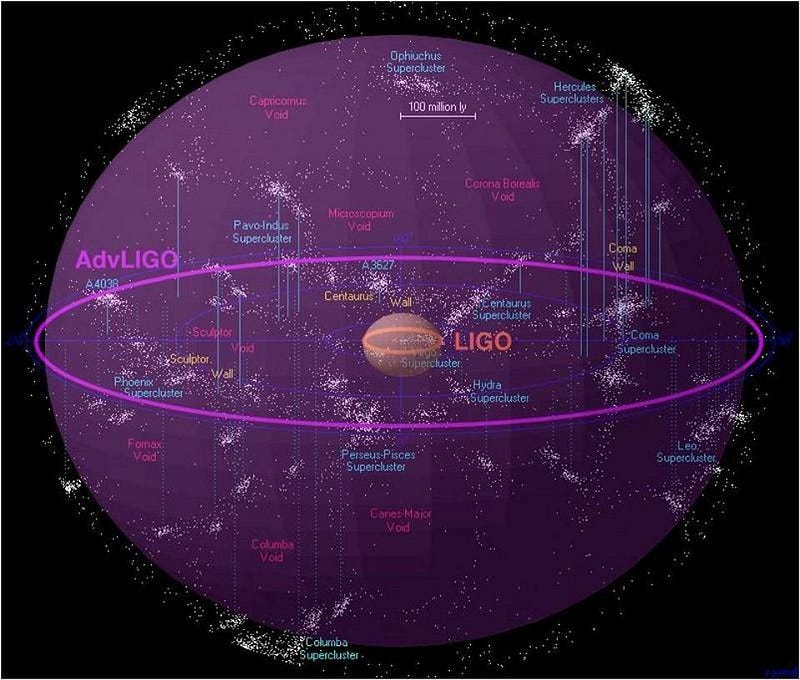
5.) With everything we’ve seen so far, we fully expect there are lower-mass, more frequent black hole mergers just waiting to be seen. The most massive black hole mergers produce the largest-amplitude signals, and so are the easiest to spot. But with the way volume and distance are related, going twice as distant means encompassing eight times the volume. As LIGO gets more sensitive, it’s easier to spot massive objects at greater distances than low-mass objects that are close by.
We know there are black holes of 7, 10, 15, and 20 solar masses out there, but it’s easier for LIGO to spot a more massive one farther away. We expect there are black hole binaries with mismatched masses: where one is much more massive than the other. As our sensitivities improve, we expect there are more of these out there to find, but the most massive ones are easier to find. We expect the most massive ones to dominate the early searches, just as “hot Jupiters” dominated early exoplanet searches. As we get better at finding them, expect there to be greater numbers of lower-mass black holes out there.
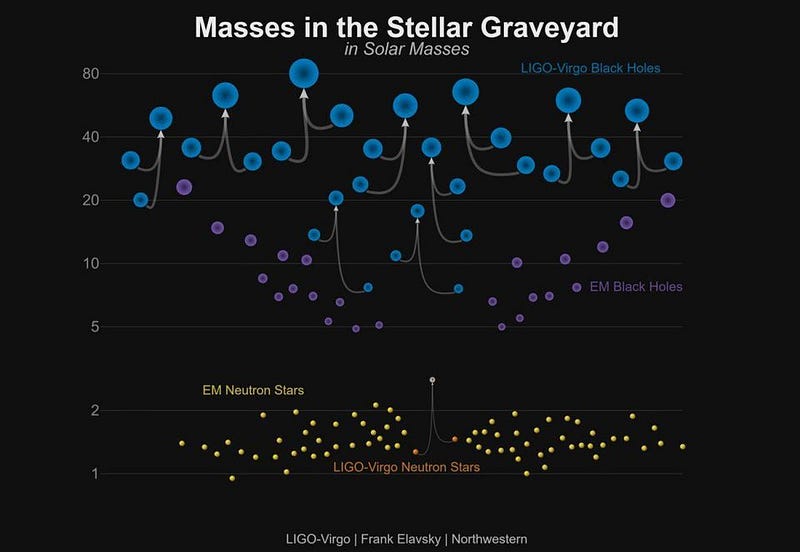
When the first gravitational wave detection was announced, it was heralded as the birth of gravitational wave astronomy. People likened it to when Galileo first pointed his telescope at the skies, but it was so much more than that. It was as though our view of the gravitational wave sky had always been shrouded in clouds, and for the first time, we had developed a device to see through them if we got a bright enough gravitational source: merging black holes or neutron stars. The future of gravitational wave astronomy promises to revolutionize our Universe by letting us see it in a whole new way. And that future has already arrived; we are seeing the first fruits of our labor.
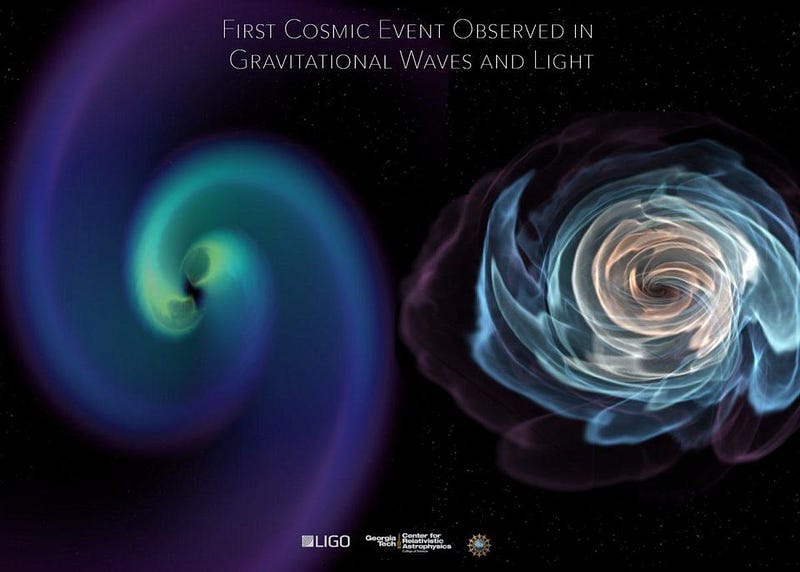
As our technology improves, we gain an ever-improved ability to see through those clouds: to see fainter, lower-mass, and more distant gravitational sources. When LIGO starts taking data again in 2019, we fully expect greater rates of ~30 solar mass black holes merging, but we hope to finally know what the lower-mass black holes are doing. We hope to see neutron star-black hole mergers. And we hope to go even farther out into the distant reaches of the Universe.
Now that we’ve made it into the double digits for the number of detected events, it’s time to go even farther. With LIGO and VIRGO fully operational, and at better sensitivities than ever, we’re ready to go one step deeper in our exploration of the gravitational wave Universe. These merging, massive stellar remnants were just the start. It’s time to visit the stellar graveyard, and find out what the skeletons are truly like.
Ethan Siegel is the author of Beyond the Galaxy and Treknology. You can pre-order his third book, currently in development: the Encyclopaedia Cosmologica.