Exoplanets: from fluke to fact

Astrophysicists have been looking for worlds like Proxima b since the 19th century. At last, they’re found!
This article is contributed by Sabine Hossenfelder. Sabine is a theoretical physicist specialized in quantum gravity and high energy physics. She also freelance writes about science.
“How vast those Orbs must be, and how inconsiderable this Earth, the Theatre upon which all our mighty Designs, all our Navigations, and all our Wars are transacted, is when compared to them. A very fit consideration, and matter of Reflection, for those Kings and Princes who sacrifice the Lives of so many People, only to flatter their Ambition in being Masters of some pitiful corner of this small Spot.” –Christiaan Huygens
Today, extrasolar planets, or exoplanets for short, are all over the news. Thousands are known and cataloged in the openly accessible Extrasolar Planets Encyclopaedia and NASA’s exoplanet archive. Seemingly every week, another remarkable specimen has been found. And some of these extrasolar planets even orbit stars in what is believed to be a habitable zone, fertile ground for the evolution of life. Last week’s spectacular announcement of the discovery of Proxima b, a potentially habitable, rocky planet around our nearest neighboring star, has shown us that perhaps Earth-like worlds may be more ubiquitous around stars than we’ve ever dreamed.
We owe most of these amazing finds to NASA’s Kepler satellite (and the follow-up mission K2) which has looked for several years at a small patch of the Milky Way that hosts an estimated 145,000 stars similar to our own sun. The data that Kepler has gathered, and still gathers, is analyzed for transits of planets that temporarily block part of the star’s surface and diminish its emission. The Kepler mission so far found more than 3,500 confirmed exoplanets with over 1,000 additional candidates. The unconfirmed ones are now subject to closer investigation.
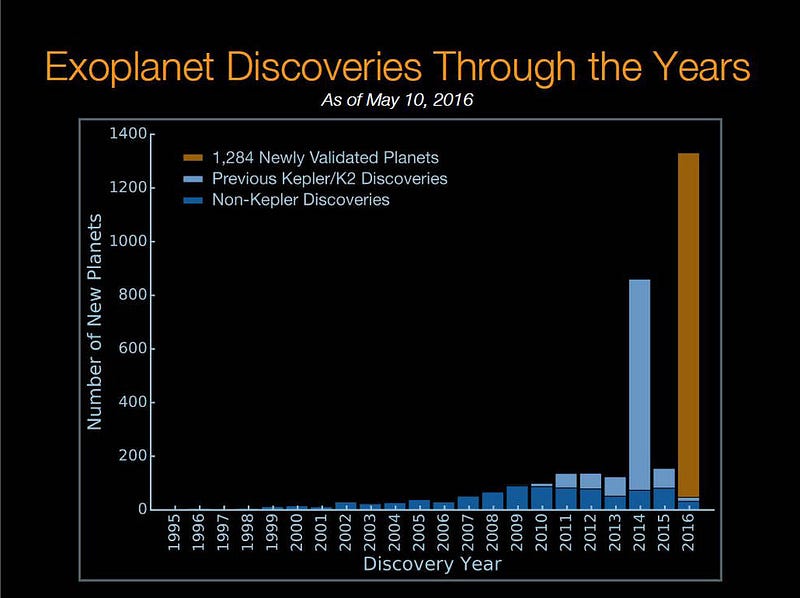
The progress in the field in the last decades can’t be called anything but stellar, but the scientific road to the discovery of the first exoplanet has been bumpy. Once you know that stars in the night sky are suns like our own, it doesn’t take a big leap of imagination to think that they might be accompanied by planets. Indeed, astrophysicists looked for exoplanets already in the 19th century, though without success. Beginning in the 1950s, several candidates for exoplanets made it into the popular press, but they turned out to be data flukes.
At that time, the experiments relied on detecting minuscule changes in the motion of the star caused by planets. If you recall the two-body problem from introductory physics, it’s not that one body orbits the other, but they both orbit around their common center-of-mass. But if one body is much heavier than the other, it might look almost like the lighter one is orbiting the heavier one, while the heavier one appears motionless. But if a sufficiently heavy planet orbits a star, astronomers can find out by closely monitoring the star because it should wobble around the center-of-mass. In the 50s, closely monitoring a star meant observing its distance as compared to other stellar objects. But the precision by which this could be done simply wasn’t sufficient to reliably tell the presence of a planet.
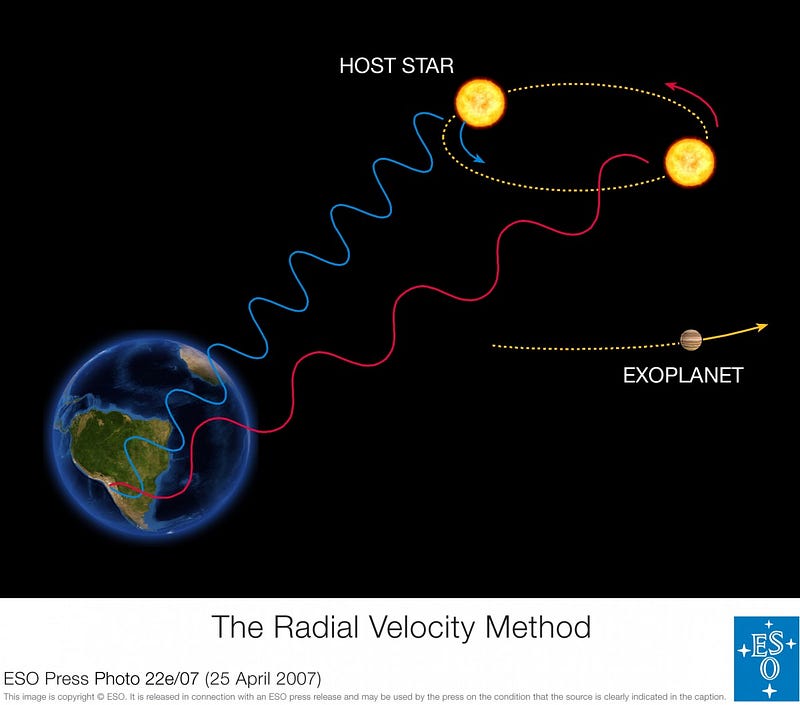
In the early 80s, however, Gordon Walker and his postdoc Bruce Campbell from British Columbia, Canada, pioneered a new technique for tracking the motion of stars. It relied on measuring the star’s absorption lines, whose frequency depends on the motion of the star relative to us because of the Doppler effect. This method allows to resolve much finer details and increased the precision by which the motion of stars could be tracked by two orders of magnitude.
To put that method to work, Walker and Campbell had to find a way to compare spectral images taken at different times so they’d know how much the spectrum had shifted. They found an ingenious way to do that: they would use the (very regular and well-known) molecular absorption lines of hydrogen fluoride gas. The comb-like absorption lines of hydrogen fluoride served as a ruler, relative to which they could measure the star’s spectrum, allowing them to detect even smallest changes.
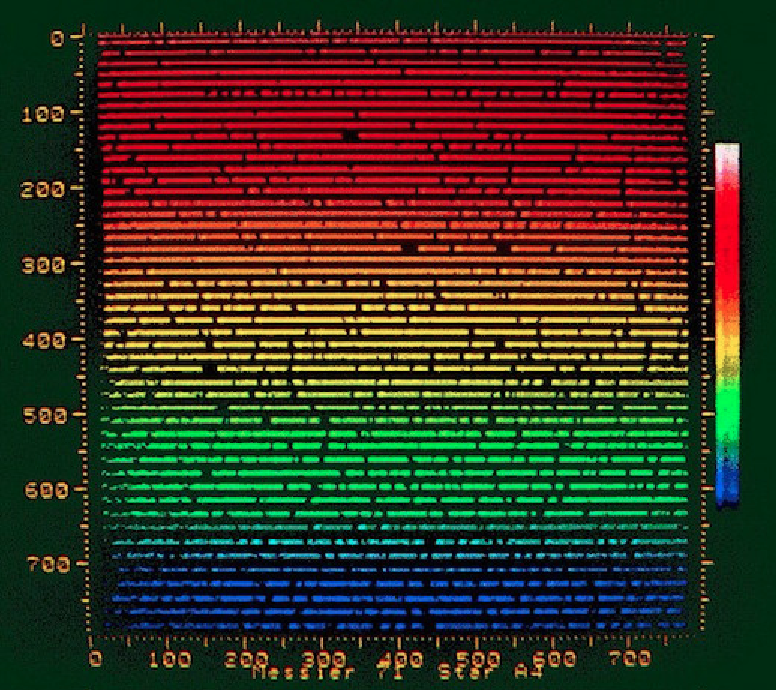
Once that problem was solved, Walker and Campbell, together with astronomer Stephenson Yang, started looking at candidate stars which might be accompanied by Jupiter-like planets. The scientists realized that to detect the motion of the star due to the planet, they would have to record the system for several orbits. Since our planet Jupiter needs about 12 years to orbit the sun, that meant they were likely in for a long-term project. And unfortunately they had a hard time finding support for it.
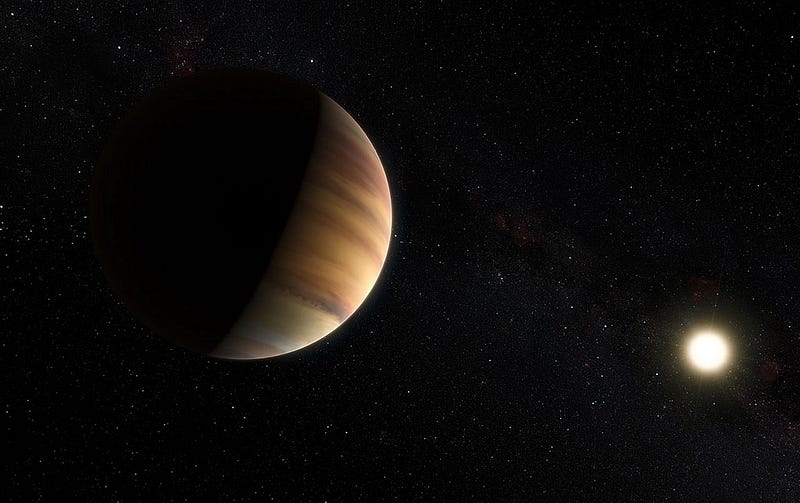
In his recollection “The First High-Precision Radial Velocity Search for Extra-Solar Planets” (arXiv:0812.3169), Gordon Walker recounts that it was difficult to get time for their project at observatories: “Since extra-solar planets were expected to resemble Jupiter in both mass and orbit, we were awarded only three or four two-night observing runs each year.” And though it is difficult to understand today, back then many of Walker’s astronomer colleagues thought the search for exoplanets was a waste of time. Walker writes:
It is quite hard nowadays to realise the atmosphere of skepticism and indifference in the 1980s to proposed searches for extra-solar planets. Some people felt that such an undertaking was not even a legitimate part of astronomy. It was against such a background that we began our precise radial velocity survey of certain bright solar-type stars in 1980 at the Canada France Hawaii 3.6-m Telescope.
After years of data taking, they had identified several promising candidates, but were too cautious to claim a discovery and decided to stick with “promising candidates.” At the 1987 meeting of the American Astronomical Society in Vancouver, Campbell announced their preliminary results. The press happily jumped to conclusions and reported yet another exoplanet discovery. But the other astronomers were skeptical even about Walker and Campbell’s cautious interpretation of the data.
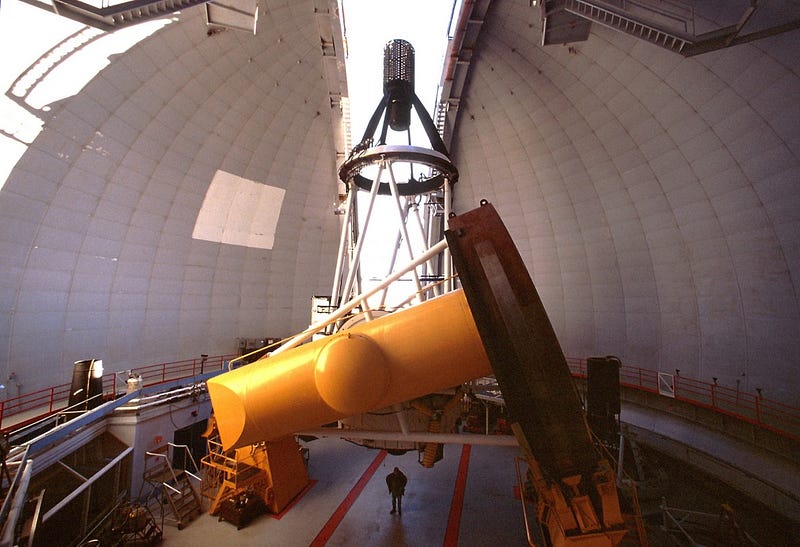
In his article “Lost world: How Canada missed its moment of glory,” Jacob Berkowitz describes the subdued reaction of the scientific community:
[Campbell]‘s professional colleagues weren’t as impressed [as the press]. One astronomer told The New York Times he wouldn’t call anything a planet until he could walk on it. No one even attempted to confirm the results.
Walker’s gifted postdoc Bruce Campbell suffered most from the slow-going project that lacked appreciation and had difficulties getting continued funding. In 1991, after more than a decade of data taking, they still had no discovery to show up with. Campbell meanwhile had reached the age of 42, and was still sitting on a position which was not only untenured, it wasn’t even tenure-track. Campbell’s frustration built up to the point where he quit his job. And not only that — when he left, he erased all the analyzed data in his university account. Luckily, his (both tenured) collaborators Walker and Yang could recover the data. Campbell made a radical career change and became a personal tax consultant.
But in late 1991, Walker and Yang were finally almost certain to have collected sufficient evidence of an exoplanet around the star gamma Cephei, whose spectrum showed a consistent 2.5 year wobble. Then, in a fateful coincidence, when Walker just thought they had pinned it down, one of his colleagues, Jaymie Matthews, came by his office, looked at the data and pointed out that the wobble in the data coincided with what appeared to be periods of heightened activity on the star’s surface. Walker looked at the data with new eyes and, mistakenly, believed that they had been watching all the time an oscillating star rather than a periodic motion of the star’s position.
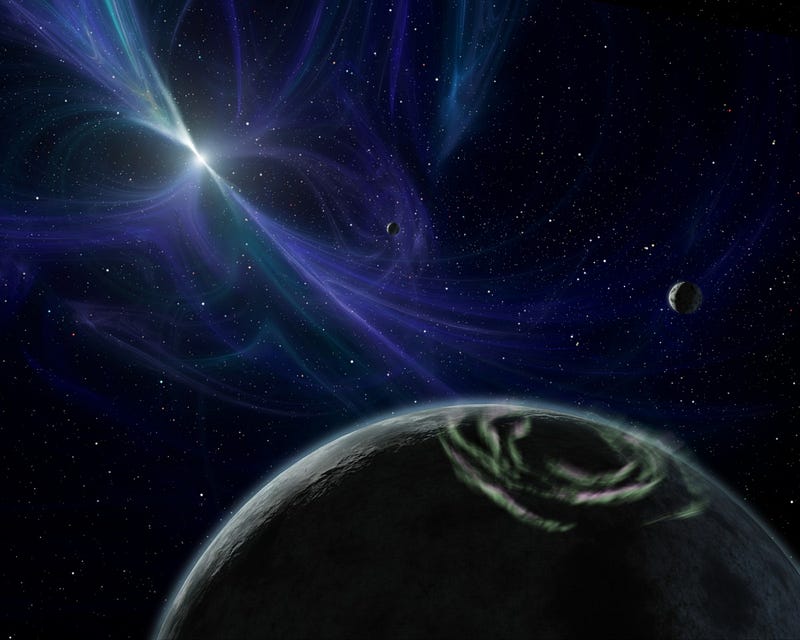
They weren’t the only one closing in on a discovery and that moment of doubt was enough to allow another team to win the race. In early 1992,Nature reported the first confirmed discovery of an exoplanet by Wolszczan and Frail, based in the USA. Yet, the planet they found orbits a millisecond pulsar (probably a neutron star), so for many astrophysicists this discovery doesn’t really count because the star’s collapse would have wiped out all life in that planetary system long ago.
In 1995 then, astronomers Mayor and Queloz of the University of Geneva announced the first definitive observational evidence for an exoplanet orbiting a normal star. The planet has an orbital period of a few days only; no decade-long recording was necessary. It wasn’t until 2003 that the planet that Walker, Campbell and Yang had been after was finally confirmed.
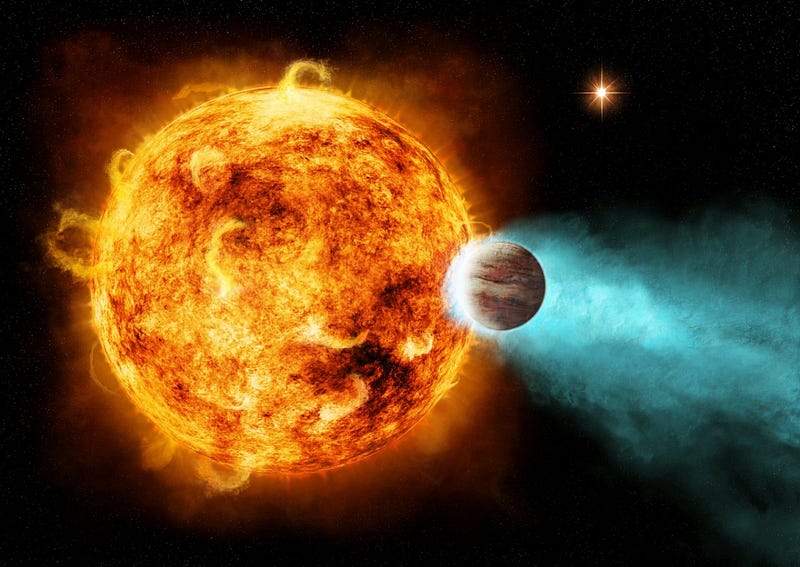
The Kepler mission launched in 2009. To get an impression for the remarkable amount of detail which can now be measured, look at the image below. It shows a time-series of measurements of the flux from some star observed with Kepler for several orbits. You can clearly recognize the dips that occur when the planet covers part of the surface — even though that decrease isn’t more than a tenth of a percent of the star’s total brightness.
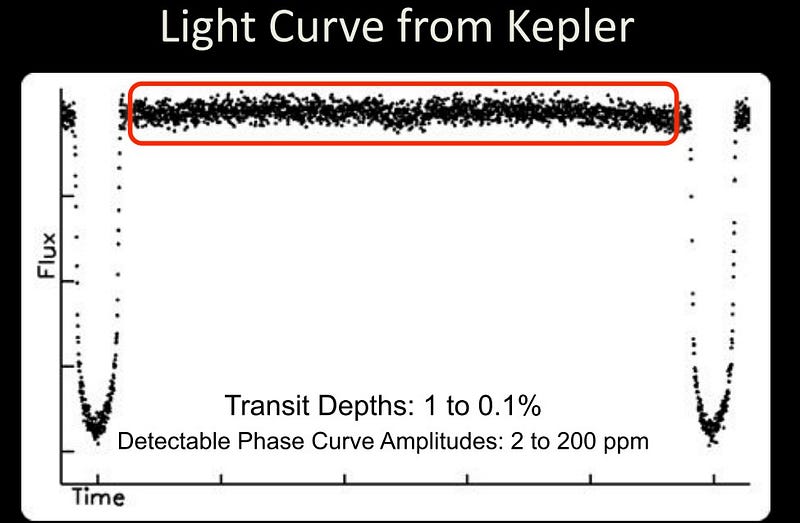
A decade ago that observation would have been an amazing feat all on its own. But now, take a look at the (red marked) data taken between the transits. If the planet doesn’t cover part of the star’s surface, it will reflect light from the star, and that too is observable. This reflection should be largest when the planet is just about to vanish behind the star, and then dip. That means there should be a fine-structure in the flux between the transits, at about two orders of magnitude smaller still than the already small transit signal. And in fact, the data and data analysis is already so good that even the vanishing of the planet behind the star can be measured!
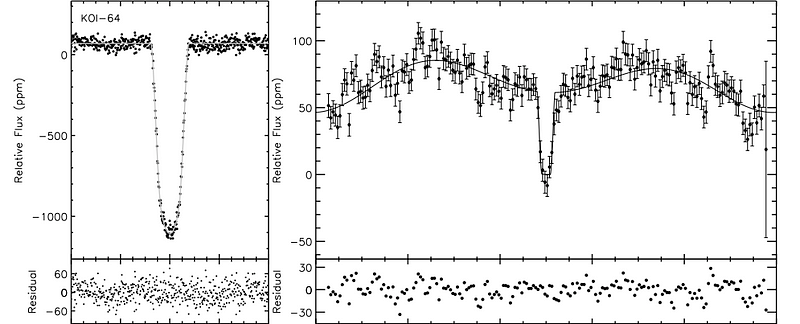
In the last decades, exoplanets have become one of the most rapidly developing research areas in physics. One of the biggest lessons we have learned is that planetary systems like ours are much more common outcomes of stellar formation than had previously been expected. The properties of distant solar systems can now be measured to a precision high enough to let physicists infer properties of the planet’s atmosphere and to index any new planet for potential habitability. Yet even with all we’ve discovered to date, we’re only just beginning to understand what else is out there.
This post first appeared at Forbes, and is brought to you ad-free by our Patreon supporters. Comment on our forum, & buy our first book: Beyond The Galaxy!